Fig. 10.1
Schematic representation of olfactory neuroepithelium. The olfactory neuroepithelium is composed by olfactory receptor neurons, supporting cells, and basal cells. The dendritic extension of olfactory receptor neurons carries on its surface several cilia, where the olfactory receptors are located. The axons run through the cribriform plate of the ethmoid bone and reach the olfactory bulb where they synapse with mitral cells in spherical structures named glomerulus
The ORNs are bipolar cells, with their dendritic extensions directed toward the olfactory cleft and carrying on its surface several cilia that project into the mucus. Odorants are carried through the mucus layer by olfactory binding proteins and bind to olfactory receptors located on the ORNs. In 1991, Axel and Buck (Buck and Axel 1991) discovered a family of approximately 1,000 genes that encode for an equivalent number of olfactory receptors, corresponding to the largest family of genes in the mammalian genome (Zhang and Firestein 2002), highlighting their important role in physiology. In the majority of mammals, most of these genes are functional, but in primate the number of functional genes decreases and is to about 350 in humans (Crasto et al. 2002). Axel and Buck found that each ORN possesses only one type of odorant receptor and each receptor is specialized for a small number of odors. Hence, a given odorant will bind a typical pattern of olfactory receptors. The binding results in the activation of G proteins. The activation of G proteins stimulates the formation of cyclic AMP. Increased levels of cAMP open cyclic nucleotide-gated channels. This causes the opening of the channels and Ca++ influx. This influx activates chloride channels, opening them up and, causing Cl- to leave, finally depolarizing the ORN and generating the action potential.
ORN axons converge into the olfactory nerves, passing through the cribriform plate of the ethmoid bone and projecting directly to the ipsilateral olfactory bulb where they synapse into spherical structures known as the glomerulus.
10.2.2.2 The First Olfactory Structure: The Olfactory Bulb
The olfactory bulb is ovoid in shape and located in the anterior cranial fossa, above the cribriform plate of the ethmoid bone, under the frontal lobe. It contains a major structure that can be considered to be the first olfactory structure: the glomerulus. The glomerulus is the only relay between the periphery and the cortex. Each glomerulus collects ORN axons from the same type of odorant receptor (Fig. 10.2). ORN axons and dendrites of mitral cells synapse in the glomerulus.
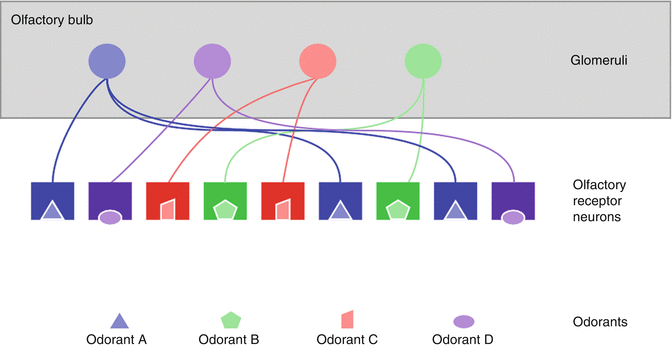
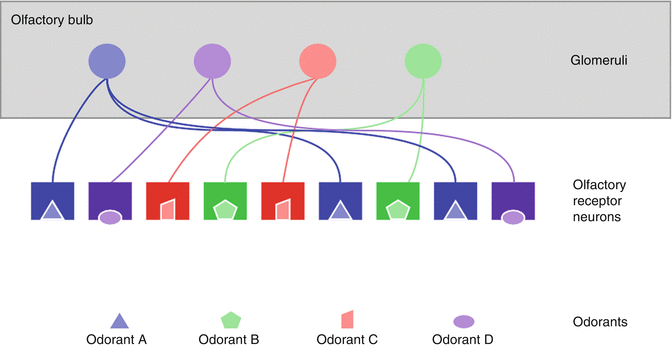
Fig. 10.2
Basic schematic representation of odor coding at the level of neuroepithelium and glomeruli. Odorant molecules bind with specific olfactory receptor neurons. Each olfactory receptor neuron possesses only one type of odorant receptor. Olfactory receptor neurons carrying the same type of receptor send their axon to the same glomerulus at the level of the olfactory bulb
The olfactory bulb has a multilayered cellular architecture. It encompasses 6 different layers: (1) the external layer is composed of ORN axons, (2) the glomerular layer is composed by glomeruli wherein axons of ORN synapse with dendrites of mitral cells, (3) the external plexiform layer consists of dendrites of mitral and tufted cells, (4) the mitral and tufted cell layer contains cell bodies of mitral and tufted cells (second-order olfactory neuron), (5) the internal plexiform layer, and (6) the granule cell layer contains rows of mitral and tufted axons and granule cells which are interneurons.
Axons of the mitral cells and tufted cells coalesce to form the olfactory tract, located at the base of the forebrain.
Centripetal information is secondary to neuronal activation, with glutamate as the principal neurotransmitter.
10.2.2.3 The Second Olfactory Structures: The Primary Olfactory Cortex
As compared to all other senses, olfaction is particular in that second-order olfactory neurons send information directly to primary olfactory cortex. In humans, the olfactory bulb is connected to the primary olfactory cortex by the fibers of the lateral olfactory tract (LOT). The LOT conveys olfactory information to a wide number of brain areas within the frontal lobe and the dorsomedial surface of the temporal lobe, often referred to as the primary olfactory cortex.
The primary olfactory cortex comprises the piriform cortex, which covers the uncus, the entorhinal cortex, the anterior olfactory nucleus, the periamygdaloid cortex, the olfactory tubercle, and nucleus. These projections are mainly ipsilateral but there are also contralateral connections via the anterior commissure (Cleland and Linster 1995; Royet and Plailly 2004; Lascano et al. 2010). Some of the structures of the primary olfactory cortex then project to the tertiary highest cognitive centers of the brain. The major projection of the piriform cortex is the thalamus, but it will also project to the insular cortex, the orbitofrontal cortex (neocortex), and the hypothalamus. The entorhinal cortex supplies afferent input to the hippocampus, while the olfactory tubercle connects to the thalamus. The amygdala is the major source of afferents to the hypothalamus (Fig. 10.3). Interestingly, there are many interactions between the secondary olfactory structures: between the anterior olfactory nucleus and the piriform cortex, the piriform cortex and the olfactory tubercle, and the piriform cortex and the entorhinal cortex.
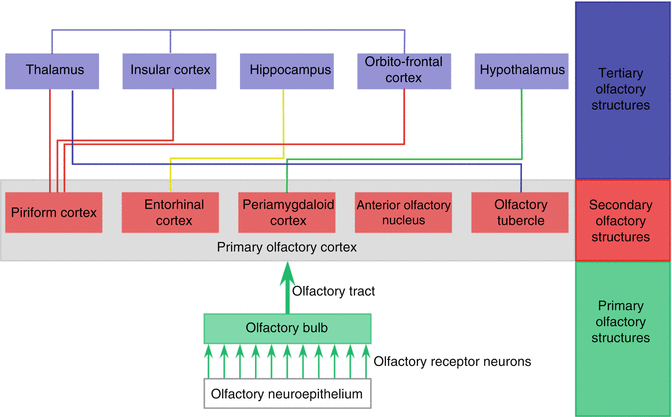
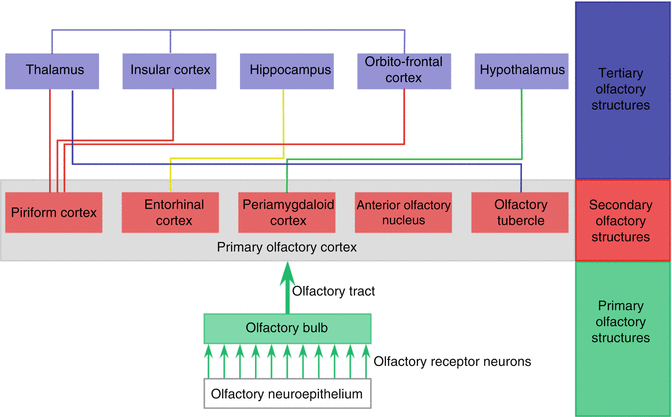
Fig. 10.3
Schematic diagram of major olfactory pathways
10.2.2.4 The Tertiary Olfactory Structures
The tertiary olfactory structures are the thalamus, the hypothalamus, the amygdala, the hippocampus, the orbitofrontal cortex, and the insular cortex.
The thalamus receives information from the piriform cortex and the olfactory tubercle. The hypothalamus, the orbitofrontal cortex, and the insular cortex also receive afferent input from the piriform cortex, while hippocampus is connected to entorhinal cortex. We should also note that there are also some interactions between these tertiary olfactory structures. In this way, the thalamus connects to the orbitofrontal cortex and the insular cortex. Therefore, the orbitofrontal cortex and the insular cortex receive direct input from the piriform cortex and indirect input via the thalamus.
10.2.2.5 Centrifugal Information
Most secondary and tertiary structures have numerous centrifugal fibers leading to the olfactory bulb, with GABA and acetylcholine as principal neurotransmitter. The supposed aim of this centrifugal information is to allow the brain to control the incoming flow of olfactory signals.
10.2.2.6 Properties of Olfactory Pathways
The olfactory pathways are distributed to different brain structures that are involved in the determination of our personal and social behavior. For example, the connections with the:
1.
Hippocampus and limbic system are thought to influence our memory system.
2.
Amygdala system could act on emotional, motivational, and craving circuits.
3.
Hypothalamus, that mediates feeding regulation, could influence our feeding behavior.
4.
Orbitofrontal cortex mediates our conscious perception of odors and could influence our preferences (Shepherd 2006).
Hence, odor perception may affect our behavior and plays a major role in our interaction with the environment.
The olfactory system presents unique properties as compared to other sensory systems. They are (1) the predominance of ipsilaterality of the olfactory projections, (2) the conduction of odor-evoked signals without an obligatory thalamic relay, and (3) the intimate overlap with limbic regions of the brain (Gottfried 2006):
1.
Odor processing remains principally ipsilateral (Cleland and Linster 1995; Royet and Plailly 2004; Lascano et al. 2010) all the way from the nasal periphery to the primary olfactory cortex. This feature is different for other sensory modalities such as the visual or auditory systems which, early in the processing pathways, supply sensory information in both hemispheres. This may help the cortex to better discriminate and to make bilateral odor comparisons and perhaps to provide differential access to odor memories.
2.
The absence of an obligatory thalamic relay is also in contrast with other sensory modalities in which an incoming signal undergoes thalamic modulation prior to being delivered to the sensory-specific cortex (Gottfried 2006). The absence of thalamic sensory integration in the olfactory pathways would seem to have an evolutionary explanation (Gottfried 2006).
3.
The connections between the olfactory system and the limbic system appear to be involved in the emotional and memory background to odorant stimuli, our social behavior, and the formation of novel stimulus-reinforced associations (Gottfried 2006).
10.2.3 Orthonasal and Retronasal Olfaction
Paul Rozin noted that smell is unique in having a “dual nature” – meaning that it can sense signals originating outside (orthonasal) or inside (retronasal) the body (Rozin 1982).
Orthonasal olfaction refers to odorants originating outside and sniffed in through the nares to reach the olfactory neuroepithelium. This route is used to smell odors from the environment, such as perfumes, food aromas, smoke, predator smell, social odors, or pheromones. Orthonasal olfaction is processed by olfactory pathways and is influenced by the visual pathway.
Retronasal olfaction refers to odorants originating from the back of the mouth and reaching the olfactory neuroepithelium via the nasopharynx. This retronasal stimulation occurs during food ingestion. It is activated only when breathing out through the nose, between mastication, or swallowing (Taylor et al. 2000; Shepherd 2006). The retronasal olfaction, also termed as “flavor,” account for an important part of food identification. This explains why a majority of patients suffering from smell disorder also complain of “taste” disorder, although their sense of taste is intact. On contrast to orthonasal olfaction, flavor perception is not only processed by olfactory pathways but is also influenced by almost all sensory modalities, which are taste, touch, sound, and proprioception (for a review, see Shepherd 2006). Indeed, the orbitofrontal cortex receives connections from other sensory neocortical areas (taste, hearing, touch, and vision) (Ongur et al. 2003) (Fig. 10.4). Since it is receiving multisensory input and integrating these different sensory informations, the orbitofrontal cortex is an important area to influence our food preferences and choices.
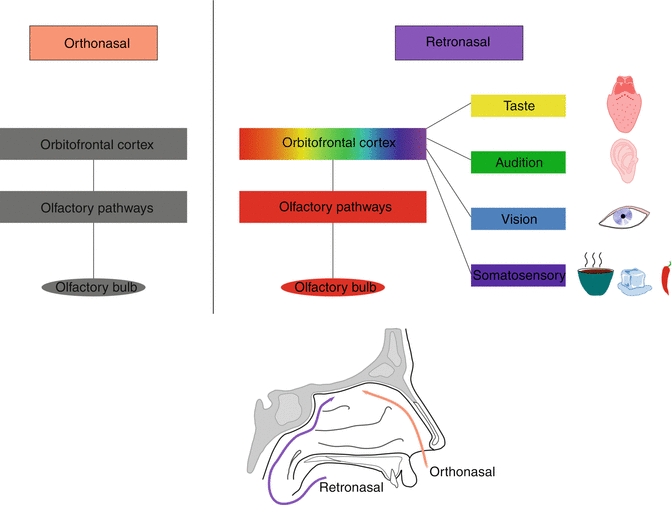
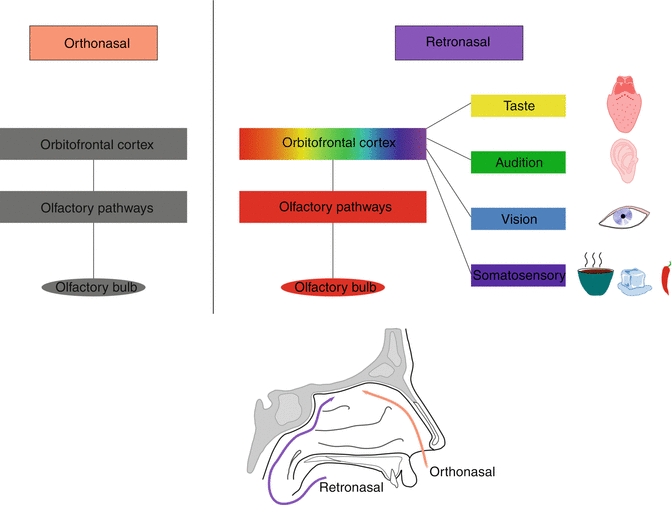
Fig. 10.4
Schematic representation of the central processing of orthonasal and retronasal olfaction. Orthonasal olfaction is processed by the olfactory pathways. On contrast, retronasal olfaction is not only processed by olfactory pathways but is also influenced by other sensory modalities, which are taste, sound, vision and proprioception. These multisensory informations are integrated in the orbitofrontal cortex
10.2.4 Olfactory and Trigeminal Interactions
The nasal fossa has double innervations from olfactory and trigeminal afferents. Although odorants are defined as volatile compounds having the ability to activate the olfactory system, the vast majority of odorants will actually activate both the olfactory and trigeminal system. Sensations resulting from the activation of the olfactory system are those of odors, while sensations induced by the stimulation of the trigeminal nerve are somatosensory (tactile, thermic, pain, humidity).
Olfactory and trigeminal systems closely interact with each other, and the stimulation of these two systems leads to important overlap in their activation pattern in the brain (Zald and Pardo 2000; Hummel et al. 2005; Boyle et al. 2007; Iannilli et al. 2007; Bensafi et al. 2008). The interaction between both systems is complex and takes place both at a peripheral and central level (for a review, see Hummel and Livermore 2002; Brand 2006). This interaction is difficult to predict, but it has a powerful influence on odor perception both at different concentration of a single stimulus and between mixtures of chemosensory stimuli. According to the literature, the pattern of interaction seems to depend on stimulus quality, intensity, and relative intensity of olfactory and trigeminal components of the mixture (for a review, see Hummel and Livermore 2002). Some reports have investigated the olfactory modulation of trigeminally mediated sensations in patients with olfactory loss, demonstrating that a close interaction and many compensatory mechanisms exist (Frasnelli et al. 2007).
10.2.5 Variability in Normal Olfactory Function
Such as other senses the olfactory function decreases over time, and it has been described in numerous previous studies that there is a strong decrease in olfactory function above the age of 55 years (Murphy et al. 2002; Hummel et al. 2007). Several mechanisms have been proposed to explain this age-related olfactory dysfunction. At a peripheral level, changes in mucociliary movement, mucus composition, submucosal blood flow, and epithelial thickness might disturb the transport of the odorant to the receptor (Rawson 2006). At the level of the neuroepithelium, it is assumed that the regeneration of olfactory receptor neurons decreases over age (Naessen 1971; Conley et al. 2003). At a central level, brain damages due to chronic ischemia or systemic disturbance might also be proposed as a potential cause of age-related olfactory disorder.
Hummel et al. reported that there is a differential change of olfactory functions with aging. Indeed, olfactory thresholds decrease more strongly with age as compared to odor discrimination and odor identification (Hummel et al. 2002a, 2007). Since threshold measurements best reflect the function of the peripheral olfactory system than other olfactory tests (Jones-Gotman and Zatorre 1988; Hornung et al. 1998; Moberg et al. 1999), this finding might indicate that age-related change of olfactory function is at least in part due to damage of the olfactory epithelium (Hummel et al. 2007). Nevertheless, we should also keep in mind that age-related decrease of olfactory function might also be a consequence of side effects of drugs, onset of neurodegenerative diseases, etc.
A sex-related difference in olfactory function has also been widely reported (Doty et al. 1985; Brand and Millot 2001; Lundstrom et al. 2005; Lundstrom and Hummel 2006; Hummel et al. 2007), with women outperforming men. Several causes have been proposed to explain this phenomenon, such as hormonal effects and congenital factors. However, the origin of this sex-related difference is still unclear.
Finally, some healthy people might present a specific anosmia. That is a physiological condition where a person of otherwise normal olfactory acuity is unable to detect a specific odorant. Specific anosmias have been described for series of odors (Amoore 1991). It is admitted that specific anosmia has a genetic basis, and the occurrence of specific anosmia indicates that specific receptors are necessary for perceiving specific odors (Amoore et al. 1968; Wysocki and Beauchamp 1984; Gross-Isseroff et al. 1992; Lancet et al. 1993; Menashe et al. 2003). One of the most frequent and well-known specific anosmia is androstenone anosmia. The prevalence of this specific anosmia is still a matter of debate. It is usually admitted that about 30 % of the population is unable to detect the odor of the androstenone. But there is a high variability in the prevalence reported in the literature, ranging from 1.8 to 75 % (for a review, see Bremner et al. 2003). This might be at least in part explained by the various stimulation methods, criterion for non-detection, and concentrations that were used in the different studies (Bremner et al. 2003).
10.3 Pathology
Although olfaction is often described as one of the less important sense, smell disorders have severe consequences, including impaired quality of life, daily life problems (cooking, detection of potentially dangerous odors) (Temmel et al. 2002), altered food choices and consumption patterns that can negatively impact health (decreased body weight, overuse of salt inducing blood hypertension, overuse of sugar inducing diabetes mellitus, impaired immunity etc.), and even depression (Deems et al. 1991).
The incidence of olfactory dysfunction among the population is still a matter of debate. Authors report an incidence of 1–3 % of dysfunction among population (Hoffman et al. 1998; Murphy et al. 2002). Nevertheless a study by Landis et al. reported higher values of olfactory dysfunction among population without sinonasal complaints, with a rate of 4.7 % of anosmia and 16 % of hyposmia. The frequency of parosmia and phantosmia were reported with a rate of 2.1 and 0.8 %, respectively (Landis et al. 2004).
The evaluation of patients suffering from olfactory disorders requires a precise clinical workup procedure in order to (1) determine the etiology of the olfactory dysfunction, (2) assess olfactory function, and, hence, (3) provide an optional treatment, a prognosis, and appropriate counseling to patients. Assessment of olfactory function is reviewed in Chapter 33.
Table 10.1
Classification of smell disorders
Quantitative smell disorders | Qualitative smell disorders |
---|---|
Hyposmia | Parosmia |
Anosmia | Phantosmia |
Functional anosmia | Olfactory agnosia |
Specific anosmia | |
Hyperosmia |
10.3.1 Classification of Olfactory Disorders
10.3.1.1 Quantitative Olfactory Disorders
Quantitative olfactory disorders are hyposmia, hyperosmia, and anosmia (Table 10.1). Hyposmia refers to a decreased ability to smell. This is a common condition. Indeed, Landis et al. reported that up to 16 % of the general population is hyposmic (Landis et al. 2004).
Hyperosmia is a rare condition and refers to enhanced ability to smell. It can happen after exposure to toxic vapors (Henkin 1990) or during migraine (Blau and Solomon 1985).
Anosmia refers to the lack of ability to smell. It is assumed that about 5 % of the general population exhibit functional anosmia (Landis et al. 2004). Functional anosmia refers to a significantly reduced ability to smell although some smell sensations can be present.
10.3.1.2 Qualitative Olfactory Disorders
Qualitative olfactory disorders are parosmia, phantosmia, and olfactory agnosia. Parosmia is a sensation that a given odor is different than the typical odor for this substance. Parosmia is typically associated with reduced olfactory sensitivity and is particularly frequent in patients suffering from postinfectious olfactory loss (up to 50 %) (Reden et al. 2007). It is also associated with posttraumatic olfactory loss or sinonasal-related olfactory disorder. Studies found a prevalence of parosmia in 19 % (Nordin et al. 1996), 20 % (Landis et al. 2010), and 28 % (Reden et al. 2007) of patients presenting to “smell and taste” clinics, while the prevalence of parosmia in the general population is reported to be 2.1 % (Landis et al. 2004) to 4 % (Nordin et al. 2007). It is typically unpleasant. Euosmia is a rare form of parosmia with a pleasant parosmia to selected odorants (Landis et al. 2006). The pathophysiology of parosmia is not clear. There are two hypotheses: the central and the peripheral hypotheses. In periphery, loss of olfactory receptor neurons changes the integrity of the olfactory image, resulting in an incomplete and meaningless picture of the odorant. Centrally, it has been proposed that the integration and interpretation of odors are altered (Leopold 2002).
Phantosmia is the perception of an odor when none is present. It may be reed to a wide range of pathologies (postinfectious olfactory loss, posttraumatic olfactory loss, rhinosinusitis, neurologic, etc.).
Finally, olfactory agnosia is defined as the inability to recognize odor sensation.
10.3.2 Etiology of Olfactory Disorders
There are several causes of olfactory dysfunction. The most frequent are chronic rhinosinusitis, postinfectious olfactory loss, and posttraumatic olfactory loss. These three etiologies account for up to two-third of the patients with olfactory disorder (Murphy et al. 2003; Rombaux et al. 2009b); therefore, we will largely extend on these three pathologies. However, several pathologies might also affect olfactory function, such as neurological disease, metabolic diseases, toxics, and tumoral disease of the sinonasal cavities or brain (Table 10.2). It is therefore essential to investigate about the etiology of olfactory dysfunction. An algorithm for the management of olfactory dysfunction is proposed in Fig. 10.5.
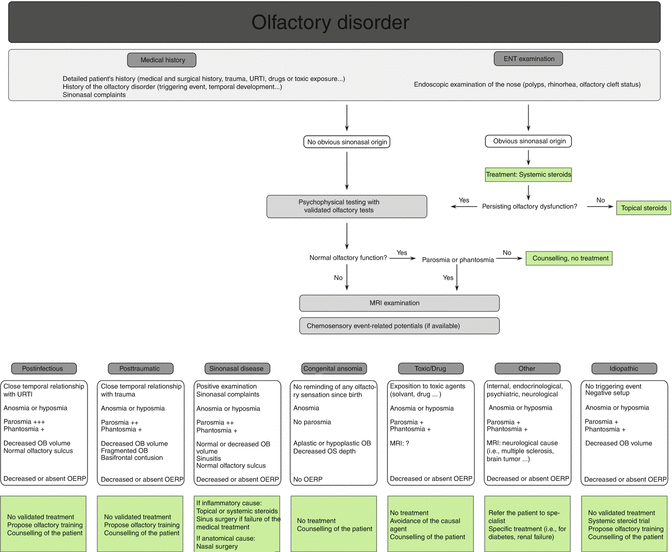
Table 10.2
This table summarizes the different etiologies of olfactory disorders
Rhinologic disease |
Chronic rhinosinusitis (with or without nasal polyps) |
Atrophic rhinitis (Huart et al. 2012) |
Olfactory cleft syndrome |
Postinfectious olfactory loss |
Posttraumatic olfactory loss |
Congenital anosmia |
Neurological disorder |
Alzheimer’s disease |
Idiopathic Parkinson’s disease |
Tumor |
Intranasal |
Esthesioneuroblastoma |
Adenocarcinoma |
Intracranial |
Gliomas |
Olfactory meningiomas |
Metals (cadmium, manganese, mercury, aluminum) |
Gases (formaldehyde, methyl bromide, styrene, chlorine) |
Solvents (toluene, butyl acetate, benzene) |
Hairdressing chemicals |
Intranasal zinc |
Chemotherapy drugs |
Analgesic (antipyrine) |
Local anesthetics (cocaine HCl, procaine HCl, tetracaine HCl, lidocaine) |
General anesthetics |
Antimicrobial (amoxicillin, aminoglycosides, macrolides, doxycycline, pyrazinamide) |
Antirheumatics (mercury/gold salts, d-penicillamine) |
Antithyroids (propylthiouracil, thiouracil) |
Cardiovascular, hypertensives (angiotensin conversion enzyme inhibitors, nifedipine, amlodipine) |
Gastric medication (cimetidine) |
Intranasal saline solutions (with acetylcholine, menthol, zinc sulfate) |
Opiates |
Sympathicomimetics |
Metabolic/endocrine (for a review, see Schiffman 1997) |
Adrenocortical insufficiency |
Cushing’s syndrome |
Hypothyroidism |
Pseudohypoparathyroidism |
Psychiatric (Turetsky et al. 2009) |
Idiopathic |
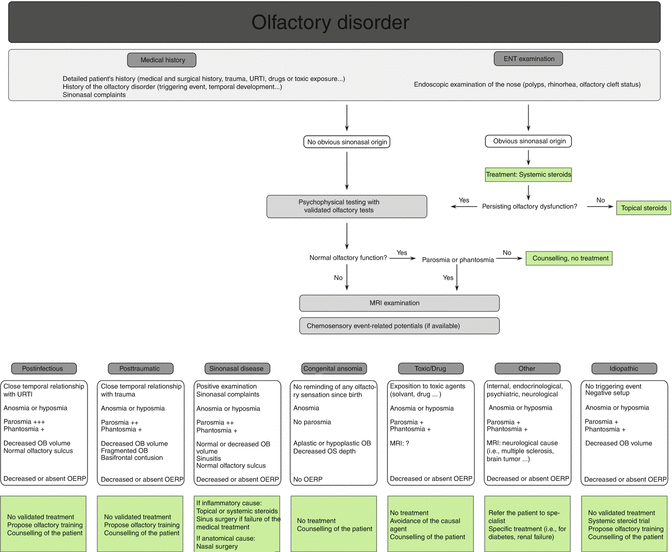
Fig. 10.5
Algorithm for the management of olfactory disorders. URTI upper respiratory tract infection, OB olfactory bulb, OS olfactory sulcus, OERP olfactory event-related potentials
10.3.3 Chronic Rhinosinusitis
In the literature, chronic rhinosinusitis (CRS) is described as the most common cause of olfactory dysfunction, accounting for 14–30 % of cases (Mott and Leopold 1991; Seiden and Duncan 2001; Raviv and Kern 2004; Holbrook and Leopold 2006). Inversely, olfactory impairment is a common symptom affecting 61–83 % of patients with CRS (Orlandi and Terrell 2002; Bhattacharyya 2003; Litvack et al. 2008; Soler et al. 2008). Nevertheless up to one-quarter of patients with CRS are unaware of their decreased olfactory abilities, probably because the olfactory dysfunction in CRS develops slowly, and in consequence only a few patients note this disorder (Nordin et al. 1995).
Psychophysical test results show that patients with CRS have quantitative disorders, between hyposmia and anosmia (Mott and Leopold 1991; Seiden and Duncan 2001; Raviv and Kern 2004; Holbrook and Leopold 2006; Welge-Luessen 2009), and may report fluctuating symptoms (Apter et al. 1999). Also it is widely known that patients with CRS with polyps have a higher incidence of smell symptoms and anosmia than patients with CRS without polyps (Hellings and Rombaux 2009). Some studies have described that the severity of quantitative disorders is related to the importance of the sinonasal disease (Litvack et al. 2008; Litvack et al. 2009a). Indeed, the mean endoscopy score and the mean CT score are significantly higher (more abnormal) in patients with hyposmia and anosmia than in patients with normosmia (Litvack et al. 2009a). Also, the opacification of the olfactory cleft on the CT scan seems to have a negative correlation with the olfactory function (Chang et al. 2009).
Patients with CRS not only report quantitative olfactory dysfunction but also qualitative dysfunction such as parosmia and phantosmia. However, these symptoms seem less frequent when related to sinonasal disease than to other etiologies (i.e., postinfectious, posttraumatic), and Reden et al. (2007) reported incidence of parosmia and phantosmia in patients with CRS of 28 and 7 %, respectively.
Traditionally, olfactory dysfunction in CRS is explained by a conductive olfactory loss, caused by swollen or hypertrophic nasal mucosa or nasal polyps, inducing an impaired access of odorants to the olfactory cleft. But clinical studies have failed to prove this hypothesis, as there is only little correlation between nasal resistance and the degree of olfactory dysfunction (Doty and Frye 1989). In addition, results of surgical therapy, although improving the nasal patency, are sometimes uncertain when considering the olfactory dysfunction.
Nowadays, it is generally agreed that the olfactory disturbance is due to an inflammatory process in the olfactory cleft (Konstantinidis et al. 2007) rather than a pure obstruction. Indeed, biopsies of the olfactory neuroepithelium in patients suffering from CRS revealed inflammatory and apoptotic pathological changes in the nasal mucosa, including the olfactory receptor neurons and olfactory supporting cells (Naessen 1971; Hellings and Rombaux 2009), and the degree of inflammation in the neuroepithelium was proved to be related to the severity of olfactory dysfunction (Kern 2000). This could be explained by the fact that inflammatory cells release inflammatory mediators, which are known to trigger hypersecretion in respiratory and Bowman’s glands (Getchell and Mellert 1991; Downey et al. 1996; Hellings and Rombaux 2009). Hypersecretion of Bowman’s gland is thought to alter the ion concentrations of olfactory mucus, affecting the olfactory transduction process (Joshi et al. 1987; Kern et al. 1997). Finally, it has been demonstrated that cytokines and mediators, particularly those released by eosinophils, may be toxic to olfactory receptor neurons (Nakashima et al. 1985; Apter et al. 1992).
Patients with nasal polyps show a higher incidence of olfactory disturbances and a higher incidence of anosmia than patients with CRS without polyps. This more severe symptomatology may be explained by the conductive olfactory loss induced by polyps but also by degenerative changes associated with recurrent infections, scarring, chronic nasal medication, exotoxins, and enhanced secretion of cytokines from Staphylococcus aureus infection and neurotoxic cytokines released by a huge eosinophilic population (Joshi et al. 1987; Vento et al. 2001; Litvack et al. 2008; Wang et al. 2010; Bernstein et al. 2011).
Medical imaging is useful in the assessment of patients suffering from sinonasal-related olfactory dysfunction. Using CT scan, Litvack et al. (2009a) have shown that the severity of quantitative olfactory disorder is associated with the importance of the sinonasal disease and that mean CT score is significantly higher in patients with hyposmia and anosmia than in normosmic patient. It was also demonstrated that the opacification of the olfactory cleft has a negative correlation with the olfactory function in patients with CRS and that it is significantly correlated with the postoperative olfactory results: patients with mild opacification having better postoperative results than patients with moderate and severe anterior olfactory cleft opacification (Kim et al. 2011). MRI is also interesting. Indeed, Rombaux et al. (2008) demonstrated that the olfactory bulb volume is correlated with the sinonasal disease score, and patients having a sinonasal disease score ≥12 significantly have larger olfactory bulb volume than patients with higher score. Smaller olfactory bulb volume is thus associated with a higher degree of sinonasal pathology. On contrast the olfactory function of the patients assessed with psychophysical testing was only slightly decreased or was even normal, emphasizing the idea that the olfactory bulb volume changes are more sensitive to subtle changes in the olfactory system than results of psychophysical testing.
Medical and/or surgical treatment must be proposed to patients suffering from sinonasal-related olfactory disorders since (1) they are effective in this pathology and (2) it has been demonstrated that patients reporting an improvement of their olfactory abilities have a better quality of life than patients reporting no improvement (Miwa et al. 2001). Only a few clinical studies have been conducted dealing with the improvement of olfactory function as a primary outcome in sinonasal disease treatment. Clinical trials of medical treatment for smell disorders associated with CRS have evaluated the efficacy of nasal and oral corticosteroid treatment. We found a recent study evaluating the efficacy of antihistamines (levocetirizine) on the smell loss in patients with persistent allergic rhinitis (Guilemany et al. 2012), but we found no studies about other drugs that are currently used in the treatment of CRS (antileukotrienes).
Corticosteroids with their potent anti-inflammatory effects are admitted to be the standard treatment for olfactory disorders induced by CRS. Their action mechanism on olfactory function might be explained by an inhibition of the release of proinflammatory mediators (i.e., cytokines, adhesion molecules, mast cells, basophiles, eosinophils) and a reduction in mucosa swelling (Mygind et al. 2001; Demoly 2008). Following EPOS 2012 recommendations, nasal steroids are recommended as the first-line treatment for CRS with or without nasal polyps (Fokkens et al. 2012). Studies have evaluated the efficacy of different topical corticosteroids such as betamethasone, flunisolide, mometasone furoate, fluticasone propionate, budesonide, and beclomethasone. Studies show that these drugs appear to be highly effective for most of the symptoms associated with CRS, including smell disorder, with a rapid onset of action and a cumulative effect after several days of use. In addition, they have the advantage of being a local therapy with limited side effects. Nevertheless the improvement in olfaction is frequently transient and incomplete (Lildholdt et al. 1995; Golding-Wood et al. 1996; Mott et al. 1997; Blomqvist et al. 2003; Stuck et al. 2003; Hellings and Rombaux 2009). Oral steroids are recommended in the treatment of CRS with nasal polyps as a second-line treatment (Fokkens et al. 2012). Several studies have investigated their efficacy in patients with CRS with or without polyps. They have shown that these potent anti-inflammatory drugs increase the olfactory function and they appear to be more effective than nasal steroids (Heilmann et al. 2004; Vaidyanathan et al. 2011). Moreover, an initial oral steroid therapy followed by topical steroid therapy seems to be more effective than topical steroid therapy alone (Vaidyanathan et al. 2011). Nevertheless oral steroids have important side effects if they are frequently administrated or if their administration is prolonged. Bonfils et al. (2006) evaluated the risk of oral steroid treatment in patients with CRS with nasal polyps and showed that almost 50 % of patients who received more than three short courses of oral steroid treatment had an asymptomatic adrenal insufficiency. Oral corticosteroids should thus be prescribed only if necessary and should be avoided if possible.
Functional endoscopic sinus surgery (FESS) is widely accepted as a treatment for chronic rhinosinusitis with or without nasal polyps after failure of the medical therapy. The only randomized study to attempt comparison between steroid therapy and polypectomy showed significant improvement of subjective and objective olfactory function in both groups, remaining for 1 year. However, these results should be tempered by the fact that the smell evaluation methodology was not described (Lildholdt 1989).
Several studies have investigated the effect of FESS on olfactory function (for a review, see Bonfils et al. 2009). Nevertheless the literature shows that there are major variations in the selection of patients for the surgery, and some studies have poor validity because of poorly defined patient groups, lack of clear inclusion or exclusion criteria, poor description of the surgical procedure, and poor description of the olfactory evaluation tool. In this literature, olfactory function was assessed either by subjective patient self-reported olfactory function or by semi-objective olfactory testing (i.e., UPSIT). Considering patient self-reported olfactory function, authors agree that FESS lead to a significant improvement of olfactory dysfunction (Levine 1990; Lund and MacKay 1994; Klossek et al. 1997; Jakobsen and Svendstrup 2000) (for a review, see Bonfils et al. 2009). Only few studies have investigated the effect of FESS on olfactory function by using semi-objective olfactory testing. They have also shown that FESS has a significant positive effect on olfactory function (Lund and Scadding 1994; Min et al. 1995; Downey et al. 1996; Klimek et al. 1997; Delank and Stoll 1998) (for a review, see Bonfils et al. 2009). Some authors have investigated the correlation between the severity of CRS and surgical outcomes on olfaction. It was reported that the improvement after FESS is significantly better in patients with severe olfactory dysfunction, whereas it is not in patients with mild olfactory dysfunction (Litvack et al. 2009b; Soler et al. 2010). The degree of nasal obstruction, the extent of the rhinosinusitis disease (evaluate by symptom score or CT scan), and the coexistence of nasal polyps or allergic rhinitis do not predict the possibility of olfactory improvement after FESS (Bhattacharyya 2006; Wright and Agrawal 2007; Jiang et al. 2009).
Finally, Gudziol et al. (2009) explored the influence of the treatment of CRS on the olfactory function. They measured olfactory bulb volume, using MRI, and olfactory function of patients suffering from CRS before treatment and 3 months after. They showed that the olfactory bulb volume significantly increases after treatment and that the increase of olfactory bulb volume correlated significantly with an increase in odor thresholds.
10.3.4 Postinfectious Olfactory Loss
Postinfectious olfactory loss is defined as a sudden loss of olfactory function following an upper respiratory tract infection (URTI) and was described for the first time more than 20 years ago (Henkin et al. 1975). The upper respiratory infection subsides over time and leaves the patient with an olfactory dysfunction that persists over a long period. There is a close connection in time between the URTI and the onset of the olfactory disorder (Seiden 2004). The exact pathogenic agent is rarely determined but is assumed to be viral, and so this disease is known as “post-viral” or “postinfectious” olfactory loss. The exact incidence of olfactory dysfunction following URTI is not known as many patients with URTI do not report their symptoms, so the exact incidence of common cold in the population is unknown. However, postinfectious olfactory loss is diagnosed in approximately one-quarter of the patients in groups presenting to specialized centers such as smell and taste clinics (Cain et al. 1988; Deems et al. 1991; Sugiura et al. 1998; Bonfils et al. 1999).
Patients with postinfectious olfactory loss are usually women, and the disease typically occurs between the fourth and the sixth decades of life (Sugiura et al. 1998; Seiden 2004; Rombaux et al. 2009a). Onset of the URTI is often sudden and awareness of the olfactory dysfunction is present when major symptoms secondary to the infection subside. Many patients also have endoscopic or radiological evidence of rhinosinusitis. It is therefore mandatory to treat this condition and observe the impact of this treatment on the sensorineural disorder. Patients usually complain of moderate to severe olfactory loss, but the degree of olfactory loss is usually less severe than in patients with head trauma (Duncan and Seiden 1995). Parosmia and phantosmia are also present and range to 10–50 % (Henkin et al. 1975; Leopold et al. 1991; Reden et al. 2007). Seasonal variation in the incidence of postinfectious olfactory loss has been demonstrated with the highest incidences being in March and May (Konstantinidis et al. 2006). This is probably due to the seasonal variation of viral particles such as parainfluenza virus type 3 (Sugiura et al. 1998; Suzuki et al. 2007; Wang et al. 2007).
Diagnosis should be based on (1) history of an olfactory disorder following a URTI and a close temporal relationship between the two, (2) patency of the olfactory cleft at the endoscopic examination, and (3) absence of any other causes such as toxic exposure (medication taken to treat the URTI and possibly causing an olfactory disorder themselves), an inflammatory process in the nasal fossa (diagnosed with an endoscopic evaluation), or neurological problems such as neurodegenerative diseases.
The exact mechanism leading to postinfectious olfactory loss is not yet fully understood. Viral particles may damage the olfactory receptor neuron and provoke immune response that also leads to damages in the olfactory neuroepithelium and damages the central olfactory pathways. Viruses are also capable of penetrating the brain via the fovea ethmoidalis. Many viruses may cause olfactory impairment, examples being the influenza virus, parainfluenza virus, respiratory syncytial virus, Coxsackie virus, adenovirus, poliovirus, enterovirus, and herpes virus. The exact determination of the viral agent is not useful in the clinic and viral serology is not mandatory. Experimental intranasal infection with influenza virus A leads to increased apoptosis and increased fibrosis in the olfactory neuroepithelium (Mori et al. 2002, 2004). This mechanism is thought of as a protective one that limits the access of viral particles to the brain. Histopathological findings relating to the olfactory neuroepithelium of patients with postinfectious olfactory loss have revealed that severely affected patients have reduced numbers of ciliated olfactory receptor cells (Doty 2008). Moreover, dendrites of the olfactory receptor neurons usually fail to reach the epithelial surface and therefore have no contact with odorant particles. Attempting to correlate the importance of the olfactory neuroepithelial damage with the extent of the olfactory dysfunction as well with the chances of recovery generated conflicting results (Yamagishi et al. 1994; Doty 2008). Overall, postinfectious olfactory loss is probably secondary to a viral attack both at a peripheral level (olfactory neuroepithelium) and at a central level (olfactory bulb), and these two sites interact both in the pathological condition and in the recovery phase.
MRI findings show that olfactory bulb is reduced in patients with postinfectious olfactory loss, and there is a strong correlation between olfactory bulb volume and the olfactory dysfunction, with the lowest olfactory bulb volume being in patients with severe olfactory dysfunction and parosmia (Mueller et al. 2005; Rombaux et al. 2006).
At present there is no medical therapy that has been proven effective. Many drugs have been tried in nonrandomized and uncontrolled trials: topical or systemic corticosteroids (Heilmann et al. 2004), zinc sulfate (Henkin et al. 1976; Aiba et al. 1998), quinoxaline derivative (Quint et al. 2002), alpha lipoic acid (Hummel et al. 2002b), and pentoxifylline (Gudziol and Hummel 2009). Although early promising results with some molecules have been demonstrated, these medications helped patients to achieve partial or full recovery in unpredictable ways (Hummel 2000). Olfactory training has also been provided with some interesting results: 28 % of the patients achieved olfactory improvement (Hummel et al. 2009). Olfactory training was given for 12 weeks based on four different odors (phenylethyl alcohol, eucalyptus, lemon, cloves) and was required at least 10 min twice a day by this protocol. For patients with qualitative disorders such as phantosmia or parosmia, some authors advocate the surgical removal of the neuroepithelium, possibly damaging quantitative capacity but resolving the qualitative problem (Leopold et al. 2002). This option has been adopted in very few cases. Although such treatment is based on empirical grounds, patients with second or multiple episodes of olfactory dysfunction during an URTI should receive corticoid treatment if the olfactory loss persists after the URTI symptoms in order to reduce the risk of viral injury and permanent olfactory dysfunction.
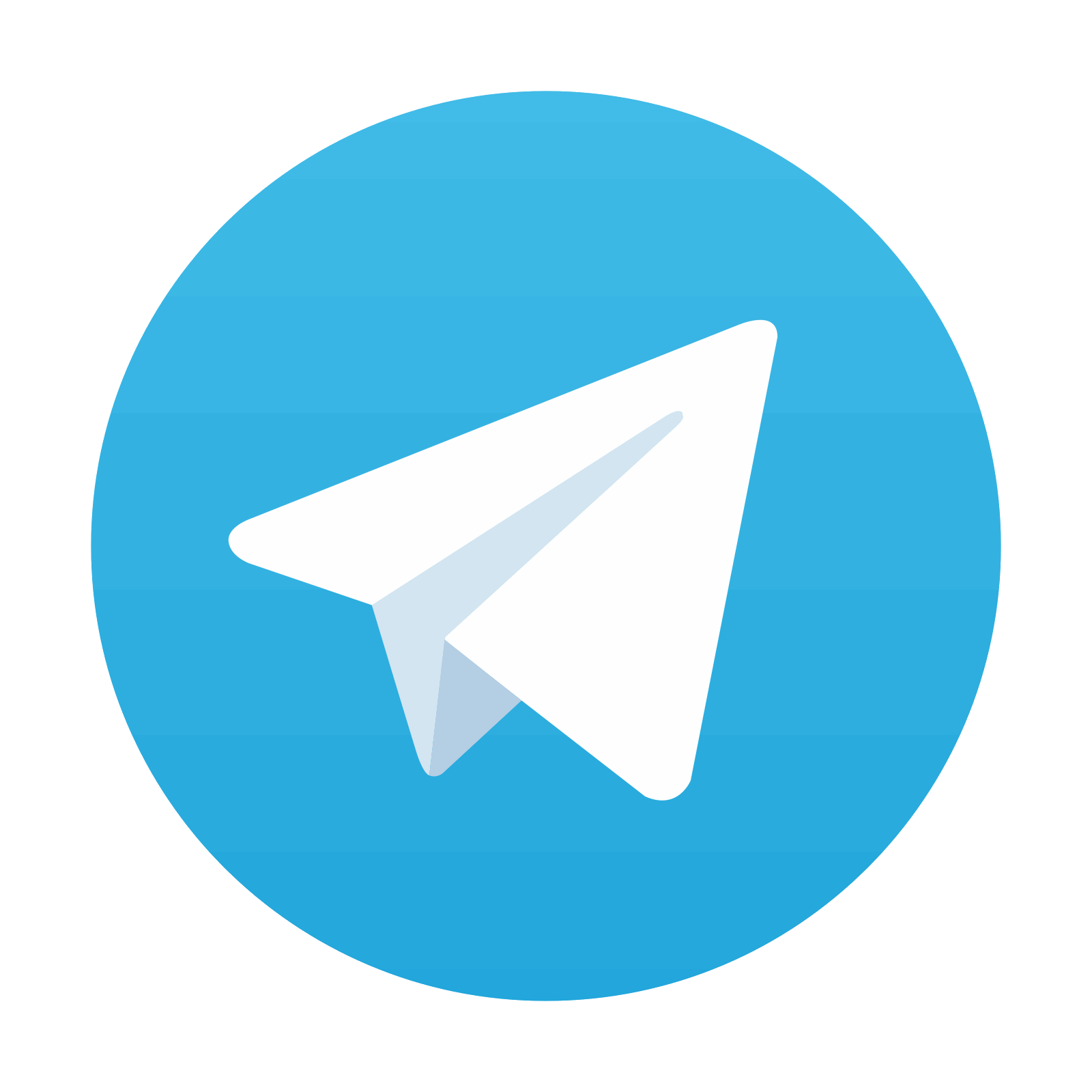
Stay updated, free articles. Join our Telegram channel

Full access? Get Clinical Tree
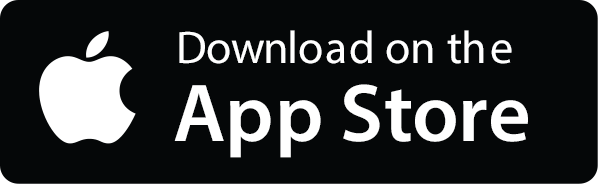
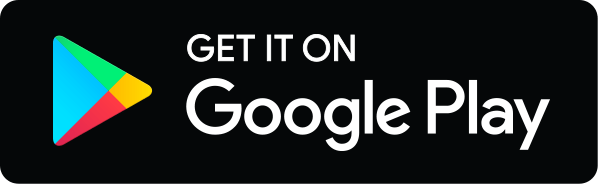