Mendelian modes of inheritance observed in hearing loss. (a) Autosomal recessive; (b) autosomal dominant; (c) X-linked inheritance. Circles, female individuals; squares, male individuals. Horizontal connections indicate partnerships. Symbols of children are horizontally arranged below the parents. Solid symbols, affected individuals. Open symbols, unaffected individuals. Grey bars below individuals in A and B represent the two copies of autosomal deafness genes. ‘X’ and ‘Y’ in C stand for the sex chromosomes. Red dots and red X indicate presence of deafness-causing mutation
Approximately 20% of patients have autosomal dominant non-syndromic hearing loss (ADNSHL), which is typically post-lingual and progressive. In ADNSHL, hearing loss already occurs when only one allele carries the mutation (Fig. 3.1b). The recurrence risk for offspring of affected persons is 50%.
X-linked hearing loss (XNSHL) is caused by mutations on the X chromosome and therefore primarily affects males; heterozygous (female) carriers usually have normal hearing. XNSHL is very rare (<1%) (Fig. 3.1c).
Moreover, hearing loss may—very rarely—result from mutations of mitochondrial DNA. Because mitochondria are exclusively inherited maternally, transmission occurs only through affected females whose children will all be affected.
3.1.3 Molecular Genetic Testing
Conventional DNA Sequencing (Sanger Sequencing)
Because of the extensive genetic heterogeneity of hearing impairment, the identification of the causative mutation in patients has been the exception until recently. The exons are the protein-coding parts of genes and harbour more than 80% of disease-causing mutations, and they therefore represent the regions of primary diagnostic interest. Because they are interrupted by the non-coding introns, genetic testing has so far required the laborious individual amplification and conventional sequencing (‘Sanger sequencing’) of all exons of a gene of interest. For all currently known deafness genes, this amounts to more than 1500 exons, and comprehensive genetic analysis has therefore not been possible so far.
3.1.4 High-Throughput Sequencing (Next-Generation Sequencing): A Breakthrough in Diagnostics
Bypassing the Bottleneck of Conventional Sequencing

Workflow scheme of next-generation sequencing (NGS)
Dramatically Increased Diagnostic Yield
NGS has begun to transform not only the field of medical genetics but medicine as a whole. In our experience, targeted NGS of all known deafness genes, including those for the clinically most important and prevalent syndromes, identifies the molecular basis of the sensory deficit in more than 60% of the patients (in both autosomal dominant and autosomal recessive forms).
With NGS increasingly being applied for genetic diagnostics of hearing loss, the true distribution of mutations across the genes is now becoming known. This in turn helps in prioritising genes when carrying out genetic testing via conventional (Sanger) sequencing—which is important because not every laboratory can afford the expensive NGS infrastructure and because healthcare providers do not yet generally reimburse NGS-based testing.
Quantification of NGS Data Uncovers Hidden Structural Mutations

A Schematic representation of the mapped sequencing reads (reverse strand) visualised with the Integrative Genomics Viewer (IGV) for a patient with non-syndromic deafness due to a homozygous nonsense mutation (p.Trp77*) of the GJB2 gene. The c.231G > A mutation (C > T on the reverse strand displayed here) is present in virtually all reads covering this region of the gene

Quantitative readout of NGS data. Unlike Sanger sequencing, NGS is capable of unmasking large structural mutations such as deletions of one or several exons. Every cell contains two copies of every autosomal gene, a maternal and a paternal copy. Boxes represent the exons that undergo targeted enrichment. The coverage, consisting of sequenced reads that redundantly cover every exonic position, is shown below. In this example, the red exon is deleted on the maternal gene copy. As a consequence, only about half of the normal read number will be generated for the red exon, compared with the respective exon in control samples and to the neighbouring exons. The bioinformatic pipeline can reliably detect the ~50% reduction of NGS reads in such patients
3.1.5 The Relevance of an Early Genetic Diagnosis in Hearing Loss: The Role of NGS
Although the identification of the genetic basis of the hearing deficit is still the exception in most patients, it is crucial, especially in newborns and infants, to provide effective medical care for several reasons.
Identification of Syndromes Before Manifestation of Extra-cochlear Symptoms
Without a genetic diagnosis, children with hearing loss of then unknown aetiology should, in their first decade of life, regularly be investigated by different specialists, at least including ophthalmologists (for Usher syndrome), cardiologists (for Jervell and Lange-Nielsen syndrome), nephrologists (for Alport syndrome) and endocrinologists (for Pendred syndrome). This results in significant economic costs for the healthcare system but also in a considerable psychological burden for the patients and their parents.
About 10% of children with apparently non-syndromic congenital or early-onset hearing loss have Usher syndrome and develop retinal degeneration at an older age (in the first decade in Usher syndrome type 1 or in the second decade in type 2). The identification of mutations in an Usher syndrome gene thus predicts severe visual impairment (potentially ending up in blindness) with currently no therapeutic option and therefore is important information for the parents when considering cochlear implantation versus hearing aids.
Another important example is Jervell and Lange-Nielsen syndrome (JLNS), a condition resulting from mutations in the genes KCNQ1 and KCNE1: JLNS patients initially appear to have non-syndromic hearing loss. Often, the first clinical manifestation of the accompanying arrhythmia is sudden cardiac death. This may affect several children in a family before it is realised that deafness in such families signals the risk of sudden death. Thus, the identification of KCNQ1/KCNE1 mutations by comprehensive NGS-based testing of deafness genes in newborns with hearing loss must result in follow-up by a cardiologist, which is potentially life-saving. Importantly, the overall prevalence of JLNS is low but higher in populations where consanguinity is common. In view of increasing migration from Middle East countries into Europe, paedaudiologists should be aware of this syndrome.
Patients with apparently recessive non-syndromic hearing loss may have mutations in SLC26A4, the gene associated with Pendred syndrome. These patients may develop (or already have) goitre with hypothyroidism, requiring levothyroxine treatment to ensure normal somatic and mental development. Moreover, the patients usually have enlarged vestibular aqueducts and endolymphatic sacs and anatomical abnormalities of the cochleae (Mondini dysplasia). They should therefore avoid activities that can lead to minor head trauma (e.g. contact sports, etc.).
Disentanglement of Complex Genetic Causes
Disease may be due to mutations in more than one gene, especially in offspring of consanguineous partnerships. For example, deaf-blindness is not always caused by mutations in one of the Usher syndrome genes. It may also reflect an overlap of non-syndromic deafness and non-syndromic retinal degeneration, resulting from independent mutations of a deafness gene (e.g. OTOA) on one hand and a retinal dystrophy gene (e.g. NR2E3) on the other—together mimicking a syndromic condition. Disentangling such constellations has a major impact on genetic counselling. In the given example, instead of an Usher syndrome recurrence risk of 25% for further offspring, there are separate recurrence risks: 25% for either deafness or retinal degeneration and 6.25% for the combination of both (presenting like Usher syndrome).
Identification of Potentially Treatable Conditions
Mutations in PEX genes cause peroxisome biogenesis disorders (PBD), including Refsum syndrome, a metabolic condition very similar to Usher syndrome. Comprehensive NGS-based panel analysis may identify the Refsum syndrome patients among those with apparent Usher syndrome (Zaki et al. 2016). Importantly, and in contrast to Usher syndrome and other deaf-blindness disorders, therapeutic options do exist for patients with mild PBD, who may benefit from a phytanic acid-restricted diet and extracorporeal lipid apheresis (Kohlschütter et al. 2012; Baldwin et al. 2010; Ruether et al. 2010).
3.1.6 Newborn Hearing Screening Should Be Supplemented by Comprehensive Genetic Testing
In view of the impact that an early genetic diagnosis has on the clinical management of patients and with the availability of comprehensive genetic testing with NGS of large gene panels, such genetic analyses should become part of the diagnostic workup in newborns and infants who fail hearing screening.
3.2 Genetic Counselling
3.2.1 Introduction
The expanding knowledge in human genetics has led to practical applications at an increasing rate in genetic counselling. Conventional and invasive diagnostic procedures have been complemented or entirely replaced by genetic testing. DNA tests allow the prediction of diseases and the modification of risk figures. With increasing numbers of both diagnostic and predictive genetic tests available, genetic counselling is becoming more important in clinical practice.
Genetic counselling is an important area of applied human genetics. Patients request advice or are referred by their physicians for counselling in order to understand biological facts, medical implications and recurrence risks of genetic diseases (Fletcher et al. 1985; Fuhrmann and Vogel 1983; Harper 2010; Resta 2006; Speicher et al. 2010).
3.2.2 Definition of Genetic Counselling
Establish the diagnosis.
Assess the recurrence risk.
Communicate the likelihood of recurrence to the patient and family.
Provide information regarding the many problems raised by the disease and its natural history, including the potential medical, economic, psychological and social burdens.
Provide information regarding potential reproductive options, including prenatal diagnosis.
Provide referral of patients to appropriate specialists.
The range of problems and questions arising during genetic counselling covers a wide area. Generally, not all patients and families turn out to have classical genetic illnesses, such as monogenic diseases or chromosomal aberrations. Many consultations deal with various birth defects, mental retardation, delayed development, dysmorphic-looking children, short stature and similar problems that may or may not have a genetic cause.
3.2.3 Indications of Genetic Counselling
Important questions in genetic counselling are as follows:
Birth of a Child with a Congenital or Developmental Disorder
If a child with birth defects or developmental delay is born to healthy parents, the most common question concerns the risk to any other of their children.
A Parent Is Affected
The illness of a parent is a frequent concern leading to the question of recurrence risk in the parents’ children. If the person consulting is affected or even pregnant, the question of the impact of pregnancy on the course of the disease is another important issue.
Diseases or Developmental Disorders in Relatives of an Affected Person
As a rule, this situation requires communication with multiple members of a family. Careful pedigree analysis and risk calculations frequently yield relatively low risks. The issue of predictive testing for late-onset diseases in unaffected but at-risk family members must be considered carefully.
Age Risks
Owing to an increasing number of pregnancies in women of advanced age in Western societies, the demand for counselling in this topic is increasing. Most consultants are aware that increased maternal age increases their risk of having a child with a chromosomal disorder. Elevated paternal age increases the risk of point mutations, but this risk is relatively low (1% or less).
Teratogenic or Mutagenic Effects
A child’s exposure to exogenous factors such as drugs, radiation, alcohol or prenatal infections during pregnancy (teratogenic risks) is a frequent indication for genetic counselling. Potential risks for adverse effects, however, are often overestimated. The number of drugs with proven teratogenic or mutagenic effects is rather small (Shepard and Lemire 2010).
A history of drug and alcohol consumption should be carefully evaluated and discussed. Prenatal infections represent complex situations that require interdisciplinary management.
Consanguinity
First cousins and more remote relatives who contemplate marriage occasionally ask for advice about the risks of having children with inherited diseases. Consanguinity definitely increases the risks of disease caused by homozygosity of recessive genes, but the absolute risks are relatively low. It has been estimated that the rate of various diseases, birth defects and mental retardation among offspring of first-cousin matings is at most twice the background rate faced by any given couple. These risks are even lower for more remote consanguinity and thus are difficult to separate from the population background rate for such disorders.
3.2.4 Genetic Diagnosis
Accurate diagnosis of a genetic disease by the use of all the modalities of modern medicine is the cornerstone of genetic counselling. Diagnostic accuracy is emphasised since similar phenotypes may sometimes have different modes of inheritance or may not be inherited at all. The family history is important because a clear-cut pattern of inheritance such as in autosomal dominant traits often provides the basis for counselling when a definitive diagnosis may not be clear. Previous medical and hospital records are helpful in arriving at a correct diagnosis. Since many genetic diseases are associated with somewhat characteristic facial features, inspecting photographs of family members may be helpful. Chromosomal examinations in addition to array CGH analysis are frequently required in the diagnosis of complex birth defects. Since many genetic diseases are rare, even trained medical geneticists and specialists in a given field of medicine may have difficulty in arriving at an accurate diagnosis. They cannot be equally knowledgeable about all genetic diseases in every area of medicine but do need to be aware of recent monographs and computerised expert systems to establish the appropriate diagnosis. The Catalogue of Mendelian Traits in Man by McKusick and its computerised version OMIM are helpful (OMIM et al. 2016).
A definitive clinical diagnosis often cannot be made even by experienced specialists owing to the enormous complexity of development and its possible perturbation by frequently unknown genetic, epigenetic and environmental factors. Fewer diagnostic uncertainties occur with monogenic diseases than with various birth defects. However, even in this area, the growth of the McKusick catalogue over the years (i.e. from 866 definitive loci in 1971 to more than 15,000 definitive loci in November 2016 (OMIM et al. 2016)) attests to the rapid expansion of knowledge in this field.
3.2.5 Molecular Diagnosis
As more genes are being cloned and the molecular nature of mutations causing disease becomes known, direct DNA diagnosis of genetic disease is increasingly possible. Unlike indirect diagnosis from the use of linked DNA markers, a family study is not required for direct genetic testing. However, the exact nature of the mutation to be detected must usually be known. It is good practice to isolate and store DNA from patients with genetic diseases for appropriate future study. The resultant information may be of great help in counselling family members in the future.
Many diseases such as hearing loss and language delay are genetically heterogeneous with many different disease-causing genes. With increasing identification of disease-causing genes in combination with improving novel sequencing techniques such as exome analysis or panel diagnostics, the analysis of many candidate genes in a single step allows the disclosure of the underlying genetic defect in an increasing number of affected families.
3.2.6 Multifactorial Disorders

Threshold model for multifactorial (disease) traits. For multifactorial conditions, it is postulated that there is a liability threshold. People whose liability is above this will manifest the disease. A certain combination of genetic and environmental factors, which add together, is needed in order for a person to be affected with a multifactorial condition. Left curve, distribution of liability in the general population; right curve, in relatives of affected individuals, according to the multifactorial threshold model
In contrast to that of monogenic disorders, the risk in multifactorial disorders increases with an increasing number of affected relatives, with increasing severity of the disease, and is usually negligible for distant relatives.
Careful search for the rare monogenic variety of a disease that appears multifactorial must always be kept in mind.
Transmitted chromosomal abnormalities, such as translocations, do not segregate by Mendelian ratios, and counselling must be based on empirical risk figures.
3.2.7 Recurrence Risk
Genetic risks in Mendelian diseases are clearly defined and depend upon the specific mode of inheritance (Fuhrmann and Vogel 1983; Harper 2010; Speicher et al. 2010). The actual clinical risks to the patient, particularly in autosomal dominant inheritance, depend upon variable penetrance and expression, especially in late-onset disorders. Patients are more interested in the actual recurrence risk of the clinical feature than in the formal genetic risks alone. In diseases with decreased penetrance, the actual recurrence risk is lower than the formal risk of genetic transmission. For example, an offspring’s risk of an autosomal dominant disease with 70% penetrance is 35% rather than 50% (0.5 × 0.7 = 0.35). The risk declines with late-onset diseases, as a person remains unaffected beyond the age at which the disease first becomes manifest. McKusick’s catalogue is available as a computerised data base online (OMIM) and is updated frequently (OMIM et al. 2016).
3.2.8 Communication and Support
The meaning of genetic risks must be conveyed in terms that can be understood by patients. The probability that 3–4% of all children of healthy parents are born with birth defects or possible genetic diseases should be communicated as a baseline risk figure that applies to the general population. There may be problems in communicating the extent of uncertainty. For example, with a sporadic case of a non-diagnosable disorder, the risk might be zero if the disease is non-genetic, 2–3% if there is a multifactorial aetiology, and 25% if it is caused by an autosomal recessive trait. It must be emphasised that the individual risk of a specific family can differ significantly from the empirical risk figure available for this constellation. Empirical risk figures include families with individual higher as well as lower risks that require special explanation in genetic counselling.
The physical as well as emotional burden of the disease must be discussed. It is well known that very severe but invariably fatal conditions in early life often carry a less severe burden to the family than those associated with chronic or slowly progressive diseases. Since problems may be complex and may prove to be emotionally difficult for the patient, it may be necessary to have several counselling sessions. In any case, the counsellor should provide a written summary of the counselling session by using lay language.
3.2.9 Directive Versus Non-directive Genetic Counselling
The practice of medical genetics clearly has evolved in the direction of non-directive counselling. Non-directive genetic counselling mirrors the trend to increasing patient autonomy. Since each family is unique and reactions to risks vary, non-directive counselling fosters mature decision-making. However, absolutely neutral advice is rarely possible or even desirable. The person or family requesting advice usually wants and needs more than a computer-like professional who only dispenses facts. The counsellor may unconsciously emphasise the more positive or the more frightening aspects of a given disease. These feelings tend to affect the counselling process directly or indirectly, often by nonverbal clues. Not all couples have the necessary educational background and social or emotional maturity to make fully informed decisions. In addition, many couples expect the medical geneticist, whom they consider an experienced expert on their disease, to assist them in arriving at a decision they can live with. ‘What would you do if you were in our position?’ is a frequent question from those counselled, regardless of background. However, since a couple’s economic situation, religious affiliation and cultural background may differ substantially from that of the counsellor, the counsellor’s choice for his or her own circumstances is usually not appropriate. Reproductive decisions differ widely among individual couples even if the genetic facts and the disease burden are identical.
3.2.10 Conclusions
Knowledge of human genetics can be applied to genetic counselling of individuals and families at risk of hereditary anomalies and diseases. Genetic counselling refers to the sum of activities that (a) establish the diagnosis of such diseases, (b) assess the recurrence risk, (c) communicate to the client and family the chance of recurrence and (d) provide information regarding the many problems raised by the disease, including natural history and variability of the disease. Formal methods applied in risk assessment include the use of genetic algorithms, statistical considerations and empirically derived risk estimates if the mode of inheritance is unknown. An increasing number of underlying gene defects can be diagnosed at the DNA level, thus allowing a precise assessment of genetic risks for members of affected families. Genetic counselling is not merely concerned with scientific issues that are at the root of genetic disease. It must provide understanding and empathy for the clients’ concerns, and it must deal appropriately with the many psychological aspects of the process. As such, genetic counselling is an important part of comprehensive medical care (Fletcher et al. 1985; Resta 2006).
3.3 Neuropaediatric Approaches to a Child with Suspected Language Disorder
3.3.1 Introduction
Developmental disorders in children are often accompanied by language disorders. Such language disorders can have structural, functional or psychological causes. They can also be a consequence of complex disorders with impaired sensomotoric and cognitive abilities.
The development of language is based on a number of capabilities of the central nervous system. It depends on an intact primary and secondary auditory pathway, the sensomotoric development of the speech organs and the integrity and proper functioning of various structures of the cerebral cortex and their neural connections.
Early indicators of language delay or communication disorder
Lack of consonant and vowel utterances | By 10 months of age |
Lack of pointing | By 18 months of age |
Fewer than 50 words | By 2 years of age |
No two-word combinations | By 2 years of age |
3.3.2 Diagnosis
Articulation disorder
Disorder of expressive language
Disorder of receptive language
Language impairments due to the environment
Secondary language impairments
Differential diagnosis of developmental language disorder
• Hearing impairment |
• Intellectual disability |
• Oral motor dysfunction |
• Autistic spectrum disorder |
• Selective mutism |
• Landau Kleffner syndrome/epileptic encephalopathy |
• Environmental deprivation |
Evaluation of a child with a suspected language disorder
Methods | Recommended age for investigation (years) |
---|---|
• Complete neurodevelopmental and family history | 2 |
• Detailed neurological examination including social interaction and communicative behaviour | 2 |
• Hearing test | 2 |
• Developmental screening test, e.g. Denver Developmental Screening Test (DDST), 0–6 years (Frankenburg et al. 1992) | 2 |
• Psychometric testing to establish general cognitive function, e.g. Snijders-Oomen Nonverbal Intelligence Test—Revised (SON-R), 2½–7 years (Tellegen et al. 1998) | 3 |
• Depending on history and examination, other tests to consider: EEG – Sleep EEG – Genetic studies: karyotype, fragile X study, array CGH – Metabolic screening: urinary analysis of organic acids, creatine and guanidine acetate, mucopolysaccharides and oligosaccharides. Blood lactate, specific enzyme activities in leukocytes – MRI of the brain | 2–4 |
Language regression
Verbal auditory agnosia
Genetic syndromes known to be associated with language delay/impairment
• Fragile X syndrome |
• Klinefelter syndrome |
• Trisomy 21 |
• Angelman syndrome |
• Smith-Magenis syndrome |
• Rett syndrome |
• Velocardiofacial syndrome |
• Prader-Willi syndrome |
A number of metabolic disorders can initially present as a developmental language disorder. Mitochondrial respiratory chain defects, organic acidurias and disorders of creatine biosynthesis (Vodopiutz et al. 2007) especially are found within the first years of life. The lysosomal storage diseases mucopolysaccharidosis type III (Sanfilippo syndrome) (Cleary and Wraith 1993) or the juvenile progressive form of Tay-Sachs disease (Fernandez-Filho and Shapiro 2004) can also be mistaken as a developmental language disorder. A metabolic screening test and specific determination of enzyme activity in leukocytes or on dried bloodspot cards (Table 3.3) should be performed in cases of clinical suspicion.
If clear neurological deficits or pathological EEG findings are reported, a cranial MRI scan is required in order to identify structural changes in the brain. Neuronal migration disorders (Guerreiro et al. 2002), congenital bilateral perisylvian syndrome (Brandao-Almeida et al. 2008; Nevo et al. 2001) and a neurocutaneous syndrome, such as tuberous sclerosis, are clinical entities that can be detected.
3.3.2.1 Neurophysiological Studies in a Child with a Global Developmental Disorder
Electroencephalography (EEG)
Method
Fluctuations in field potentials caused by activity in the underlying brain structures can be recorded on the scalp. The majority of the activity which can be ascertained on EEG is derived from postsynaptic potentials, which are summed as field potentials over the cerebral cortex. With a defined distribution of electrodes covering the four areas of the brain (frontal, parietal, temporal and occipital regions), the multiline graphical plot of the EEG can display a topographical structure.
Cortical potentials are susceptible to internal as well as external influences, so monitoring conditions must be clearly defined and followed. Photostimulation, hyperventilation and sleep deprivation are used as provocation methods.
Indications
Identification of electrical patterns typical of epilepsy, such as spikes and sharp-waves that are important for diagnosis, classification and observation of children who suffer from epileptic seizures
Searching for focal disorders as evidence of brain area-related dysfunction
Change in background activity as a sign of globally impaired brain function, such as toxic-metabolic or inflammatory changes in the brain
Assessment of bioelectrical maturation
Limitations
Interpretation of the EEG is much more difficult in children than adults. Alongside the specific challenge of complying with the monitoring conditions, particular sharp-waves and rhythmic waves, especially related to tiredness, falling asleep or sleeping, are present in healthy children, which could be misinterpreted as epileptiform patterns
Because of the spatial separation between the scalp and the cortex, within which various conducting media lie (skin, bone, meninges, vessels, fluid):
Definite topographical mapping of EEG potentials is not possible
Epilepsy cannot be ruled out, even in the absence of patterns that are typical of epilepsy
Visually Evoked Potentials (VEP)
Method
Light stimuli on the retina generate potentials that are transferred along the optic nerve, optic chiasma, lateral geniculate body and Gratiolet line of sight to area 17 (area striata) of the occipital lobe (the primary visual cortex). By an averaging technique, a summation of numerous evoked potentials can be recorded over the scalp. Older children are required to fixate on the centre of a rapidly reversing chessboard pattern shown on a screen. Newborns and infants receive retinal stimulation via LED-light-flash glasses.
The VEP curves that are measured by using either of these two stimulation methods correspond to cortical potentials with age-related, relatively constant latencies and amplitudes.
Indications
Disorders of the myelin sheath lead to a prolonged latency of the VEP
Axonal damage to the optic nerve leads to a more or less pronounced reduction in VEP amplitude
No VEP is found in cortical blindness
In certain forms of neuronal ceroid lipofuscinosis, high-amplitude short-latency VEP at the beginning of the disease, or the loss of VEP in a later stage, can give a clue to this group of diseases
VEP testing should be considered in the following clinical settings: optic neuritis, in isolation or as part of multiple sclerosis; leuko-encephalopathy; tumour of the visual pathway (optic glioma as part of neurofibromatosis type 1, craniopharyngioma, etc.); papilloedema as part of cerebral phantom tumour; optic atrophy; cortical blindness; and neuronal ceroid lipofuscinosis.
Limitations
Arguments for limitations of this method are:
Noncompliance with the measurement conditions can easily lead to measurement errors. The informative value of the investigation can therefore be limited in children.
Somatosensory Evoked Potentials (SSEP)
Method
Through repeated suprathreshold sensitive skin stimulation with a defined square pulse of very short duration, sensitive or sensory neural action potentials can be triggered, which are transmitted to the central nervous system. The afferents in the neural pathways begin in free nerve endings or in special receptor organs in the skin, following the sensitive parts of the nerves through the dorsal root of the equilateral posterior funiculus in the spinal cord (spinothalamic tract) through the cuneate nucleus (upper extremity) or the gracile nucleus (lower extremity) into the medulla oblongata and, after crossing in the pons, through the medial lemniscus to the thalamus. Onward transmission of the signal takes place after switching to the second central neuron over thalamocortical pathways passing through the internal capsule into the sensory postcentral region.
According to the somatotopic structure of the cortex, the SSEP are recorded over the contralateral scalp in reference to the area of stimulation. The choice of nerve to be stimulated (e.g. the median nerve in the area of the wrist or the tibial nerve in the area of the ankle) depends upon the clinical issue that is investigated.
Owing to increasing myelinisation and fibre thickness of the conducting nerves and maturation of the synapses in the developing child, the spinal and central conduction time decreases from birth until the sixth to eighth year of life. At the same time, the latency of the SSEP increases because of increasing body length. Age and body-length-related reference values should be applied to the child being investigated.
Indications
Diseases of the peripheral nervous system (sensory polyneuropathy, entrapment syndrome, polyradiculitis, plexus/nerve root affection)
Diseases of the spinal cord (myelitis, spinocerebellar ataxia, tumour, vascular changes, tethered cord)
Diseases of the central nervous system (leuko-encephalopathy, tumour)
Forms of neuronal ceroid lipofuscinosis (as in VEP, some high-amplitude, short-latency SSEP, also loss of SSEP)
Limitations
Only fast-conducting nerve fibres are stimulated (tactile sensitivity and proprioception)
Disorders of pain or temperature sensitivity are not registered
Motor Electroneurography
Method
The stimulation of a motor nerve triggers a muscle action potential (MAP). The nerve conduction velocity (NCV) of the stimulated nerves is calculated from the distance between a distal and proximal site of stimulation and their difference in latencies to the site of measurement—defined by MAP. The NCV of peripheral nerves, with their variously structured fibres, depends upon the thickness of the myelin sheath, fibre cross section and body temperature. The myelination of peripheral nerves undergoes a developmental process that begins in the 15th week of gestation and can extend up until the fifth year of life, depending on the type of nerve fibre.
The most important parameters when analysing neurography traces are latency (which is an indicator of myelin sheath function) and amplitude (which provides information about the axonal integrity of the nerve fibres). Age-related reference values exist for NCV, distal latency and amplitude of the MAP.
Indications
Demyelinating and axonal neuropathy
Axonal lesions
Entrapment syndrome
Leuko-encephalopathy with involvement of the peripheral nervous system
Limitations
Arguments for limitations of this method are:
Stimulation must be at a supramaximal level; otherwise the detected NCV will be too slow. Full cooperation of the child is therefore necessary.
3.3.2.2 Neurometabolic Studies in a Child with a Global Developmental Disorder
Basic Laboratory Studies
Blood
Full blood and differential leukocyte count (looking for vacuolated lymphocytes), glucose, pH and blood gases, lactate, sodium, potassium, calcium, phosphate, alkaline phosphatase, transaminases, creatine kinase, creatinine, uric acid, homocysteine, iron, ferritin, thyroid-stimulating hormone (TSH), free triiodothyronine (fT3), free thyroxine (fT4) and very-long-chain fatty acids. If neonatal metabolic screening has not been done: amino acids and acylcarnitines in tandem mass spectrometry,biotinidase.
Urine
Organic acids, creatine and guanidinoacetate.
Further Tests According to Clinical Findings
Microcephaly: toxoplasmosis and CMV serology, possibly also CMV DNA analysis (PCR) on postpartum preserved dried blood spot card if necessary. In girls one should consider a genetic investigation for Rett syndrome
Dysmorphia: isoelectric focusing of transferrins (congenital disorder of glycosylation (CDG syndrome)), copper/ceruloplasmin (Menkes disease) and 7-dehydrocholesterol (Smith-Lemli-Opitz syndrome). Genetic investigations such as chromosome analysis, fragile X syndrome investigation and array comparative genomic hybridisation (aCGH). Targeted genetic investigation for suspected monogenetic disease. Oligosaccharides/mucopolysaccharides in urine
Autistic behaviour: purine and pyrimidine metabolites in urine
Cherry-red spot fundus: specific determination of enzyme activity in leukocytes or on dried blood spot cards (GM1 and GM2 gangliosidosis, Niemann-Pick disease)
Cerebral seizures: investigation of enzyme activity PPT1, TPP1 on the dried blood spot card for infantile or late-infantile neuronal ceroid lipofuscinosis
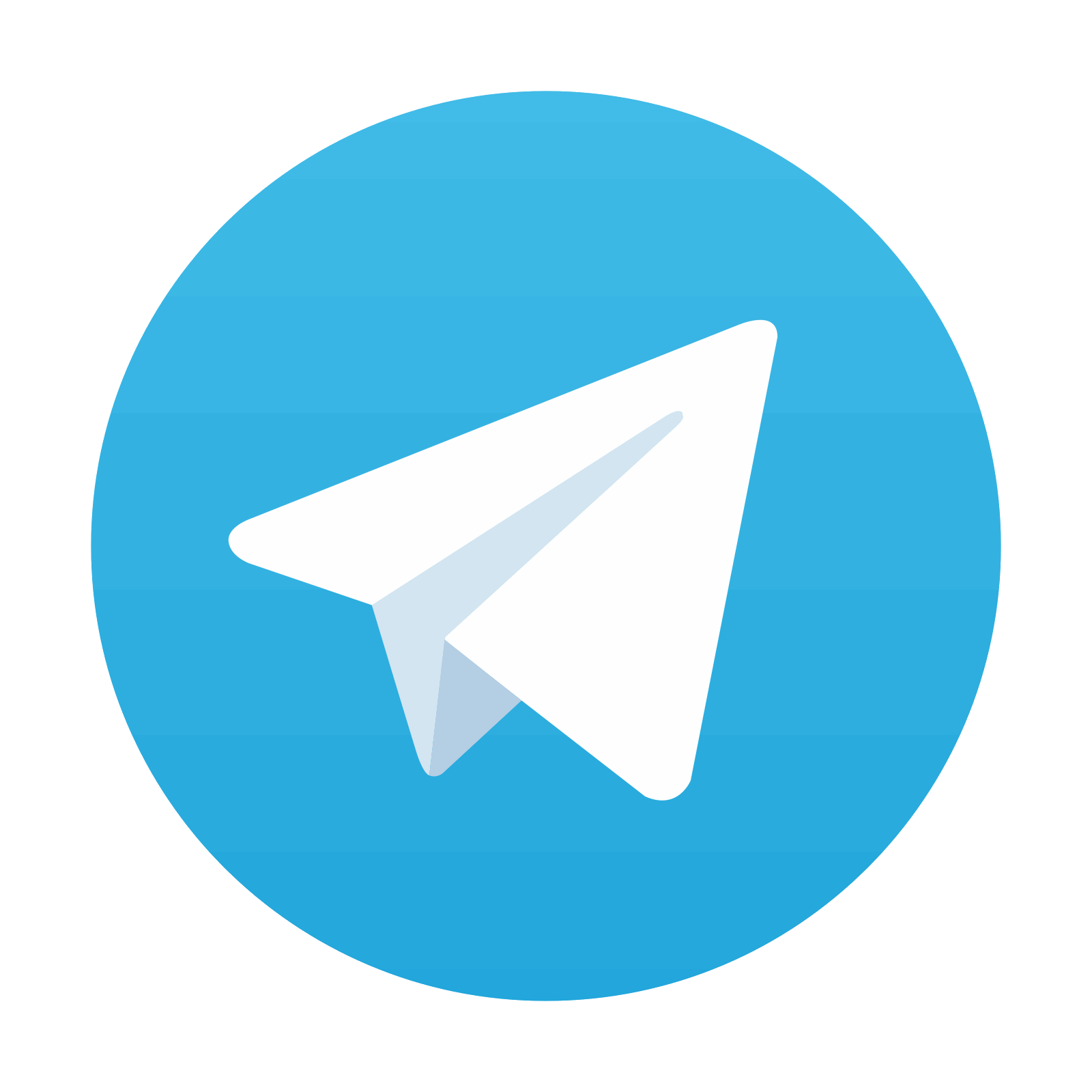
Stay updated, free articles. Join our Telegram channel

Full access? Get Clinical Tree
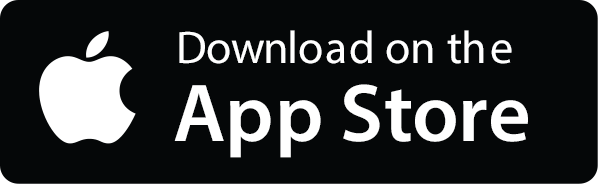
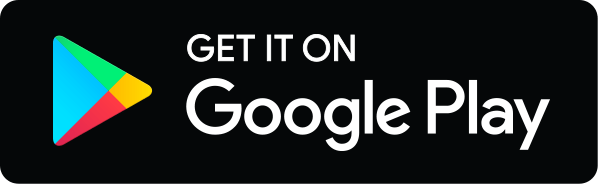