Grade
ASHA Guidelines (1994)
BIAP (1996)a
WHO Global Burden of Disease 2000 (Mathers et al. 2003)a
Münster classification (Schmidt et al. 2007)
CTCAE v.4.0 NIH NCI (2010)
Chang Practical Grading System (Chang and Chinosornvatana 2010)
SIOP Boston Ototoxicity Scale (Brock et al. 2012)
WHO Global Burden of Disease (2013)a
0
I. Normal or subnormal: <20 dB
0: No impairment: ≤25 dB HL
0: No impairment: ≤10 dB HL at all frequencies
0: ≤20 dB HL at 1, 2 and 4 kHz
0: ≤20 dB HL at all frequencies
1
(A) 20 dB or greater decrease in pure-tone threshold at any test frequency
II. Mild: 21–40 dB
1: Slight impairment: 26–40 dB HL
1: Onset of impairment: >10 and ≤20 dB HL at one or more frequencies, or tinnitus
1: Mild; asymptomatic or mild symptoms; clinical or diagnostic observations only; intervention not indicated: In adults 15–25 dB HL at two contiguous frequencies/subjective change; in children >20 dB HL at 8 kHz
1a: ≥40 dB HL at any frequency 6–12 kHz
1: >20 dB HL above 4 kHz
Mild: 20–34 dB HL
1b: >20 and <40 dB HL at 4 kHz
2
(B) 10 dB or greater decrease at two adjacent test frequencies
III. Moderate:
1st degree: 41–55 dB
2nd degree: 56–70 dB
2: Moderate impairment: 41–60 dB HL
2: Moderate impairment: >20 dB HL at 4 kHz and above
2: Moderate; minimal intervention indicated:
In adults >25 dB HL at two contiguous frequencies/limiting instrumental ADL; in children >20 dB HL at 4 kHz and above
2a: ≥40 dB HL at 4 kHz and above
2: >20 dB HL at 4 kHz and above
Moderate: 35–49 dB HL
2a: >20 to ≤40 dB HL
2b: >20 dB HL and <40 dB at any freq below 4 kHz
Moderately severe: 50–64 dB HL
2b: >40 to ≤60 dB HL
2c: >60 dB HL
IV. Severe:
1st degree: 71–80 dB
2nd degree: 81–90 dB
3
(C) Loss of response at three consecutive test frequencies where responses were previously obtained
V. Very severe:
1st degree: 91–100 dB
2nd degree: 101–110 dB
3rd degree: 111–119 dB
3: Severe impairment:
61–80 dB HL
3: Considerable impairment, rehabilitation with hearing aids indicated: >20 dB HL at <4 kHz
3: Severe or medically significant; disabling:
In adults >25 dB HL at three contiguous frequencies/therapeutic intervention indicated/limiting self-care ADL; in children >20 dB HL at 3 kHz and above
3: ≥40 dB HL at 2 or 3 kHz
3: >20 dB HL at 2 or 3 kHz and above
Severe: 65–79 dB HL
3a: >20 to ≤40 dB HL
3b: >40 to ≤60 dB HL
3c: >60 dB HL
Profound: 80–94 dB HL
4
VI. Total hearing loss: >120 dB
4: Profound impairment including deafness: ≥81 dB HL
4: Loss of function, cochlear implant indicated: ≥80 dB HL at <4 kHz
4: Life-threatening consequences; urgent intervention indicated: In adults >80 dB HL at 2 kHz and above; in children cochlear implant indicated
4: ≥40 dB HL at 1 kHz and above
4: >40 dB HL at 2 kHz and above
Complete: ≥95 dB HL
14.2 Epidemiology of Hearing Disorders in Children
14.2.1 Introduction
the study of the distribution and determinants of disease frequency in [the] human population.
Davis et al. (2004)
the proportion of individuals with a defined type of hearing impairment in a specified population cohort.
Sancho et al. (1998), cited in Davis et al. (2004)
This is typically expressed as a percentage of that population or the number of affected individuals per thousand of the population. The term incidence means the number of new cases within a defined time period.
Various figures for the prevalence of hearing disorders in children have been reported, such as 1.3 per 1000 (Fortnum and Davis 1997), 5.6 per 1000 (Watkin et al. 1990) and 1.4% (Stevens et al. 2013). Faced with such differing prevalence values, we need to know the details behind each study. When factors such as differences in the study populations and definitions of hearing impairment are taken into account, the prevalence emerging from these studies is more clearly comparable, resulting in the figures 1.3 per 1000 (Fortnum and Davis 1997), 2.1 per 1000 (Watkin et al. 1990) and 4.0 per 1000 (Stevens et al. 2013). The following section goes into greater detail about these and other examples.
14.2.2 Prevalence of Childhood Hearing Loss and Case Definition
Fortnum and Davis (1997) reported the prevalence of permanent childhood hearing impairment as 1.3 per 1000. The population sampled was all children born and resident within a defined regional health authority within the UK between 1985 and 1993 (552,558 live births). Hearing loss in this study was defined as a permanent hearing threshold of ≥40 dB HL in the better ear, averaged across 0.5, 1, 2 and 4 kHz, including sensorineural, permanent conductive and mixed impairments. 0.73 per 1000 were moderate hearing losses (40–69 dB HL), 0.28 were severe (70–94 dB HL) and 0.31 were profound (≥95 dB HL).
Watkin et al. (1990) studied the population of children born between 1973 and 1988 in a different UK regional health authority and reported a prevalence of 5.6 per 1000. In this case, hearing loss was defined as permanent bilateral thresholds >21 dB HL averaged in the better ear or unilateral hearing loss >55 dB HL in the affected ear. 1.3 per 1000 had mild hearing loss (21–40 dB HL), 0.8 moderate (41–70 dB HL), 0.4 severe (71–95 dB HL) and 0.7 profound (>95 dB HL). Unilateral hearing losses accounted for 2.4 per 1000. Once the unilateral and mild bilateral hearing losses were removed, the prevalence rate was reduced to 2.1 per 1000.
In 2013, the World Health Organization’s Global Burden of Disease Hearing Loss Expert Group reported the global prevalence of hearing impairment in children aged 5–14 years old as 1.4%, which equates to 16 million children, based on a meta-review of 42 studies across 29 countries (Stevens et al. 2013). Prevalence was lowest in high-income countries (Western Europe, Australia and the USA) at 0.4% and highest in South Asia at 2.2%. ‘Hearing impairment’ was defined here as an average hearing threshold of ≥35 dB HL in the better ear calculated across 0.5, 1, 2 and 4 kHz. Data were taken only from studies that reported their methodology in detail, had adequate sample sizes, had response rates ≥80% and where study samples were representative of the target population. All contributing studies had to report detailed audiological data (thresholds and frequencies tested) and include audiological test techniques of sufficient quality (e.g. specific minimum background noise levels), but no distinction between temporary and permanent hearing loss was made.
The differences between the three examples cited above are largely removed once the definitions of hearing loss used in each case and the sample populations are considered. Watkin’s results are comparable to Fortnum and Davis when mild and unilateral hearing impairments are excluded (2.1 per 1000 and 1.4 per thousand, respectively). When focusing on high-income countries, the WHO figures are reduced to 4.0 per 1000. The remaining difference of 1.9–2.6 per thousand between the WHO and the two UK-based studies may be accounted for by the lower threshold used by the WHO to define hearing loss (35 dB HL rather than 40 dB HL) and inclusion of temporary hearing impairments.
Other factors may also contribute to such differences as discussed above. Age range, for example, is crucial, as highlighted by Morton and Nance (2006) who reported increasing prevalence of hearing loss within the US increasing from 1.86 per 1000 at birth to approx. 2.7 per 1000 by 5 years old and 3.5 per 1000 during adolescence (hearing loss here defined as bilateral or unilateral sensorineural loss of >35 dB HL). All of these prevalence figures are legitimate but their specific contexts must be understood.
Two relatively recent studies analyse changes in the prevalence of hearing loss over time. Shargorodsky et al. (2010) compared the data gathered on teenagers between 1988–1994 and 2005–2006. The authors found a 77% increase in the prevalence of uni- and bilateral hearing loss ≥25 dB HL (air-conduction thresholds averaged across frequencies) over this time period (from 3.2 to 5.3% of the US teen population). Van Naarden Braun et al. (2015) used data on 8-year-old children during each of the years 1991–2010, finding an average prevalence of 1.4 per 1000 (bilateral thresholds ≥40 dB HL averaged over 500–2000 Hz) with no significant change across the 20-year time period. Comparing WHO data from 1985 to 2011, the prevalence of hearing disability has risen about eightfold, partly because of the inclusion of lower hearing loss grades in the definition but also due to—among others—increasing presbyacusis, earlier detection of hearing loss and increasing use of ototoxic medication (Olusanya et al. 2014).
14.2.3 Normal Hearing in Children
Normal-hearing in adults, which has been set as 0 dB HL for purposes of audiometric measurement, is defined as the average of the lowest distinguishable sound pressure level measurable in young adults. This level has been studied and revised several times over the years (International Organization for Standardization (2010) 8253-1), so when comparing auditory thresholds described in the literature, the fact that the baseline may not always be the same has to be taken into account.
In children there is no clear definition of normal-hearing threshold in tone audiometry because hearing sensitivity changes with age and results from different hearing test methods of differing sensitivity and considerable variation (especially in subjective tests) also change with age. In general, children and adolescents often show lower hearing thresholds than the audiometric zero. For example, one study found that 75% of children aged from 5 to 14 years had hearing threshold levels better than audiometric zero (calibration according to the American standard specification for audiometers for general diagnostic purposes Z24.5-1951) (Eagles et al. 1963). Two other studies found that over 50% of children and adolescents showed lower threshold results than the 1951 ASA audiometric zero (Roberts and Ahuja 1975; Roberts and Huber 1970), but when compared with the audiometric zero from ANSI 1969, a substantially lower percentage showed results under zero (Roberts and Ahuja 1975).
Another problem that has to be considered is differences in children’s sensitivity or responsiveness to different stimuli, with pure tones, warble tones, narrowband noise and FRESH noise (frequency-specific hearing assessment noise, a narrowband noise with steep filter slopes (see Moore and Violetto (2016)) being the most commonly used nowadays). Greatest sensitivity/responsiveness has been found in response to warble tones (Orchik and Rintelmann 1978; Walker et al. 1984). Close agreement between warble tone and pure-tone thresholds has been found in adults (Dockum and Robinson 1975) with equally high test-retest reliability for both types of stimuli (Staab and Rintelmann 1972).
Behavioural observation audiometry is the most common subjective hearing test used in babies, with visual reinforcement audiometry (VRA) being the standard from about 8 months of age and play audiometry from approximately 2.5 years of age. These different methods are described in Sect. 16.4.
Babies and young children generally show auditory responses at higher levels than adult hearing thresholds, and this is reflected in the common use of the term ‘minimal response level’ instead of ‘threshold’. Increasing hearing sensitivity can be seen with increasing age. This can be explained by the greater test sensitivity and compliance with age but also by maturation of the auditory pathway after birth (Talero-Gutierrez et al. 2008) and by changes in the anatomy of the middle and external ear (Schneider et al. 1980).
Overview of studies of normal-hearing thresholds in childhood
Group | Age | Stimulus, calibration | Method | Criteria for patient selection | Results (special remarks, explanation for given thresholds) Mean threshold (standard deviation) | Normal-hearing thresholds/minimal response levels (dB HL/dB SPL where given) at frequency (Hz) (std. deviation) | Source | |||||||||
---|---|---|---|---|---|---|---|---|---|---|---|---|---|---|---|---|
125 | 250 | 500 | 1000 | 2000 | 3000 | 4000 | 6000 | 8000 | 10,000 | |||||||
USA, n = 132 | 6 months | 250 ms tones, Earphones | Tone burst VRA, go/no-go | Normal and healthy upon paediatric examination | 23 | 20 | 19 | Berg and Smith (1983) | ||||||||
10 months | 27 | 17 | 23 | |||||||||||||
10 months | Sound field | 20 | 18 | 21 | ||||||||||||
14 months | 19 | 20 | 21 | |||||||||||||
18 months | 19 | 18 | 21 | |||||||||||||
USA, n = 22 | 8 months | 30-s Pure tones, sound field | Behavioural observation, 2 observers, ascending method of limits | Screened to eliminate “high-risk” babies and considered to be developmentally normal | 45 | 50 | Hoversten and Moncur (1969) | |||||||||
Sweden, n = 120 | 3 months to 1 year | Pure tones | VRA mean monaural sound field thresholds/conditioned orientation reflex audiometry-mean binaural thresholds | Reported normal hearing, clinical exam | Estimated threshold from figures | 29 | 31 | 30 | 33 | 33 | Liden and Kankkunen (1969) | |||||
1–2 years | 29/33 | 29/33 | 34/34 | 29/34 | 32/40 | |||||||||||
2–3 years | 24/31 | 21/31 | 20/32 | 21/34 | 23/40 | |||||||||||
3–4 years | 14/30 | 14/33 | 13/31 | 15/34 | 18/40 | |||||||||||
4–5 years | 12/26 | 12/26 | 10/26 | 10/27 | 12/29 | |||||||||||
5–6 years | 12/22 | 9/19 | 7/24 | 7/23 | 8/23 | |||||||||||
USA, n = 39 | 8–24 months | Warble tone | Sound field, 2 speakers, 125° left/right of child, Two clinicians, Hughson-Westlake procedure | Negative otological history, normal acoustic immittance, normal development, good health | 29.0 | 28.0 | McDermott and Hodgson (1982) | |||||||||
25–36 months | 29.0 | 27.8 | ||||||||||||||
37–48 months | 23.9 | 25.0 | ||||||||||||||
8–24 months | Narrow band noise | 22.0 | 25.0 | |||||||||||||
25–36 months | 25.9 | 24.4 | ||||||||||||||
37–48 months | 22.9 | 23.6 | ||||||||||||||
USA, n = 9 | 6–7 months | 2-s, 5% warbled tones | Supra-aural headphones | 38 | 25 | 23 | Moore and Wilson (1978) | |||||||||
n = 10 | 12–13 months | 31 | 27 | 25 | ||||||||||||
n = 9 | 6–7 months | Sound field | 18 | 18 | 18 | |||||||||||
n = 10 | 12–13 months | 21 | 16 | 14 | ||||||||||||
USA, screened n = 11 | 6 months | 500-ms pure tones | Supra aural headphone VRA, go/no-go | If screened: Tympanometry pressure peak >−100 daPa H2O | Mean threshold (Standard Deviation) | 21 (5.72) | 16 (4.2) | Nozza and Wilson (1984) | ||||||||
n = 17 unscreened | 23 (7.3) | 20 (10.00) | ||||||||||||||
n = 12 screened | 12 months | 18 (3.62) | 14 (6.10) | |||||||||||||
n = 17 unscreened | 22 (9.4) | 15 (6.75) | ||||||||||||||
USA, n = 11 | 8–11 months | 1 s pure tones | Insert earphones, left ear, operant head turn with VRA, yes/no, infants with >25% false-positive rate on two occasions were excluded from the study | Tympanometric screening | 12.6 (3.7) | Nozza (1995) | ||||||||||
USA, n = 10–28 | 3 months | Pure tone | VRA, observer-based psychoacoustic procedure | Full-term birth, no complications, normal postnatal development, never diagnosed hearing loss, no colds, no middle ear infection <3 weeks before testing, no >2 prior ear infection, no family history of congenital hearing loss | Estimated values from Fig. 4 in reference | 47 | 41 | 30 | 26 | 23 | 36 | Olsho et al. (1988) | ||||
n = 10–22 | 6 months | 47 | 30 | 21 | 12 | 14 | 16 | |||||||||
n = 10–11 | 12 months | 43 | 32 | 22 | 10 | 13 | 21 | |||||||||
UK, n = 46 | 8–11 months | Warble tones | Insert earphone VRA, 2 testers, 1–2 visits | Screening passed, tympanometry, TEOAE, development questionnaire | Mean minimal response levels | 16.4 | 13.3 | 7.1 | 6.4 | Parry et al. (2003) | ||||||
Canada, n = 23–31 per freq./age | 6 months | Half-octave bands | Refined VRA, free-field, 2 speakers 45° right/left from baby | – | 19,000 Hz: 6 months: 41 dB 12/18 months: 38 dB 24 months: 31 dB | 24 | Schneider et al. (1980) | |||||||||
12, 18 months | 22 | |||||||||||||||
24 months | 19 | |||||||||||||||
USA, n = 11–16 | 7–11 months | 1-s wave tones, sound field | VRA, go/no-go operant head-turning technique | Appeared in good health on test days | 37.7 | 30.3 | 31.2 | 36.1 | 34.3 | Sinnott et al. (1983) | ||||||
n = 2–7 | 0.5-s wave tones, sound field | 33.6 | 35.0 | 36.5 | 31.2 | 25.0 | ||||||||||
Canada, | Octave-band noise | VRA modification with 2 speakers in 45° | Free of cold | 200 Hz: | 400 Hz: | Trehub et al. (1980) | ||||||||||
n = 89 | 6 months | 38 | 33 | 25 | 28 | 23 | 18 | |||||||||
n = 74 | 12 months | 27 | 25 | 18 | 16 | 17 | 10 | |||||||||
n = 76 | 18 months | 31 | 24 | 19 | 20 | 14 | 19 | |||||||||
Brazil, n = 50 | 6–12 months | Instrumental sounds | VRA | Absence of risk indicators for hearing loss, presence of bilateral otoacoustic emissions, type A tympanometric curve | Average | 32.24 | 27.07 | 26.72 | 27.41 | Vieira and Azevedo (2007) | ||||||
13–24 months | 27.5 | 22.14 | 20.71 | 21.79 | ||||||||||||
24–34 months | 24.29 | 21.43 | 21.43 | 21.43 | ||||||||||||
USA, n = 2078 | 5 years | Octave band | Earphones, hand raising/play technique | Representative cross selection of Pittsburg school children, otoscopy | Increasing sensitivity with age, girls more sensitive than boys | 11.8 | 11.4 | 9.6 | 6.9 | 5.1 | 9.4 | 12.6 | Eagles et al. (1963) | |||
7 years | 6.6 | 8.5 | 6.1 | 4.5 | 2.9 | 7.7 | 8.8 | |||||||||
10 years | 6.2 | 7.3 | 5.7 | 5.1 | 2.8 | 7.9 | 8.7 | |||||||||
14 years | 6.2 | 7.0 | 6.0 | 5.5 | 4.4 | 9.2 | 9.3 | |||||||||
Finland, n = 471 | 7 years | Pure tones, earphones | Pure tone audiometry with bracketing method | Healthy tympanic membrane, normal objective acoustic immittance tympanometry and acoustic reflex measures | 10.2 | 6.9 | 3.7 | 0.8 | 0.7 | 1.6 | 1.2 | 6.1 | 5.8 | Haapaniemi (1996) | ||
10 years | 5.9 | 2.7 | 0.1 | −1.2 | −5.1 | −0.7 | −0.8 | 3.3 | 2.6 | |||||||
14 years | 5.2 | 2.6 | −0.7 | −1.5 | −1.8 | −0.5 | −1.4 | 4.8 | 2.1 | |||||||
USA, n = 6166 | 6–11 years | ANSI S3.6–1969 | Screening questionnaire | AC., average left/right ear, male:female | 7.2:7.1 | 5.3:5.5 | 4.0:3.6 | 5.7:5.2 | 5.3:5.0 | 8.9:9.5 | 11.7:11.7 | Holmes et al. (2004) | ||||
12–19 years | 6.2:6.0 | 4.8:4.4 | 3.4:3.2 | 6.5:4.2 | 5.8:3.9 | 10.1:10.4 | 9.3:8.9 | |||||||||
Finland, n = 420 | 5 years | Pure tone, cal. ISO 389 std. | Air/bone conduction thresholds | No otological selection | AC., average left/right ear, male:female | 18.3:15.7 | 13.1:11.2 | 10.5:9.5 | 8.1:7.9 | 7.1:7.4 | 8.0:7.6 | 12.7:11.2 | 14.3:13.6 | Karma et al. (1989) | ||
USA, n = 20 | 3.5 years | Pure tone, warble tone, narrowband | Earphone 3.5/4.5 years: conditioned play audiometry 5.5/6.5 years: raising hand when sound was heard | Normally-hearing = passing a pure-tone screening test at the 20 dB hearing threshold level (re ANSI, 1969) | Estimated threshold from Fig. 1 in reference Thresholds for the three test stimuli (pure tone, warble tone and narrow-band noise) are combined | 25 | 16 | 15 | Orchik and Rintelmann (1978) | |||||||
4.5 years | 24 | 14 | 13 | |||||||||||||
5.5 years | 36 | 21 | 12 | 9 | 15 | |||||||||||
6.5 years | 33 | 18 | 10 | 10.5 | 13 | |||||||||||
Denmark, n = 46 | 10–12 years | 2 s pure tones | Quasi free-field, stimulus from a point source | Normal medical history, hearing anamnesis (no noise exposure, middle ear dysfunction), otoscopy, impedance | 4.02 | 25.33 | 28.75 | Osterhammel (1978) | ||||||||
Korea, n = 15,606 | 12–19 years | Pure tones, GSI SA-203 audiometer | Air cond., earphones, modified Hughson-Westlake procedure | Normal tympanic membrane and no history of regular or occupational noise exposure | Better ear, average 12–19 years, male:female | 7.2:6.9 | 2.0:1.9 | 1.7:2.1 | 1.9:1.6 | 1.9:2.0 | 9.0:10.6 | Park et al. (2016) | ||||
UK, n = 11370 | 7 years | Pure tone | Air conduction, audiometry locally done | No exclusion | Hearing loss in better ear | Estimated 50th percentile | 9.0 | 9.5 | 6.0 | 4.0 | 3.0 | 6.0 | Richardson et al. (1976) | |||
n = 12406 | 11 years | 9.5 | 9.5 | 5.0 | 2.5 | 1.5 | 3.0 | |||||||||
USA, n = 7119 | 6 years | Pure tone, 1951 American Standard | Air-conduction earphones | Non-institutionalised children, no otological selection | Median hearing levels of the better ears, male:female | −7.8:−7.7 | −7.3:−7.6 | −7.0:−7.2 | −8.1:−8.7 | -4.6:−5.5 | −4.1:−4.8 | −2.6:−3.0 | −4.7:−6.6 | Roberts and Huber (1970) | ||
7 years | −8.7:−8.3 | −7.6:−8.0 | −7.2:−7.8 | −8.8:−8.8 | −4.1:−5.3 | −4.7:−4.9 | −2.8:−2.7 | −6.4:−5.9 | ||||||||
8 years | −10.2:−10.1 | −9.3:−9.9 | −7.8:−8.6 | −9.4:−9.8 | −5.2:−5.9 | −5.5:−5.7 | −3.5:−4.0 | −6.9:−7.9 | ||||||||
9 years | −11.1:−9.6 | −10.0:−9.4 | −8.9:−8.3 | −10.1:−10.1 | −5.8:−5.2 | −5.9:−5.2 | −3.8:−3.5 | −7.6:−7.4 | ||||||||
10 years | −11.5:−10.8 | −10.5:−11.1 | −9.2:−9.8 | −9.6:−10.4 | −5.7:−6.4 | −5.6:−6.4 | −3.0:4.0 | −7.7:−7.5 | ||||||||
11 years | −11.9:−11.2 | −11.2:−10.8 | −10.0:−10.3 | −9.9:−10.5 | −5.6:−6.2 | −6.0:−5.9 | −3.1:−3.4 | −7.2:−7.8 | ||||||||
USA, n = 7119 | 7 years | Pure tone | Modified Hughson-Westlake method | Without reported hearing trouble | Average of better ear | 8.0 | 7.9 | 4.7 | 1.5 | 5.5 | 6.5 | 10.1 | 8.9 | Roberts and Federico (1972) | ||
10 years | 5.9 | 5.1 | 2.6 | 0.7 | 4.3 | 5.3 | 8.9 | 7.6 | ||||||||
USA, n = 6768 | 12 years | Pure tones, ASA 1951/ANSI-1 969 | Modif. Hughson-Westlake method, 40 locations in the USA | Probability sample of the non-institutionalised youths 12–17 years in the USA | Mean threshold of better ear vs. audiometric 0 ANSI 1969 | 9.2 | 6.4 | 2.0 | 1.1 | 5.2 | 8.0 | 10.2 | 7.0 | Roberts and Ahuja (1975) | ||
13 years | 9.4 | 6.2 | 1.8 | 1.1 | 5.6 | 8.4 | 11.0 | 7.2 | ||||||||
14 years | 8.8 | 5.8 | 1.6 | 1.1 | 5.2 | 7.7 | 10.9 | 6.5 | ||||||||
15 years | 9.0 | 6.0 | 1.5 | 1.2 | 5.6 | 8.0 | 11.4 | 6.8 | ||||||||
16 years | 9.0 | 5.5 | 1.4 | 1.0 | 5.2 | 8.0 | 12.2 | 6.2 | ||||||||
17 years | 9.4 | 5.4 | 1.2 | 1.0 | 5.0 | 8.0 | 11.4 | 6.4 | ||||||||
Taiwan, n = 1411 | 7 years | Pure tone, ANSI S3.1-1991 std. | Normal otoscopy and tympanometry | Mean threshold AC, male:female | 15.7:14.7 | 13.2:11.6 | 11.5:10.2 | 10.4:9.7 | Yang et al. (2011) | |||||||
10 years | 13.8:13.1 | 12.4:10.6 | 11.4:10.4 | 9.5:8.7 | ||||||||||||
14 years | 14.2:12.4 | 12.4:9.5 | 11.5:9.2 | 10.6:7.6 | ||||||||||||
16 years | 14.2:12.6 | 12.7:10.1 | 11.4:9.1 | 10.5:7.4 |
14.3 Normal Development Stages of Hearing and Auditory Processing
Development stage | Age | Developmental steps |
---|---|---|
Foetal stage | Conceptional age 26 weeks to birth | Hearing of maternal sounds and speech as well as damped external sounds and speech via bone conduction |
Neonatal stage | First days after birth | Loss of amniotic fluid from the middle ear; hearing of external sounds and speech switches from bone conduction to air conduction |
Postnatal stage | First year | Lowering of reaction thresholds and development of sound localisation (see Table 14.4); acceleration of auditory brainstem responses (auditory maturation (see Table 14.5)); use of hearing to control crying, babbling and first words |
Infantile stage | 1 to ~16 years | Development of auditory processing abilities; speech and language development |
It is interesting that hearing begins long before birth when the hair cells are fully developed and connected to the central nervous system. Normally, this is the case at a gestational age of around 19 weeks (Hepper and Shahidullah 1994). From that time on, the foetus uses hearing intensively, so far as we can judge by reports from pregnant women experiencing foetal movements in reaction to loud sounds, music and shouting.
These sounds are transmitted to the foetal cochleae via bone conduction. Testing of prenatal behavioural bone conduction seems to be feasible in utero and might be a promising method for the detection of hearing impairment—once a standard testing procedure and normative values for such a prenatal ‘diagnostic test’ have been made available. Other interesting observations are foetal auditory-evoked potentials (Cook et al. 1987). In animal studies with lambs, these have been detected at a gestational age of 117 days (from a total 144 days until birth). Human auditory-evoked potentials can be detected immediately before birth (Staley et al. 1990; Woods and Plessinger 1985) by the use ECG monitoring electrodes and clicks delivered by a loudspeaker, which has been used as an alternative to the conventional newborn hearing screening. In fact, much of the auditory abilities measured in newborns and infants are present long before birth. But this is true only for healthy children without congenital hearing loss and genetic hearing disorders. In contrast, children with genetic hearing loss have never been able to hear until they have been fitted with hearing aids or cochlear implants.
Age | Behavioural threshold (dB) as deviation from behavioural thresholds in adults (nHL) | Sound localisation |
---|---|---|
Newborn | 80 | No localisation but arousal from sleep |
3 months | 60 | Rudimentary head turn in the horizontal plane |
6 months | 50 | Slow localisation to side |
9 months | 45 | Slow localisation to side and below |
12 months | 40 | Slow localisation to side, below and above |
18 months | 30 | Rapid localisation to side, below and above |
2 years | 20 | Rapid localisation in all directions |
4 years | 10 | Rapid localisation in all directions |
6 years | 5 | Rapid localisation in all directions |
With air conduction, owing to experience from the foetal stage, the newborn is able to recognise a presented female voice as belonging to his or her mother (DeCasper and Fifer 1980).
In the postnatal stage, infants develop reactions to sounds of lower intensities and begin to localise sounds by turning their heads to the sound source (Table 14.4) (Northern and Downs 2014). Sound localisation requires two hearing ears and binaural interaction in the auditory pathway of the central nervous system.
Conceptional age (weeks) | Deviation from the latency of adults (ms) | Deviation from the threshold of adults (dB nHL) |
---|---|---|
25 | +4 | |
30 | +2 | |
35 | +1.5 | |
40 | +1 | +20 |
50 | +0.75 | +10 |
100 | +0.5 | +0 |
200 | +0.25 | |
300 | +0.1 |
For instance, if a 3-month-old infant born at term presents a behavioural threshold around 90 dB (Table 14.4) and a threshold in the auditory brainstem response of around 40 dB (Table 14.5), then the estimated ‘true’ threshold is around 30 dB. Assuming a conceptional age of 50 weeks for this example, the calculation is as follows: 90 – 60 = 30 (Table 14.4) and 40 – 10 = 30 (Table 14.5).
In the postnatal stage, infants develop abilities that allow for auditory feedback in the control of vocalisations and speech-sound production. It has been shown that at the age of 4 months, infants are able to imitate the vowels /a/, /i/ and /u/ selectively (Kuhl and Meltzoff 1996); at the age of 4 to 9 months, normal-hearing infants are able to control prosodic patterns of crying by using auditory feedback, while infants with so far unaided profound hearing loss are not (Möller and Schönweiler 1999). These abilities cannot be perceived by untrained listeners but can be discovered by acoustic analyses and linguistic transcripts (Kuhl and Meltzoff 1996; Möller and Schönweiler 1999). Finally, at the age of 8–10 months, untrained listeners can perceive reduplicated babbling in infants with normal hearing. In children with unaided profound hearing loss, reduplicated babbling cannot be observed (Northern and Downs 2014).
In the infantile stage, children develop abilities of auditory processing and auditory perception that allow for speech understanding in noisy environments, speech-sound discrimination, dichotic listening, auditory memory and phonological awareness. For many of these abilities, a number of test procedures have been developed that permit comparison with age-dependent normative data. If a child presents typical symptoms of auditory processing disorders (APD), the diagnosis can be confirmed from age 7 on. These issues will be discussed in the sections on auditory processing disorders (Sects. 15.2 and 16.8).
14.4 Normal Development of Behavioural Responses to Sound in Children
In a study by Hepper and Shahidullah (1994), one foetus had been observed to move in response to sound as early as at 19 weeks of gestational age, and babies are born with basically functioning hearing. In those incredible early months after birth, babies grow and learn, demonstrating behavioural responses to sound that change as they mature.
Different types of behavioural responses to sound that develop in normal children
Approximate age (months) | Behavioural responses to sound (Northern and Downs 2014; American Speech-Language-Hearing Association 2005; Lewis et al. 2006; First Years 2011; Center for Early Intervention on Deafness 2016; Centers for Disease Control and Prevention 2016) |
---|---|
0–2 | Arousal from sleep; eye widening; change in sucking patterns during feeding; limbs move or slow down; change in breathing pattern; quietens, cries, startles to sudden loud sounds; may be soothed by certain songs or music |
2–4 | Eye widening or searching; stilling or quietens to sounds; crying/smiling or other change in facial expression; limb extension; rudimentary head turn; turn taking cooing; vocalises to get attention |
4–6 | Localisation of sound to side; looks puzzled when hears something new or unexpected; turns quickly to mother’s voice across room; begins to imitate vocalisations of adults |
6–9 | Localisation of sound to side and directly below; able to make a full head turn to the sound; increasingly interested in its own voice and sounds; sways/bounces to music; begins to copy rhythm and actions of rhymes/songs; begins to make bisyllabic babble, practising the sequence of the same sounds |
9–12 | Localises to side and below; answers to/recognises its own name; responds to keywords in play; understands the meaning associated with some environmental sounds (e.g. telephone ringing) |
12–15 | Localises to side, below and immediately above; immediately turns to its own name; points to pictures in a book when item named; finds an object when asked to |
15–18 | Localises to side, below and above; follows simple spoken commands; begins to fill in a familiar missing word when an adult leaves a pause in rhymes and story books; increasing quality of prosody in vocalisations; increasing ‘word-like’ babble; emergence of single words |
18–24 | Recognises and names environmental sounds; understands when called from another room; follows a conversation on a known topic |
24–36 | Understands most of a direct conversation; enjoys listening to longer stories; answers questions about familiar topics |
36–48 | Hears television at same level of loudness as others; listens closely and retells stories; accurately repeats predictable sentences |
48–60 | Understands most of what is said in school and at home; can follow more complex instructions; can identify rhyming or non-rhyming words from small sets |
There is a great variability as to when different babies reach different milestones.
Babies do not consistently respond to sound until they are around 7–9 months of age when they sit up and turn their head; one cannot rely solely upon the observation of development of behavioural hearing responses to exclude deafness.
Very young babies do not respond to the quietest sounds that they can hear but only to supra-threshold sounds that they can hear (Williamson 2002).
In most babies born with significant hearing loss, this impairment is invisible. In a baby with a syndrome (e.g. Down syndrome), professionals are more easily alerted to the possibility of hearing loss.
14.5 Speech and Language Development of Normal-Hearing and Hearing-Impaired Children
14.5.1 Language Acquisition
Language acquisition is the most complex task humans have to accomplish in their early development. Hearing, cognitive, motor as well as social development must work hand in hand to promote this process. However, no explicit instruction is necessary to achieve this. During the sensitive period for language acquisition, adequate language input of the environment is sufficient. Discrimination of phonemic contrasts for all language is an inborn ability, and the base for the production of vowel-like sounds typically starts at 3 months of age. During the first year of life, foreign language-specific perception abilities decline as native language perception is enhanced. Speech production starts with a universal phase in which the production of non-speech sounds is followed by vowel-like sounds and canonical babbling. Around 10 months of age, language-specific speech production starts, and first words are expected at age 1 year. Generally speaking, children have acquired the full phonemic repertoire of their specific language, as well as most grammatical structures and a wide range of vocabulary, from the ages 4 to 5.
There is a slight variation in the acquisition of sounds depending on the language of a child. However, generally the phonemes first acquired are bilabial sounds, followed by first and second articulation zone sounds and succeeded around 2.5 years by the third articulation zone sounds. By 3 years the first complicated consonant combinations are present. At age 4 all phonemes of the mother tongue have been learned, though difficult consonant combinations and sibilant sounds might still be imperfect. In a normally developing child, perfection is achieved by age 6 (O’Hare and Bremner 2015).
The first words are nouns that are connected with the environment of the child and therefore depend on where and how the child lives. By age 2 around 50 words are known and the first verbs and adjectives appear. In the period from 2 to 4 years, active and passive vocabulary multiplies, and when school starts, the vocabulary should be from 2000 to 3000 active words. Acquisition of vocabulary never stops and adult vocabulary consists of 20,000–250,000 words (O’Hare and Bremner 2015).
One-word utterances around the age 1 consist of nouns. At age 2 most children use two- to three-word utterances and ask first questions by changing intonation. Sentences are grammatically still incorrect. Children start to use interrogatives at approximately age 3, and short sentences are grammatically accurate, subordinate clauses appear. Around age 4 sentences get longer and grammatical constructions more complex. Grammar is usually correct at age 6, and children are able to express their thoughts in different time forms as well as re-narrate events and stories (O’Hare and Bremner 2015).
14.5.2 Sign Language
If an auditory-oral approach is not possible, or signed language may be helpful as an additional communication mode, or the child is born to deaf parents and sign language is preferred, characteristics of signed language have to be considered. Signed languages such as British Sign Language (BSL) or German Sign Language (DGS) are natural languages with the same structures as spoken languages, and learning these is a task as complex as learning spoken languages. Caregivers adapt their sign language to the need of the child as is done for spoken language, and the child develops speech the same way as children do in spoken language, starting with ‘sign babble’, simple signs and one-word utterances (Morgan 2014). As for spoken language, competent role models are needed to facilitate normal sign language development. As less than 5% of prelingually deaf children have signing deaf parents, early acquisition of sign language for a child in a hearing family has to be supported by bringing a natural signing adult into the child’s environment.
14.5.3 Conductive Hearing Loss
Conductive hearing loss is the most prevalent type of hearing loss in childhood, commonly due to otitis media with effusion. Although this condition is the most frequent, little consistency is found regarding its long-term consequence on speech and hearing. This is partly because there are hardly any studies available covering the most sensitive period of these developmental processes, which lies between birth and age 2. Furthermore, most reports are retrospective, and the chosen methodology and the age at test may not be suitable for the detection of the consequences of early disease (see Zumach et al. 2009 for discussion).
Studies have shown a greater signal-to-noise ratio in children of age 7 (Zumach et al. 2009) than 4 (Gravel and Wallace 1992). This is a relevant finding, as hearing in noise is the normal condition in everyday life. In the Zumach study, correlations were not found between socio-economic status and cognitive development but with the frequency and level of hearing loss due to middle ear effusion.
Another study has shown that when long lasting, otitis media with effusion is prone to lead to auditory deprivation and can result in deterioration of listening and language performance as well as central auditory processing deficits (Klausen et al. 2007).
On basis of auditory event-related potentials, Haapala et al. (2015) studied neural mechanisms of involuntary attention in 22–26-month-old children with repetitive middle ear infections and healthy controls. The analysis of auditory P3a showed an atypical neural organisation, the late negativity LN a longer latency in children with recurrent middle ear problems suggesting long-term effects on the developing central auditory system.
In conclusion, although a direct correlation between otitis media and long-term effects on speech and language development cannot be proven at present, children having problems in language acquisition need to be considered at risk of further deterioration of their condition by an additional conductive hearing loss due to effusion. Therefore, they should be treated straight away, not only taking conservative but also surgical options into consideration.
14.5.4 Speech and Language Development in Subgroups of Sensorineural Hearing Loss
Bilateral Sensorineural Hearing Loss
If pan-cochlear bilateral sensorineural hearing loss exceeds 40 dB, deficits in speech perception have to be expected. The individual extent of deviation from the norm varies a lot, ranging from a simple delay to a complete inability to acquire spoken language. Generally speaking, a higher degree of hearing loss leads to more abnormalities. Deficits can be seen in all linguistic fields.
Articulation of vowels and consonants may be problematic with substitutions, distortions and omissions. For affected children it may be difficult to differentiate between different vowels and voiced and voiceless consonant sounds. Phonological development is considerably slowed (Dobie and Van Hemel 2004); speech intelligibility is reduced.
Vocabulary acquisition is slowed and might plateau early. Nonliteral and abstract words are especially difficult to integrate in the lexicon (Briscoe et al. 2001; Jerger et al. 2002). Grammatical knowledge is impaired with sentences being shorter, of more simple structure, and passive voice is rarely seen (Dobie and Van Hemel 2004). As language proficiency is a prerequisite for reading achievement, literacy skills are often impaired in school age children with hearing loss (Carney and Moeller 1998).
This statement is still true with today’s hearing aid technology and timely rehabilitation (Eisenberg 2007; Tomblin et al. 2015).
Bilateral High-Frequency Sensorineural Hearing Loss
Bilateral high-frequency hearing loss is known to cause problems with phoneme acquisition for fricatives. It is important to remember that high-frequency hearing loss cannot be detected by the measurement of TEOAE (transient evoked otoacoustic emissions), which is the most common method applied for newborn hearing screening in Europe. So children with deficits in the pronunciations of these sounds have to be tested differently, since the deficits might have been missed in early testing. Behavioural testing, DPOAE (distortion product evoked OAE) and frequency-specific evoked response audiometry have to be applied.
Mid-frequency Sensorineural Hearing Loss
Mid-frequency hearing loss up to a maximum of 50 dB may not cause significant symptoms during early stages of language acquisition. However, those children, if not detected by newborn hearing screening or preschool hearing test, often are presented at school age with listening problems in the classroom environment.
Low-Frequency Sensorineural Hearing Loss
There is no literature on isolated low-frequency hearing loss and speech development. The low frequency loss seldom exceeds 50 dB and is a relatively rare condition. As these children are usually only presented for doctor’s advice at school age, one can assume that their speech development is not grossly disabled. Late diagnosis is possible as for cases with unilateral or mild hearing impairment (Feder et al. 2017).
Unilateral and Mild Sensorineural Hearing Loss
Mild and unilateral hearing loss primarily result in difficulties understanding speech in noisy situations and impaired localisation abilities (Bess et al. 1986, 1998).
About 30% of children with mild or unilateral hearing loss are found to have delayed speech development if not supplied with an amplifying hearing device (Yoshinaga-Itano et al. 2009). Difficulties arise in the fields of use of language, vocabulary, articulation and phonological discrimination (Tharpe 2008). Lower performance in reading and spelling is possible (Winiger et al. 2016). Nevertheless this kind of hearing loss may be unnoticed and late-diagnosed (Feder et al. 2017).
Auditory Processing Disorder
The influence of auditory processing disorders on speech development is discussed controversially, and there is limited literature on direct correlations between particular deficits in auditory processing and specific features of speech development. This is partly because there is little knowledge about norms in many fields of auditory perception and little agreement on what auditory processing really is. However, children with delay in speech development often have pathological scores in auditory memory tests. A defined subset of dyslexic children has difficulties with the discrimination of phonemes. Though, the most common reason for dyslexia is a deficient phoneme grapheme assignment (McArthur et al. 2013).
Auditory Dyssynchrony
The group of children with auditory dyssynchrony is very heterogeneous with many subjects suffering from additional neurological and intellectual handicaps that impact on speech development. Children suffering from this condition, but who are cognitively within the normal range, show variable outcomes in respect to speech development, ranging from near normal to severely retarded or disturbed (Uhler et al. 2012; Nikolopoulos 2014).
14.5.5 Outcomes After Interventions for Sensorineural Hearing Loss
In general, it is agreed that intervention for sensorineural hearing loss should be as early as possible. In the age of universal newborn hearing screening, the goal therefore is that children are diagnosed and get their hearing aids by the age of 6 months. For all other children, intervention should start immediately after diagnosis of hearing loss (Silverman 1983).
Hearing Aids
Little prospective, controlled design research has been undertaken to study formally the impact of hearing aids and hearing aid fitting on the hearing and speech development of children with hearing impairment. Clinical experience shows that acquisition of language is facilitated by hearing aids. However, there are vast differences in the progress between children who suffer from a similar hearing loss. Apart from the degree of hearing loss, the following contributing factors have been assessed retrospectively to reveal a positive impact on speech development: early use of hearing aids, highly educated mothers, reliance on spoken language, integration and structured teaching individually (Yoshinaga-Itano et al. 1998; Tomblin et al. 2015).
Cochlear Implantation
For the profoundly hearing-impaired, cochlear implantation is a valuable way to gain more auditory access to speech. Owing to the success of this rehabilitation method, inclusion criteria for cochlear implantation have been broadened towards more residual hearing.
Today if young children receive cochlear implants of high technological standard, acceleration of speech development is observed. However, Nittrouer showed that for implant recipients who received their first implants at an average age of 20 months, at age 80 months, speech measures (utterance length in morphemes, number of conjunctions, personal pronouns, bound morphemes, different words) were at least one standard deviation or more below those of the normal-hearing group (Nittrouer et al. 2014). We expect that bilateral implantation at an even earlier age will bring additional improvements. According to Levine et al. (2016), it is crucial to experience a language model in the first year of life. But still further studies are needed concerning the acquisition of complex language, vocabulary and literacy skills for the population of children who were implanted early and profited from newer technologies.
Intelligibility of speech, which in the hearing aid area had always been a major concern for the population with profound hearing loss, has improved dramatically through cochlear implantation, both in quality and in the time needed to achieve it (see review Flipsen 2008).
Factors with an impact on speech development in early implanted children are new coding strategies, implantation at younger age, more residual hearing preimplantation, (re)habilitation emphasising auditory perception and speech development (Geers 2006).
14.6 Psychomotor and Cognitive Stages of Normal Children of Different Ages
See Part III Sect. 9.2.
14.7 Aetiology of Hearing Disorders and the Likelihood of Involvement of Other Systems
14.7.1 Risk Factors for Permanent Childhood Hearing Impairment
Thirty to 50% of cases of hearing loss are of unknown aetiology (Fortnum and Davis 1997; Parving 1984; Davis and Wood 1992; Newton 1985). Where the aetiology is known, at least half are genetic (Smith et al. 1993), and approximately 30% of this group is syndromal (Davis et al. 2004). Fortnum and Davis state that 10% of hearing loss cases in their study had pre- or perinatal aetiologies and 6% had postnatal aetiologies. Postnatally acquired and late-onset cases account for 7.5–25% of permanent childhood hearing impairment (Fortnum 2003; Weichbold et al. 2006). By far the most common childhood hearing loss is the typically temporary, conductive hearing loss caused by Otitis Media (OM) which, in its various forms, has a prevalence of 1.41 per 1000 in high-income North America and as high a prevalence as 97 per 1000 in South Asia (with hearing loss defined as auditory thresholds >25 dB HL in the better ear) (Monasta et al. 2012).
Caregiver concern§ regarding hearing, speech, language or developmental delay.
Family history§ of permanent childhood hearing loss.
Neonatal intensive care of more than 5 days or any of the following regardless of length of stay: ECMO§, assisted ventilation, exposure to ototoxic medications (gentamicin and tobramycin) or loop diuretics (furosemide) and hyperbilirubinemia that requires exchange transfusion.
In utero infections, such as CMV§, herpes, rubella, syphilis and toxoplasmosis.
Craniofacial anomalies, including those that involve the pinna, ear canal, ear tags, ear pits and temporal bone anomalies.
Physical findings, such as white forelock, that are associated with a syndrome known to include a sensorineural or permanent conductive hearing loss.
Syndromes associated with hearing loss or progressive or late-onset hearing loss§, such as neurofibromatosis, osteopetrosis and Usher syndrome; other frequently identified syndromes include Waardenburg, Alport, Pendred and Jervell and Lange-Nielsen.
Neurodegenerative disorders§, such as Hunter syndrome, or sensory motor neuropathies, such as Friedreich ataxia and Charcot-Marie-Tooth syndrome.
Culture-positive postnatal infections associated with sensorineural hearing loss, § including confirmed bacterial and viral (especially herpes viruses and varicella) meningitis.
Head trauma, especially basal skull/temporal bone fracture§ that requires hospitalisation.
Chemotherapy§.
Risk indicators that are marked with a “§” are of greater concern for delayed-onset hearing loss.
The US Preventive Services Task Force (2008) lists the following risk factors: NICU admission for ≥2 days, family history of hereditary childhood sensorineural hearing loss, craniofacial abnormalities, certain congenital syndromes (such as Usher or Waardenburg) and infections (e.g. toxoplasmosis, bacterial meningitis, syphilis, rubella, Cytomegalovirus, Herpes virus).
A retrospective study conducted by Connolly et al. (2005) found that 1 of 75 infants with such risk factors had a hearing loss, but only 1 of 811 infants without risk factors did. The National Institutes of Health (NIH) (1993) reported that of all babies born with hearing loss each year, approximately 50% were discharged from the well-baby clinic with no known risk factors for hearing loss. Universal newborn hearing screening programmes, which are already implemented in many countries, are therefore of crucial importance.
Detailed information on the aetiology of childhood hearing loss will be given in the Sect. 14.8 Infection with Neurotropic Viruses, Sect. 14.9 Ototoxicity in Children, Sect. 14.10 Genetics of Hearing Loss and Sect. 14.11 Syndromes Associated with Hearing Impairment. Aetiological aspects of special kinds of hearing loss are presented in the Sect. 15.1 Types and Time Courses of Hearing Loss, Sect. 15.2 Auditory Processing Disorders, Sect. 15.3 Hyperacusis, Sect. 15.4 Tinnitus and Sect. 15.5 Nonorganic (Functional) Hearing Loss.
14.7.2 Additional Disabilities
Prevalence data concerning additional disabilities vary in the literature from approximately 20–40% of children born with hearing loss. For example, the 2007–2008 Annual Survey of Deaf and Hard of Hearing Children in the USA shows 39.3% of children with educationally relevant conditions; the study of Cupples et al. (2014) reported 26.4% of hearing-impaired children with additional handicaps. In spite of such differences—possibly due to different methodological approaches and demographical distinctions—mental retardation and learning disability are more frequent than visual impairment, cerebral palsy or autism spectrum disorder (Cupples et al. 2014) (for further details, see Sect. 16.23).
To identify the cause of hearing loss and to detect possible coexisting medical conditions, the following overview presents a pathway for medical investigations offering the chance to avoid progression of hearing impairment and to prevent complications. Further details can be found in Sect. 16.20.
14.7.3 Medical Investigations for Clarification of Aetiology
Since the introduction of the newborn hearing screening programme (NHSP), permanent childhood hearing impairment (PCHI) is being identified at an earlier age. Parents and families are being provided with a wealth of medical information and intervention within days of having a new baby. Investigating hearing loss in a timely manner has a direct impact on understanding the cause of hearing loss in the child and importantly on the acceptance of the diagnosis by the family, although it is recognised that sometimes it may affect bonding between child and a parent. Medical investigations must be initiated by a professional with training and understanding of the complexities associated with a diagnosis of hearing loss.
Historically, congenital PCHI has been classified as either hereditary or acquired. Investigations aim to identify the cause of hearing loss, identify coexisting medical conditions (e.g. renal pathology in Branchio-Oto-Renal syndrome) and prevent progression in hearing loss in those children identified with widened vestibular aqueduct (WVA) or congenital cytomegalovirus infection (CMV). In addition, investigations may help us understand genetic hearing loss and secure genetic counselling. Investigations may help identify and prevent complications in some children with profound sensorineural hearing loss (SNHL) and cardiac conduction defects (Jervell and Lange-Nielsen syndrome). Children identified with mitochondrial m.1555A > G mutation will be given advice regarding development of progressive hearing loss associated with aminoglycoside medications, thereby preventing further deterioration in hearing by avoiding exposure to those medicines.
The objectives of performing investigations are therefore to provide the best clinical and audiological outcomes in a child with PCHI. A robust and systematic pathway for investigations by a suitably trained professional is essential for the success of the process.
14.7.4 Timing of Investigations
Investigations should be offered as soon as possible so as to maximise the chance of arriving at a diagnosis (BAAP 2015a, b, c) with consideration given to parental readiness and the general health of the baby.
Investigations should be considered as an active process as new data emerge as the child grows; therefore a review of the diagnosis at each clinical encounter is extremely useful. One positive investigation does not automatically exclude other conditions, as varied aetiology providing diagnostic clues may be present in an individual child.
Some treatable viral infections that may improve clinical outcomes benefit from early identification. Other investigations can be delayed in a family where there are difficulties with acceptance of the diagnosis.
14.7.5 History
General History
The aetiology can be traced by careful history-taking and should include the details of pregnancy (possible maternal intrauterine infections in the first trimester, exposure to drugs and alcohol during pregnancy, ABO incompatibility, rhesus disease and maternal diabetes/hypothyroidism), birth and postnatal period.
Perinatal events including hypoxia, hyperbilirubinaemia, sepsis and the potential exposure to ototoxic medication and the need for mechanical ventilation should be asked for.
Postnatal history should cover history of meningitis (neonatal meningitis is a well-known risk factor for hearing loss), noise exposure including incubator noise, ototoxic medication/radiotherapy, history of falls and accidents leading to head injuries, viral illnesses of childhood such as mumps, measles and rubella and immunisation status (Bamiou et al. 2000) (see Sect. 16.1).
Family History
A detailed family history of hearing loss or risk factors associated with hearing loss in at least three generations should be recorded wherever possible (BAAP 2015a, b, c). Any consanguinity between parents must be noted. A pedigree chart can indicate modes of inheritance such as Mendelian dominant, recessive, X-linked or mitochondrial (see Sect. 16.1).
14.7.6 Clinical Examination
It is imperative to carry out a complete physical examination after undressing the child. Height, weight and head circumference should be measured and plotted on an appropriate growth chart recording centiles for comparison. Particular note must be made of the ocular region, ears, nose, midface and neck. Otoscopy and examination of the palate are essential. Further, examination of the skin for pigmentation, limbs and digits, spine, cardiovascular system and cranial nerves is important (BAAP 2015a, b, c) (see Sect. 16.1).
14.7.7 Family Audiograms
All first- and second- (if possible) degree relatives should have formal audiometric assessment whether or not they report hearing difficulties. It is recommended that each Paediatric Audiology Department has a robust policy for performing family audiograms with the necessary referrals should a hearing loss be identified (see Sect. 17.4).
14.7.8 Consultative Examinations
Ophthalmology
It is estimated that 40% of children with PCHI may in addition have an ophthalmological condition (Guy et al. 2003). Vision and the development of communication are closely linked (Laffan 1993). Children with deafness are more reliant on their vision for their communication as they may use Picture Exchange Communication System (PECS) and lip reading to aid their communication.
The Royal College of Ophthalmologists recommends targeted clinical surveillance of certain groups at high risk of eye disorders, including children with a sensorineural deafness. It recommends the inclusion of a full orthoptic examination, cycloplegic refraction and a fundus examination (NDCS and Sense 2014).
In some children with SNHL, the eye examination may help make the diagnosis such as CHARGE association, Usher syndrome or congenital CMV.
Electrocardiography (ECG)
All children with bilateral profound SNHL must be offered an ECG to examine the possibility of a long QT interval. Jervell Lange-Nielsen Syndrome (JLNS) is an extremely rare condition with a long QT interval and profound SNHL but associated risk of sudden death, preventable if identified early. An immediate referral to a Paediatric Cardiologist is recommended.
Genetic Investigation
Examinations for connexin 26 and 30 mutations, mitochondrial mutation m.1555A > G where there is history of hearing loss through maternal inheritance or hearing loss following application of the aminoglycosides, Pendrin gene testing in children with bilateral widened vestibular aqueduct (WVA) and Mondini anomaly (Bogazzi et al. 2004) are currently recommended.
Families can also be referred to a geneticist if there are dysmorphic features, developmental delay, multiple unexplained pathologies or on parental request. The geneticist may be able to suggest additional investigations that are not routinely available. Parents must be given a thorough explanation of the advantages and disadvantages of those tests and must be assisted in making an informed decision regarding whether or not they would like to have their child investigated (see Sect. 16.22).
14.7.9 Serological Investigations
Testing for Congenital Infections
Congenital cytomegalovirus (cCMV) accounts for 20% of all childhood sensorineural hearing loss (SNHL) but is not routinely tested for at birth. Valganciclovir has been shown to prevent hearing deterioration and improve neurocognitive outcomes if started in the first month of life (Kadambari et al. 2015a).
In babies less than 1 year old, urinary or salivary CMV DNA PCR on two separate occasions should be performed. In babies less than 3 weeks of age, a positive test confirms congenital CMV. In children greater than 1 year of age, CMV IgG antibody testing with or without urinary CMV DNA PCR should be performed. In both groups a positive result must be confirmed with neonatal dried blood spot testing for CMV DNA.
At any age maternal CMV IgG antibody testing can be performed; a negative result rules out congenital CMV.
Serology for rubella, toxoplasma, syphilis and HIV can also be carried out where clinically indicated.
Thyroid Function Tests
These should be carried out in families with history of thyroid disease or goitre. They should be considered in children with WVA or Mondini deformity; as the thyroid dysfunction in Pendred syndrome tends to occur in late childhood, testing should be timed accordingly.
Urine Examination
Dipstick studies for the presence of blood and protein should be carried out in order to exclude Alport syndrome.
Autoimmune Tests
These should be reserved for children with progressive hearing loss or where there is evidence of systemic involvement. Tests may include antinuclear antibodies, antineutrophil cytoplasmic antibodies, dsDNA, RA factor, antiphospholipid, anticardiolipin, antithyroid antibody, antibodies to Sm, ESR, CRP and others as indicated (BAAP 2015a, b, c).
14.7.10 Imaging
Magnetic resonance imaging (MRI) of the inner ears and internal auditory meatuses (IAMs) is the first-line radiological investigation in children (BAAP 2015a, b, c). This investigation will show structural anomalies of the ear (soft tissue) apart from details of the ossicular chain. The computed tomography (CT) scan of the petrous temporal bone in children with permanent conductive hearing loss and craniofacial anomalies will show the bony structures (see Sect. 16.21).
14.7.11 Vestibular Investigations
These investigations should be considered when motor milestones are delayed or there are reported problems with balance or complaints of dizziness. Children presenting with imbalance, motor developmental delay and hearing impairment of various degrees should be suspected of having vestibular dysfunction. This discovery will help the establishment of the diagnosis in a child presenting with hearing impairment but also will allow for treatment and possible avoidance of life-threatening circumstances in the future.
Reported prevalence of vestibular disorders in the deaf population of children is 30–40% (Moller 2002) (see Sect. 16.15).
At times, despite thorough medical investigations, no cause of hearing impairment can be established. These children require periodic review of their investigations, concurrently with reassessment of their medical and audiological status.
14.8 Infection with Neurotropic Viruses
14.8.1 Definition and Classification
Neurotropic viruses have a predilection for nervous tissue and may be neuroinvasive (entering or infecting tissue) and neurovirulent (causing disease). Viruses may enter the nervous system via blood when infected immune cells carry the virus to nervous tissue or when blood capillaries are crossed by free viruses or in leukocytes (Nath and Berger 2003). Viruses can also travel along the axons of nerves, thus escaping the immune system (Wright et al. 2008). Once viruses are inside neurons, they can survive for the host’s lifetime and cause damage to the nervous system (Nath and Berger 2003).
Acute infection possible by: Japanese encephalitis, Venezuelan equine encephalitis and California encephalitis viruses, tick-borne encephalitis virus (TBEV), polio, coxsackie, echo, mumps, measles, influenza, rabies viruses, members of the family Herpesviridae such as herpes simplex, varicella-zoster, cytomegalo, HHV-6 and Epstein-Barr viruses
Latent infection possible by: herpes simplex and varicella-zoster viruses
Slow virus infection possible by: measles, rubella and John Cunningham (JC) viruses, retroviruses such as human T-lymphotropic virus 1 and human immunodeficiency virus
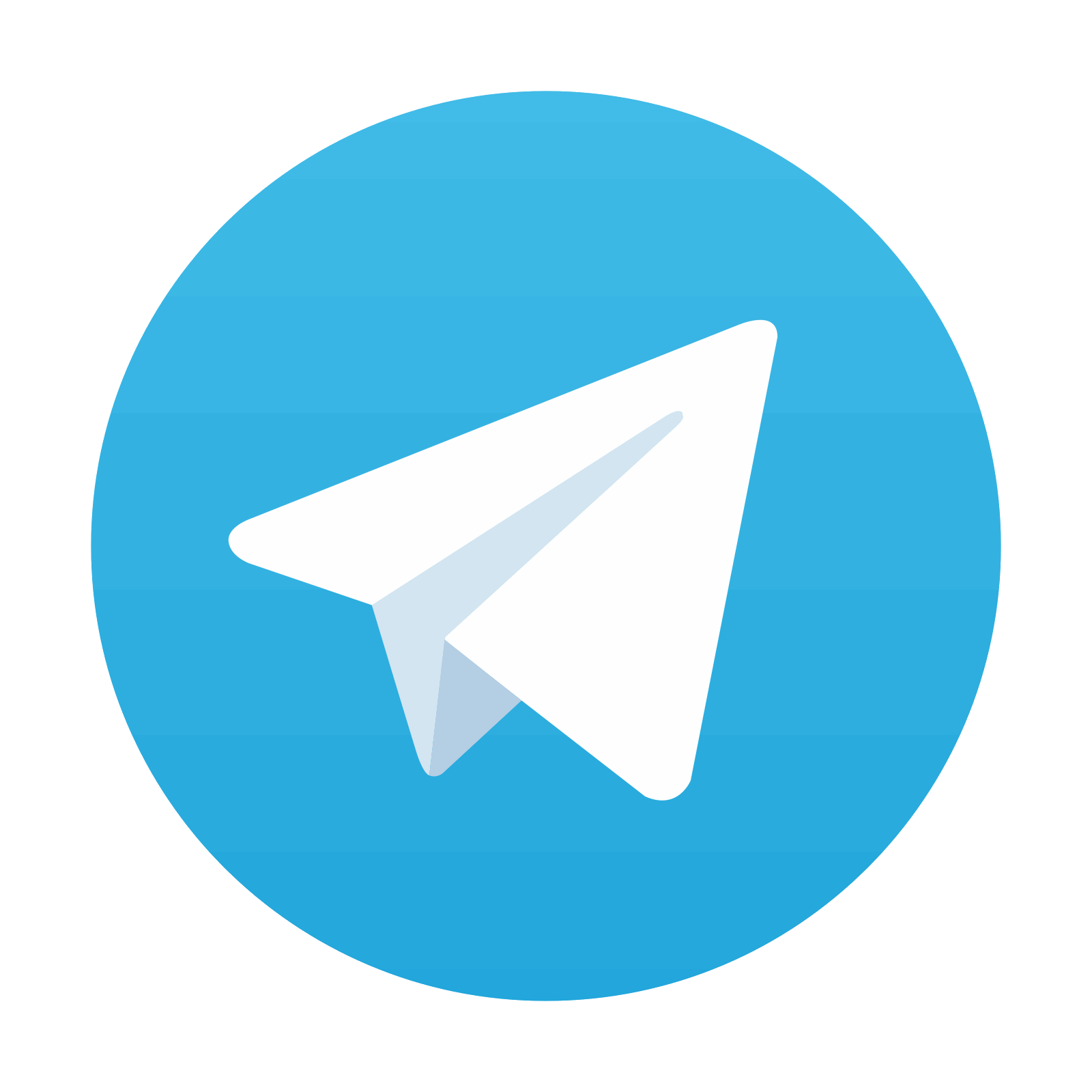
Stay updated, free articles. Join our Telegram channel

Full access? Get Clinical Tree
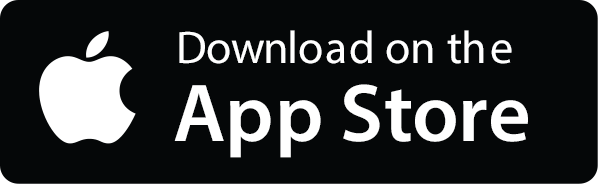
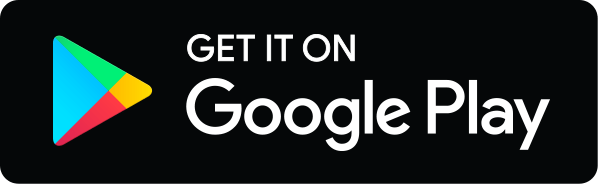