Gestational age (weeks)
Central comeal thickness (μm)
Horizonta corneal diameter (mm)
30–32
Mean
691
8.0
SD
87
0.2
34–35
Mean
648*
8.5*
SD
72
0.3
37–38
Mean
605*
8.9*
SD
59
0.3
39–41
Mean
564*
9.6*
SD
34
0.5
Central corneal thickness measured with an ultrasound pachymeter has been shown to be significantly greater in the premature newborn than in full term infants in the absence of any other ocular abnormalities. In a study of 33 patients with central corneal thickness (CCT ) measurements taken between 30 and 32 weeks and again at 39–41 weeks post conception, the CCT decreased from a mean of 691 μm to a mean of 564 μm [4]. Other studies have also shown the CCT to be thicker in the premature infant with a linear decrease as the child matures. Explanations offered for this remodeling process include better control of corneal hydration after the infant begins to open the eyes after birth. The hydration of the central cornea and the increased corneal thickness have also been suggested as the reasons premature corneas are often quite cloudy until the child approaches roughly 31 weeks post conceptual age [8].
Another important feature associated with central corneal thickness in premature infants relates to the accuracy of intraocular pressure measurements (IOP ). Common devices used to measure IOP in premature eyes are the Tonopen (Reichert Technologies, Buffalo, NY, USA), the Icare tonometer (Kansas City, KS, USA and Helsinki, Finland), and the Perkins Tonometer (Haag-Streit USA and Reliance Medical products, Mason, Ohio). In a study of premature and term infants correlating IOP and central corneal thickness, Karahan et al. found that CCT did not affect IOP significantly in preterm infants and was only moderately correlated in full term infants [9]. In contrast, Uva et al. found that IOP measurements in premature infants using the Tonopen XL were slightly greater than in full term infants because of an increased CCT . They found the mean IOP in premature babies was 18.9 ± 3.7 mmHg with a mean CCT of 599 =/− 36 μm. In full term infants the IOP was 17 ± 2.6 mmHg with a mean CCT of 576 ± 26 μm [8].
Anterior Chamber
The uses of ultrasound biomicroscopic measurements (UBM ) have greatly enhanced the understanding of anterior chamber development in the preterm infant. This technology allows for accurate imaging and measurements of the anterior segment to be taken in premature eyes. This is particularly valuable when the media are not clear or a child is confined to an incubator and access with a portable slit lamp is nearly impossible. Anterior chamber depth can be measured as the axial distance from the corneal endothelial surface to the anterior surface of the lens. Also measurable are the trabecular-iris angle and iris thickness. In a study of 39 premature infants born from 25 to 39 weeks gestational age, Kobayashi et al. established normative values related to postconceptional age and birth weight for these measurements [10]. They found that each value showed a linear relationship with post conceptual age and birth weight. Therefore, the younger the child, the more shallow the anterior chamber depth, the narrower the trabecular-iris angle, and the thinner the iris tissue .
UBM has also been used to evaluate angle closure glaucoma in premature infants which has been noted to occur in the advanced stages of retinopathy of prematurity (ROP). In a report of three infants, high resolution UBM confirmed angle closure in the setting of advanced ROP with a retrolental membrane. After peripheral iridectomy, repeat UBM showed an open angle in each of these infants. The authors concluded that a pupillary block mechanism was the cause of the angle closure in these infants [11].
More recently, hand-held spectral domain ocular coherence tomography (SD-OCT ) (Bioptigen, Inc, Morrisville, NC) has been used to provide excellent quality images of the anterior segment in infants. As with UBM , this technology is particularly helpful when the cornea is cloudy and the direct view is compromised (see Fig. 2.1a, b).


Fig. 2.1
(a) Hand-held spectral domain ocular coherence tomography (SD-OCT ) (Bioptigen, Inc, Morrisville, NC) image of a premature infant with a markedly thickened central cornea. (b) The normal cornea of the fellow eye is shown next to it
Lens
Transient lens opacities can occur in premature infants. These were initially reported by McCormick and have later been confirmed by others [12]. Alden found 2.7 % of infants examined with a birth weight of less than 2500 g had opacities present. These transient opacities were distinguishable from neonatal cataracts by their appearance and clinical course. The lens changes were symmetrical, bilateral, and consisted of clear fluid vacuoles just anterior to the posterior lens capsule. The vacuoles were initially found in clusters corresponding to the apices of the posterior inverted Y suture of the lens. These progressed in varying degrees up to a total vacuolar opacification of the posterior subcapsular space. The onset of the lens opacities was estimated to be 16 ± 4 days post-partum and had a mean duration of 25 ± 30 days until clearing. Resolution occurred in a manner opposite to the initial formation, with initial clearing centrally and the most prolonged retention of vacuoles at the lens apices [13].
The tunica vasculosa lentis may be a prominent feature in the examination of the preterm infant. It can be a contributory factor to the hazy view of the posterior pole seen in these patients. This represents a branching capillary network on the posterior lens capsule that extends anteriorly around the lens capsule. It has been suggested by Hittner et al. that the presence of this network between 27 and 34 weeks is a useful adjunct in accurately estimating the gestational age of a preterm infant [14]. Another consideration with persistence of the tunica is whether it may compromise transpupillary laser treatment for threshold ROP or perhaps predispose the child to cataract development. Paysse et al. reported a very low incidence of acquired cataract following diode laser treatment for threshold ROP. They indicated the mechanism for this cataract formation is unclear, but postulated it is more likely the result of thermal damage from absorption of laser energy by lens proteins or hemoglobin contained in a persistent anterior tunica vasculosa lentis. They reported an incidence of acquired cataract of only 0.003 % using diode laser therapy and suggested the incidence should be lower with diode than argon because of the reduced absorption of diode laser energy by hemoglobin [15]. In support of this hypothesis, other reports have shown an incidence of 1–6 % of cataract development after transpupillary argon laser photocoagulation in the setting of a persistent anterior tunica [16, 17]. Of note, there have been several reports of rapid resolution of the tunica vasculosa lentis after injection with bevacizumab, an anti-vascular endothelial growth factor medication that has been introduced into use for threshold ROP which will be discussed later in the chapter [18, 19].
Posterior Segment
Vitreous
The vitreous in the premature infant is often hazy, providing a less than optimal view of the peripheral retina. This is particularly true in infants less than 34 weeks gestational age, and is compounded by the premature corneal haze and the persistent tunica vasculosa lentis that have been discussed previously. By the end of the sixth to seventh month of gestation, the primary vitreous and hyaloid vasculature tend to atrophy and regress leaving a clearer secondary and tertiary vitreous gel. If this regression fails to occur, either partially or completely, this results in persistence of the fetal vasculature (PFV ). In PFV , a fibrovascular stalk connects between the optic nerve head and the posterior lens capsule. A whitish fibrovascular membrane covers the posterior lens capsule to varying degrees and the eye may be microphthalmic with cataract development. If the membrane is large enough, it may result in traction on the ciliary processes and will pull them centrally toward the pupil. Angle closure glaucoma is a potential risk of this process.
Retina
In 1986, Isenberg described the ophthalmoscopic appearance of the developing macula in a series of 129 premature infants [20]. He correlated the developmental changes in this region to the gestational age of the infant. At 34 weeks, pigment was first noted in the macular area. By 36 weeks, a complete annular reflex was present, and by 42 weeks in normal infants the macula appeared adult-like. The 37 infants in the study who developed retinopathy of prematurity showed a delay of 2 weeks in macular development in the later stages.
A number of studies have suggested that the presence of ROP, or premature birth alone, alters the development of the central retina. Through the use of hand-held spectral domain ocular coherence tomography (SD-OCT ), it has been shown the central retinal thickness is significantly increased in preterm infants than in age matched full term controls [21]. The thickest central retinal area was found in infants treated with laser for ROP (Table 2.2). More recent SD-OCT studies have documented the development of the human fovea after premature birth. The technology has evolved to the point that all retinal layers that in the past were only observed by histologic study, can now be seen in vivo with dramatic detail (see Fig. 2.2) [22]. At 31–33 weeks post conceptual age, the foveal thickness is greater than that found in the adult fovea. In the center of the fovea at this stage, ganglion cell, inner plexiform, and inner nuclear layers can be seen. As the retina matures in ensuing weeks, the inner retinal layers migrate in a centrifugal fashion toward the periphery and the foveal pit forms more succinctly. In conjunction with this migration, parafoveal inner retinal layers increase in thickness in contrast to the more peripheral retina. The majority of this migration of the inner retinal layers occurs between 31 and 42 weeks gestational age [22]. This increase in thickness is thought to result in the observation of a macular annular reflex by 36 weeks post conception, but the characteristic foveal light reflex generally is not visible until 42 weeks post conceptual age [20].

Table 2.2
Mean of OCT parameters
Group I | Group II | Group III | Group IV | P | |
---|---|---|---|---|---|
Total macular volume (mm3) | 7.1 ± 0.3 (6.47–7.55) | 6.9 ± 0.4 (6.2–7.52) | 6.7 ± 0.33 (6.22–6.99) | 7.1 ± 0.3 (6.29–7.39) | 0.095 |
Foveal thickness (μm) | 220.4 ± 39.1 (165–284) | 198.6 ± 23.6 (176–248) | 190.7 ± 28.9 (160–231) | 164.7 ± 16.7 (136–191) | 0.002 |
Central retinal thickness (μm) | 240.6 ± 28.9 (201–286) | 223.3 ± 14.7 (208–253) | 218.9 ± 19 (191–248) | 199.6 ± 14.5 (171–221) | 0.002 |
Inner retinal thickness (μm) | 272.7 ± 23.5 (210–291) | 269.4 ± 15.9 (244–295) | 269.9 ± 14.7 (249–291) | 273.1 ± 13.5 (256–295) | 0.65 |
Outer retinal thickness (μm) | 243.4 ± 18.6 (198–267) | 239.9 ± 17.8 (210–264) | 239.7 ± 18.5 (217–279) | 249.9 ± 9.8 (235–262) | 0.252 |

Fig. 2.2
Hand-held spectral domain ocular coherence tomography (SD-OCT ) (Bioptigen, Inc, Morrisville, NC) cross sectional images of immature and mature retinas. On the left is the retina of a 31 week post conceptual age neonate born at 27 weeks and 1205 g. On the right is a 23 year old adult born at term. (Reprinted from Maldonado RS, O’Connell RV, Sarin N, Freedman SF, Wallace DK, Cotten CM, et al. Dynamics of human foveal development after premature birth. Ophthalmology. 2011;118(12):2315–25 [22]. With permission from Elsevier.)
An interesting finding that has arisen from SD-OCT studies in premature eyes has been the presence of cystoid macular changes. These are generally not visible on examination of the retina by indirect ophthalmoscopy. Vinekar et al. in a study of 54 premature infants with ROP and 20 controls, demonstrated that no control eyes or eyes with stage 1 ROP showed any foveal edema or disruption of architecture in that region. In contrast, 29.1 % of eyes with stage 2 ROP showed cystoid foveal changes. When these eyes were re-imaged by SD-OCT at 52 weeks post conceptual age, 100 % of the eyes had normalized by this visit. The authors postulate that the macular edema noted in the more severe ROP could be due to several causes. The first is an increase in VEGF levels in this setting and the second is mechanical traction exerted on the macular by the more peripheral acting ROP process. They considered it less likely that the ridge found in stage 2 ROP exerted significant tractional forces on the macula [23].
Other studies done to assess the retina of formerly preterm children with ROP include fluorescein angiogram (FA) and electroretinogram (ERG ). A smaller than normal foveal avascular zone has been documented in formerly preterm children. In children born before 30 weeks of post conceptual age, the normal remodeling of the avascular zone from a densely vascular area does not appear to occur fully. Of note, this smaller avascular zone does not correlate with visual acuity [24]. It should also be noted that the increased central macular thickness found in formerly premature infants discussed previously also did not correlate with visual acuity. Multifocal ERG studies have documented a significant reduction in amplitude and implicit time in children with a history of ROP as compared to age-matched controls. This is postulated to occur due to the impairment of the normal centrifugal movement of foveal cone nuclei and inner retinal cells in children with ROP. This arrest can result in the bipolar and amacrine cells remaining more central and causing the ERG findings [25].
Optic Nerve
During the second trimester of fetal development, the optic nerve contains about 3 million nerve fibers. During the third trimester, a number of the fibers are eliminated during the segmentation of inputs to the ipsilateral and contralateral lateral geniculate nuclei. An adult optic nerve is left with about 1 million nerve fibers. The optic nerve and optic tract are not completely myelinated at term birth and this process continues until roughly 2 years of age. The appearance of the optic disc is different in preterm infants, assuming initially an ovoid shape and over a period of several months normally becoming more round in shape. The optic nerve of a preterm infant often appears paler than that of an older child, regardless of racial or ethnic origins [26].
Optic Nerve Hypoplasia
Definition
An abnormally small optic nerve head that may appear pale or gray in color that may be surrounded by a peripapillary halo and bordered by a ring of either increased or decreased pigmentation. This process can be either unilateral or bilateral and may occur as an isolated event or be associated with midline brain defects.
History
The first case of optic nerve hypoplasia was described in 1877 by Briere. The first schematic drawing of the condition is attributed to Schwarz in 1915. The association of optic nerve hypoplasia with absence of the midline septum pellucidum was first described by Reeves in 1941. Dr. William Hoyt described in 1970 the association between optic nerve hypoplasia and growth hormone deficiency [27].
Epidemiology
Optic nerve hypoplasia is a common cause of congenital legal blindness. In 2007 the Babies Count Registry listed optic nerve hypoplasia as the third most common cause of blindness in infants. The first two were retinopathy of prematurity and cortical visual impairment. It was also attributed to be the most likely cause of blindness in children under the age of 3 years in the United States [28].
Systemic Manifestations
The most significant potential association with optic nerve hypoplasia is hypopituitarism. Infants manifesting this condition should be monitored carefully for associated endocrine abnormalities [29]. More recently, associations have also been established with hypothalamic dysfunction, developmental delay, and autism. Of note, these are all independent of septum pellucidum development . It is important to monitor these children carefully from an early age [30, 31].
Ophthalmic Manifestations
In a study of RetCam image analysis of the optic nerve in premature infants, Mcloone et al. sought to examine children with and without ischemic brain injury. These images were combined with serial cranial ultrasonography in order to date the brain injury in children with periventricular white matter damage. There is a well-established increased incidence of intraventricular hemorrhage (IVH ) with decreasing gestational age. In the above study, 61 % of the 109 premature infants with birth between 24 and 33 weeks gestation demonstrated IVH . In this population, only the infants with grade 4 IVH had significantly more hypoplastic discs than the normal control group. The median age of injury for the patients in the Grade 4 IVH group was 26 weeks post conceptual age and this group represented 8.3 % of the study population. 44 % of infants in this group were noted to have hypoplastic discs [29]. The authors recommend that given the potential association of neurologic and visual complications, preterm infants with Grade 4 IVH be referred for eye evaluation even if they fall outside of normal ROP screening criteria. Jacobsen et al. have postulated that if early prenatal damage occurs to the periventricular white matter, prior to development of the supporting tissues around the optic nerve, then a smaller optic disc size may result. They also reported that a small optic disc area in a child with periventricular leukomalacia or periventricular hemorrhage could predict the timing of the brain injury. A small optic disc area was only seen in children with white matter damage estimated to occur prior to 28 weeks of post conceptual gestational age. If the injury occurred after 28 weeks, they suggest that a normal sized optic disc would develop but it would have a large cup area and thin neuroretinal rim [32].
In a study by De Silva et al., optic nerve head dimensions were measured in 51 infants during routine ROP screening using the Retcam with either an 80 or 130° lens. Past studies were done using postmortem specimens and were subject to fixation and shrinkage artifact. The mean values obtained were horizontal disc diameter 1.05 mm ± 0.13, vertical disc diameter 1.41 mm ± 0.19, and mean disc area 1.17 mm2 ± 0.26. The infants studied ranged from 32 to 50 weeks post conceptual age and the authors found that the optic nerve head dimensions did not change significantly over this age range [33]. Other studies have also reported the optic disc parameters of premature infants such as optic disc area and cup-to-disc ratio did not correlate with birth weight or gestational age [30, 31]. The measurements taken of optic disc height and width were found to be larger than the values previously obtained from postmortem studies. Of note, De Silva et al. also reported a high proportion of eyes (23 %) to have a double ring sign classically ascribed to optic nerve hypoplasia . They suggest this may be a normal stage of disc development since their measurements would indicate the optic nerve enlarges by 50 % after birth to reach adult proportions. This growth largely abolishes the double ring sign in most and those that do not grow retain the double ring and are left with a hypoplastic nerve [33].
Refraction and the Premature Eye
History
Children with a history of prematurity, and particularly those that have had retinopathy of prematurity (ROP), have a higher incidence of myopia than their age matched counterparts. Another interesting finding that has been reported is that premature infants tend to have a higher degree of astigmatism, particularly in more severe cases of ROP [34, 35]. It remains a subject for debate as to which element(s) of the determination of refractive status are most responsible for the development of both myopia and astigmatism. A number of studies have been published over the last several years proposing several possible answers. These studies address axial length, corneal curvature, anterior chamber depth, lens thickness, and the impact of either laser or cryotherapy induced treatment changes as causative factors [36–41]. Other factors that have been implicated are bone mineral deficiency, temperature, lighting, and visual deprivation [42, 43].
Epidemiology
Quinn et al. have reported that in eyes with any stage of untreated ROP, and eyes treated with cryotherapy for threshold stages of the disease, the incidence of myopia increases during the first year of life [34]. Others have reported that myopia in preterm infants begins at about 6 months of age and severity increases between 6 months and 3 years of age [41]. The severity of the myopia is often linked to the severity and stage of the ROP that was present. Cross sectional and longitudinal studies have reported the rates of myopia in the pre-term population to be anywhere from 5 % to in excess of 80 % varying with the age at the time the examination was performed [44–46]. Choi et al. found in a study of 125 eyes that premature infants tend to initially develop myopia at the age of 6 months and progresses to the age of 3 years. The highest levels of myopia tend to develop in children that had cicatricial retinopathy, regardless of whether they received treatment with cryotherapy or not [41]. One common theme that emerges from most of these studies is that the myopia is not due to an increase in the axial length of the eye, but rather to factors related to the anterior segment of the eye such as the cornea, anterior chamber depth, and lens.
Ophthalmic Manifestations
The normative refractive status of full term infants is generally a moderate state of hypermetropia. This tends to persist for the first several years of life with a steady decline in hyperopic refraction through the childhood years as the emmetropization process proceeds. In premature eyes, there are several factors that may contribute to the development of myopia, which may be mild to severe. In a study comparing highly myopic eyes in preterm children with a history of ROP to an age matched group of patients with high myopia born at term without any ROP, Garcia–Valenzuela et al. found that increases in lens thickness and attendant power are the primary factors causing high myopia in ROP eyes. This finding was not related to stage of ROP attained or treatment of threshold ROP [47]. This finding led them to support a theory of altered anterior segment development for the resultant high myopia. They also found only a minimal increase in axial length as compared to age matched norms (mean of 23.36 ± 1.71 mm vs. 22.21 ± 0.80 mm). In the full term group with high myopia, the cause was primarily axial length with the mean value measured at 27.02 ± 1.87 mm. They advocate comparing the ratio of lens thickness/anterior chamber depth between ROP and full term highly myopic eyes to highlight the difference in anterior structures between the two groups. The eyes with ROP had a ratio 50 % higher than full term or normative eyes [47].
Fielder et al. proposed that myopia associated with prematurity was a result of the preterm cornea not appropriately flattening in the setting of the cooler environment outside of the womb [42]. Hittner et al. reported decreased anterior chamber depth in myopic eyes that had cicatricial changes secondary to ROP [48]. Laws et al. found that axial length was inversely related to increasing stage of ROP with the higher the stage reached, the lower the axial length recorded. This remained true after correction for gestational age, sex, birth weight, and head circumference. They also found that infants reaching threshold stage 3 disease had a shorter axial length than stage 3 infants not receiving treatment. In both cases, the growth in axial length was found to be linear [49]. Yang et al. found that laser treated eyes for threshold ROP showed a significantly thicker lens and shallower anterior chamber depth than full term control infants [50]. A reasonable conclusion is that the myopia associated with prematurity has an arrested development of the anterior segment structure as its root cause and is nonaxial in nature. Its cause seems to be more related to a steeper cornea and a thicker lens with associated shallower anterior chamber depth. Findings from the Early Treatment of Retinopathy of Prematurity Study (ETROP ) would also indicate that the increased myopia in patients treated with laser is not due to any direct effect of the laser on the retinal periphery, but rather due to the severity of the ROP [51]. In a follow on report regarding the progression of myopia to ages 4–6 years, the ETROP group found that approximately 2/3 of eyes of children that had high risk pre-threshold ROP will likely be myopic into the early school-age years. The proportional increase in high myopia noted in earlier age groups was not noted to continue between ages 3 and 6 years [52].
Another potential refractive outcome in the premature infant with ROP is the development of astigmatism. In a cross-sectional study of 24 consecutive preterm children treated with diode laser for threshold disease at age 9 years, Yang et al. sought to assess the prevalence of astigmatism in this group. They matched their results with 1021 full term controls from a national survey in Taiwan, Republic of China. They found that the laser treated eyes had a mean astigmatism of 3.47 ± 1.92 diopters, with a mean spherical equivalent of −4.49 ± 3.76 diopters. Age matched controls showed a mean of 0.08 ± 0.9 diopters, with a mean spherical equivalent of −0.44 ± 1.48 diopters. 98 % of the eyes studied showed astigmatic changes, the majority of which were with- the- rule with greater steepening in the vertical meridian. They found the astigmatism to be corneal in origin and reported a statistically significant steeper vertical corneal meridian and flatter corneal meridian than those found in the control group. Their hypothesis was that there is an incomplete postnatal development of the cornea, anterior sclera, and anterior segment in this population at the age of 9 years [53]. In an ETROP study report, Davitt et al. reported that by age 3 years, over 40 % of eyes with high-risk pre-threshold ROP will develop astigmatism of greater than or equal to 1.00 diopter and 10–20 % of these eyes will develop more than 2.00 diopters. There was no evidence that earlier treatment of this population, or the presence of stage 1 disease or plus disease significantly influenced the development of astigmatism. The majority of the astigmatism was with the rule in nature [51].
Ocular Infections in the Premature Infant
Definition
An ocular infection can be considered to be located anywhere on the surface of the eye, on the surrounding ocular adnexa, such as the lids or periocular skin, or inside the eye in the form of endophthalmitis . A series of infections with the collective acronym TORCH infections can have potentially devastating clinical and ocular manifestations. This acronym stands for Toxoplasmosis, Other (syphilis, Varicella Zoster), Rubella, Cytomegalovirus, and Herpes Simplex Virus. These infections, with the exception of Herpes Simplex, will be addressed in another chapter of this text.
History
Ophthalmia neonatorm or conjunctivitis of the newborn has been a public health concern that has been recognized for over 100 years. In late nineteenth century Europe, the prevalence of this condition exceeded 10 %, with blindness occurring in about 3 % of affected infants. About 50 % of the children in schools for the blind during this era were there due to ophthalmia neonatorum infection. In an 1881 paper, Crede’ published the impact of using a 2 % silver nitrate solution in reducing the number of cases of this disease dramatically. He realized that the majority of infections resulted from transmission of Neisseria gonnorhea as the child passed through the vagina at birth. His discovery led to a dramatic reduction in the disease throughout Europe [54]. In the modern era, silver nitrate has been largely supplanted in developing countries by erythromycin or tetracycline ointment. These antibiotics are far less irritating, seldom produce a chemical conjunctivitis, and also provide better coverage for Chlamydia trachomatis , the number one cause of ophthalmia neonatorum in developed countries today. Prophylaxis is recommended by the American Academy of Pediatrics and required by state law in many states, but is not being done automatically in other developed nations such as the United Kingdom [55]. Another option for prophylaxis worldwide, but not CDC approved in the United States, and one which is highly cost effective, is the use of povidone iodine 2.5 %. The cost of a 5 cm3 container of this substance is $0.10, as compared to erythromycin ointment at $0.74 [54]. Drug shortages of erythromycin in the United States in 2009 led to the use of alternative, unproven antibiotic substitutes [56].
Epidemiology
The duration of hospitalization for a newborn infant is inversely related to the gestational age at birth. The average length of stay for newborn infants born at 26 weeks is roughly 2 months. This population of extremely low birth weight infants generally undergoes a number of invasive procedures and is commonly exposed to mechanical ventilation, including high frequency jet and oscillation, continuous positive airway pressure devices (CPAP), endotracheal suctioning, prolonged use of central venous lines , and nasogastric tube feedings. This places them at high risk for the development of infections, a number of which can affect the eyes from either exogenous or endogenous sources. In a study of two Neonatal Intensive Care Units (NICU ) over a 2 year period and involving almost 3000 premature infants, conjunctivitis was found in 5 % of patients. An association was established between low birth weight, the performance of an eye examination, and respiratory support measures as a predictor for the development of conjunctivitis. The respiratory support measures implicated included nasal cannula delivered CPAP, endotracheal intubation, and mechanical ventilation [57]. Common pathogens isolated are identified in Table 2.3 with the most common pathogen being coagulase negative Staphylococcus Aureus. These results are consistent with previous studies published in the literature [58–60]. Another important factor to consider is the potential colonization of bacteria among mothers and infants which could contribute to the development of ocular infection in vulnerable preterm infants. Studies have shown that between 1–4 % of mothers and infants are colonized with the potentially serious Methicillin Resistant Staphylococcus Aureus (MRSA ). This organism may be vertically transmitted from mother to child during vaginal birth [61]. An important consideration is that in some cases of conjunctivitis in a premature infant, the causative organism may lead to a significant keratitis or endophthalmitis , and may also result in a life threatening systemic infection. Conjunctivitis in a preterm infant warrants a thorough evaluation with cultures and bloodwork.
Table 2.3
Organisms causing conjunctivitis in neonatal intensive care unit patients
Organism | Total no. of isolates a |
---|---|
Coagulase-negative Staphylococcus | 38 (25) b |
Staphylococsus aureus | 29 (19) |
Klebsiella spp. | 16 (10) |
Pseudomonas aeruginosa | 13 (8) |
Enterococcus spp. | 13 (8) |
Escherichia coli | 12 (8) |
Serratia marcescens | 12 (8) |
Enterobacter spp. | 10 (6) |
Streptococcus spp. | 9 (6) |
Other Gram-negative bacilli | 11 (7) |
Diphtheroids | 3 (2) |
Yeast | 4 (3) |
Culture not obtained | 33 (21) |
Total | 203 (131) a |
Ophthalmic Manifestations
Bacterial conjunctivitis in the preterm infant is generally heralded by a purulent discharge from one or both eyes accompanied by ocular redness and possible lid swelling. The discharge may be less copious than that observed in an older child with a similar infection due to the immature immune system. Gonococcal conjunctivitis is a notable exception and normally presents with a hyperpurulent discharge even in the preterm infant. The most frequent cause found in the United States and other developed countries is Chlamydia trachomatis . This is a sexually transmitted pathogen normally acquired by the child during the delivery process. The incubation period is generally 5–14 days after delivery and it may present either unilaterally or bilateral. Children born to infected mothers have a 30–40 % chance of developing conjunctivitis [62]. Unfortunately, according to the 2012 Red Book, there is no effective agent to date to prevent the vertical transmission of Chlamydia from infected mother to her infant. Infections can range from mild to severe with the mildest forms presenting with a clear discharge with thickening and erythema of the palpebral conjunctiva. More serious infections can result in a thicker discharge with pseudomembrane development or corneal involvement in the form of clouding or pannus [63].
Gonococcal conjunctivitis is also a sexually transmitted pathogen acquired by the infant during vaginal delivery. Classically this presents as bilateral hyperpurulent conjunctivitis with significant lid edema, chemosis, and potential formation of membranes or pseudomembranes. Its ocular consequences may be more severe with progression to corneal ulceration or perforation if not treated promptly. The organism is able to penetrate intact epithelial cells and multiply rapidly inside of them. The onset of the infection is usually within 48 h of vaginal delivery. In the modern era, this organism represents less than 1 % of the cases of neonatal conjunctivitis [59].
Another potentially serious bacterial pathogen in the preterm infant is Pseudomonas aeruginosa, which tends to colonize in water saturated respiratory equipment Although Pseudomonas is a less common cause of conjunctivitis, it is capable of progressing rapidly to corneal ulceration or perforation if not treated promptly. An outbreak in seven ventilated infants at a NICU in Brazil suggests that the conjunctivitis may have occurred from spread from endotracheal tube aspirates. The same strain of pseudomonas was isolated from the respiratory tract of two of the infected infants [64]. In an outbreak of this organism in a pediatric hospital involving 30 patients, 70 % of the patients were preterm infants in the NICU . All of the patients except one had respiratory care interventions prior to the onset of conjunctivitis. These included endotracheal tube, tracheostomy placement or suctioning of the respiratory tract. The authors stressed the need for caution and protection around the eyes when performing these measures [65].
Other organisms, both gram positive and negative, have been identified as causative agents in conjunctivitis in the preterm infant. Common gram positive organisms are Staphylococcus aureus, Staphylococcus epidermidis, Streptococcus pneumonia, and Streptococcus viridens. These collectively make up 30–50 % of the reported cases [66]. Common gram negative organisms isolated , in addition to the previously mentioned Pseudomonas aeruginosa, are Eschericia coli, Klebsiella pneumonia, Serratia marcesans, and Haemophilus influenzae . Very low birth weight infants with commensurate early gestational age in the NICU with signs of conjunctivitis should generate immediate concern for a possible gram negative etiology. Chen et al. reported the incidence of gram negative isolates causing conjunctivitis as 38 % in a NICU setting [67].
Viral conjunctivitis is another potential issue for the preterm infant. Most herpetic infections in the preterm infant are secondary to vertical transmission of HSV-2 transmitted to the infant during birth. This infection generally occurs within the first 2 weeks after birth and may affect one or both eyes. Clinical signs may be subtle and include lid edema, bulbar conjunctival injection, and a watery discharge. Typical dendrites are seldom noted but geographic ulceration of the cornea may be seen. Herpetic infection may also result in a retinopathy causing necrosis, vasculitis, and hemorrhage. Those with retinitis will often develop a cataract and the virus can often be isolated from these lenses [68]. Ocular signs may be the initial or only manifestation of herpetic infection although serious systemic disease can result, as will be discussed in the next section. If a mother with an active genital herpetic infection delivers vaginally, there is a 40–60 % chance the child will be infected [69]. In about 60 % of infections in the preterm infant, the mother will show no signs of active herpetic infection [70].
Another potential viral pathogen of the preterm infant is adenovirus. In an outbreak in a NICU in Israel, the virus was found in seven premature infants who had undergone an eye examination 4–7 days previously. Three of the infected infants went on the manifest systemic respiratory symptoms. The temporal relationship of the eye exams to the outbreak suggests direct inoculation of the infants by ophthalmic instruments or transmission by the involved personnel. Several of the affected children had underlying pulmonary disease and were being treated with steroids which likely exacerbated the course of the illness [71]. Respiratory related pathology is a very common finding in the preterm infant. The need for aseptic techniques using separate sterile instruments for each child in the NICU during exams cannot be overemphasized.
More serious ocular infections are also a very real threat in the premature infant. Endophthalmitis may result from both exogenous and endogenous sources. It has been reported that 80 % of neonatal endophthalmitis comes from endogenous sources [72]. In the cases of exogenous spread, conjunctivitis was the initial presenting sign. These infections are generally nosocomially acquired, often from contaminants from suction devices, nasal CPAP devices, or respirators. Typical exam features in the NICU are a red eye, a compromised red reflex, and corneal clouding. Pseudomonas aeruginosa has been identified as an exogenous source agent of neonatal endophthalmitis and has been reported to be the causative agent in more than 75 % of invasive eye infections in this population. Preterm infants are particularly vulnerable to infection by this organism [73]. Gaili and Woodruff reported a case of a preterm infant who initially became ill on day 12 of life and intravenous antibiotic treatment was begun, but there were no signs of ocular infection at that time. She was started on nasal CPAP. By day 21, the eye became “sticky”, and by day 22 there was a frank purulent discharge coming from one eye and the cornea became cloudy. A culture of the conjunctiva was positive for pseudomonas aeruginosa. Despite intensive intravenous and topical therapy, the eye perforated and was lost. They postulate an initial corneal epithelial injury that likely occurred via the CPAP apparatus and progressed rapidly [74]. Figueirdo et al. describe a similar case of pseudomonas aeruginosa endophthalmitis in a preterm infant born by caesarean section. In this instance, the infant initially developed septicemia and shortly thereafter developed a red eye with discharge, a cloudy cornea and hypopyon. Blood cultures subsequently revealed pseudomonas aeruginosa, but conjunctival cultures were negative. The mother’s wound cultured positive for the same organism. As in the first case, despite aggressive intravenous and topical therapy, the ocular infection progressed and the eye perforated and was lost [75]. These cases underscore the importance of aggressive treatment of ocular infections in the preterm infant. If the organism is fulminant, as in the case of pseudomonas, morbidity is very high and the infection can be life threatening.
Other forms of endophthalmitis can also occur in the preterm infant. Group B streptococci (GBS ) are a major pathogen causing bacterial infection in this age group. Endophthalmitis from this pathogen is unusual in the neonate, but has been reported [76]. Most of the cases are associated with both sepsis and meningitis from the organism and tend to occur weeks later than the onset of the systemic infection, even with appropriate intravenous antibiotic therapy. Group B streptococcal meningitis interferes with the normal blood flow autoregulation to the eye and may contribute to an increased risk of retinal pathology in this group [77].
Another form of endophthalmitis to which the preterm infant is susceptible is Candida. This is also a rare occurrence but the visual consequences are often devastating. It is almost always associated with a septicemia due to the Candida organism and is spread to the eye endogenously. Systemic candida infection has been reported to occur in 1.6–12.9 % of premature births, particularly in very low birthweight children [78–80]. Of note, there are a number of reported cases of endogenous Candida endophthalmitis in premature infants secondary to the organism remaining sequestered in the lens [81–83]. The endophthalmitis often presents after resolution of the systemic candidemia and is thought to be potentially due to the regression of the fetal hyaloid artery between 24 and 32 weeks post conceptual age. This permits the transmission of the fungus to the lens initially with subsequent sequestration. A cataract that may develop in this setting may actually represent a fungal abscess within the lens [84]. Fungal endophthalmitis may lead to necrotic retinal detachment and glaucoma, in addition to cataract.
Systemic Manifestations
A number of ocular infections in the preterm infant can be associated with serious systemic illness and may result in serious morbidity or mortality. Premature infants who contract a Chlamydia infection during the birth process have a 10–20 % chance of developing pneumonia related to this organism [85]. This generally results from an infection in the nasopharynx or aspiration of infected secretions from the mother during birth. The presentation is generally a respiratory distress with low grade fever and cough that may progress to apnea requiring ventilator support. The infection typically occurs around 3–6 weeks after birth but has been reported between 1 and 19 weeks of chronologic age [86].
Sepsis of early or late onset is not an uncommon occurrence in the preterm infant. Infant birth weight is inversely related to the risk of developing sepsis. Organisms that can cause ocular infection that can also result in sepsis include Group B streptococcus, Staphylococcus aureus, Coagulase negative staphylococcus, Klebsiella pneumonia, Haemophilus Influenza, Candida albicans, Pseudomonas aeruginosa, Serratia marcesans, and Neisseria gonorrhea [87].
Although intrapartum prophylaxis has significantly reduced early onset of GBS infection, late onset GBS sepsis is still common in the preterm infant. Most reported cases of associated endophthalmitis are associated with later onset disease and generally occur 1 week to 3 months after birth [76, 88]. The early onset infections are generally vertically transmitted from an infected or colonized mother during the birth process. The later onset infections are either acquired from colonized mothers, staff, or equipment in the NICU . With the increased survival rates of preterm infants, the burden of potential infection remains higher than ever. If a preterm child becomes septic, pediatricians often recommend a daily eye exam for early identification of ocular disease, and prompt ophthalmology consultation for any ocular signs [87].
Candida is a major cause of sepsis in the NICU in infants born less than 1500 g. Rates have been reported between 1.6 to 12.9 % in this population of infants, and morbidity and mortality rates approach 25 % [80]. The sources of systemic candidiasis are mostly endogenous, and infections are associated with total parenteral nutrition solutions and in-dwelling intravenous lines [89, 90]. Some authors suggest that candida sepsis should be strongly suspected after the third week of NICU admission in infants on mechanical ventilation and who have undergone treatments with multiple classes of antibiotics for other infections [91]. The general colonization rate for candida in infants who have been in the NICU environment for 1–3 months may be as high as 50 %, with the GI tract often being an early area affected [92]. Once the empiric or confirmed treatment for candida sepsis is begun, the ophthalmologist is often consulted to check the eyes for evidence of candida endophthalmitis .
Meningitis is another potential systemic complication of infections in the preterm infant that can also affect the eye. The potential organisms involved are those previously described that may result in sepsis. Meningitis is usually of later onset in this population, between 1 week and 3 months after birth. The most commonly identified organism causing meningitis in the premature infant is GBS , which is involved in about 50 % of cases. E-coli is the next most common pathogen, implicated in 20 % of cases, with Listeria monocytogenes next at 5–10 % [93, 94]. Another potential causative organism in neonatal meningitis worthy of mention is the Herpes simplex virus. The impact of a meningitis infection in these infants is significant. In a large study of 1500 babies with neonatal meningitis, 8 % had motor deficits, to include cerebral palsy, 7.5 % had learning difficulties , 7.3 % suffered from seizures, and 25.8 % had hearing impairments [95].
Diagnosis
The diagnosis of ocular infection in the premature infant may be more challenging than in the adult patient. Due to the increased susceptibility of the immature immune system, a healthy suspicion of a possible coincident systemic infection must be kept in mind. For a suspected conjunctivitis, the suggested evaluation would include a conjunctival swab for culture and sensitivity, a conjunctival scraping for gram and giemsa stain, polymerase chain reaction (PCR) testing for chlamydia, chocolate agar plating for Gonococcus or Haemophilus, blood agar for other bacterial species, and PCR for suspected Herpes infection. In the setting of any associated illness, blood cultures should also be performed immediately. Blood cultures should include both aerobic and anaerobic varieties, with results usually available within 36–48 h.
In the setting of a suspected endophthalmitis , organisms may be identified through vitreous culture, either from a biopsy or retrieval from a vitrectomy specimen. Most commonly the diagnosis is established by blood cultures. In a review of endogenous bacterial endophthalmitis , blood cultures were positive with the offending organism in 94 % of cases, and vitreous samples were positive in only 56 % [76]. To evaluate for fungal etiology, routine cultures can be ordered but are often low yield. More recently, the use of pan-fungal PCR testing has improved the yields on these cultures [96].
Management
In treating conjunctivitis in the preterm infant, initial treatment should be presumptive based upon initial gram stain or giemsa stain results. Consideration should be given to the potential for maternal infection and potential transmission of N. gonorrhea, Chlamydia, Herpes, or Group B streptococcus. Examination and culture of the parents may be necessary if suspicion is present. Time honored initial treatment of the conjunctivitis is the use of erythromycin ointment for gram positive organisms or topical gentamicin or tobramycin for gram negative organisms. It is also important to initially start intravenous antibiotics to cover for potential Neisseria gonorrhea infection or Chlamydia infection pending the return of culture results. The use of topical antibiotics alone is inadequate for the treatment of either of these forms of conjunctivitis and generally should not be used once the diagnosis is confirmed. Typical intravenous regimens would include either penicillin G or ceftriaxone to cover the Neisseria gonorrhea and erythromycin to cover Chlamydia [97].
Longer duration of stay in the NICU may predispose the infant to other pathogens that may cause conjunctivitis. Gram positive organisms, to include Methicillin Sensitive Staphylococcus Aureus (MSSA ) and Enterococcus have been reported in higher frequency with greater duration of stay. Gram negative organisms such as Pseudomonas aeruginosa and Serratia marcesans were more frequently cultured after the first 10 days of admission, presumably from nosocomial transmission [98]. These organisms, with the potential exception of MRSA (see next paragraph), can be effectively treated with topical fourth generation fluoroquinolone preparations. These medications have shown very low resistance patterns to most gram positive and gram negative isolates to date. This potent class of antibiotics tends to kill the organisms quickly, thus reducing the potential for bacterial mutation [99].
Bacterial conjunctivitis caused by Methicillin Resistant Staphylococcus Aureus (MRSA ) has also been reported in the neonate. A common practice in a number of NICU ’s is to take weekly pharyngeal and skin swabs to test for colonization of MRSA . These infants are typically not symptomatic, but colonization may be associated with a nasolacrimal duct obstruction. In these cases, it is possible that the parents or staff is also colonized and they should be checked accordingly [100, 101]. Current guidelines suggest treating MRSA infections with agents other than fluoroquinolones when possible. This is due to the high in-vitro resistance rates that MRSA have shown to these agents [102]. Other options in this setting would be topical vancomycin, a topical polymixin B- trimethoprim combination, and topical chloramphenicol, although the latter is seldom used in the United States and carries with it the potential complications of bone marrow hypoplasia and aplastic anemia.
The treatment of endophthalmitis , in the immature infant needs to be aggressive in nature. It is usually endogenous in origin, associated with sepsis, and often has a poor visual outcome. The diagnosis may be established from blood culture results or vitreous sampling. Treatment is generally with intravenous antibiotics and possibly intravitreal antibiotics. The normal blood ocular barrier is usually broken down in the setting of endophthalmitis , allowing reasonable absorption of intravenous antibiotics into the eye. Initial treatment involves empiric broad spectrum antibiotics for presumed bacterial infections. These generally include vancomycin and ceftazidime or amikacin , and arguably decadron. Some favor the administration of steroids and others do not. The dosage of vancomycin for a preterm infant is a 15 mg/kg loading dose, followed by 10 mg/kg/day. The infusion is generally given over a 1 hour period and dosing can be effectively adjusted using serum creatinine concentration and desired trough levels as a guide [103]. The dosage of ceftazadime in this age group is 25–100 mg/kg/day in two divided doses.
Some preterm infants may have a persistent endophthalmitis or even develop endophthalmitis while on appropriate intravenous therapy with blood levels in the therapeutic range. This has led to the recommendation to combine intravitral antibiotic injection with intravenous antibiotic use. Vitreous biopsy can be achieved at the time of injection of intravitreal vancomycin and ceftazadime . This provides excellent initial gram positive and gram negative coverage and can be modified accordingly dependent upon gram stain and culture results. The normal dose of intravitreal vancomycin is 1.0 mg/0.1 mL and for ceftazadime 2.25 mg/0.1 mL [104, 105].
Vitrectomy is another treatment option in this age group but remains controversial. While it can relieve the bacterial burden in the vitreous, these children are often very ill and may not be well enough to undergo the surgical procedure [76]. It may be best advised for those infants who are not as ill systemically, those who may have developed a retinal detachment in the process, those with infections with particularly aggressive organisms, or in cases where the fundus cannot be visualized . Modern 25 gauge vitrectomy instruments may make this challenging surgery marginally safer.
The treatment of fungal endophthalmitis can often be initiated based upon the characteristic white appearance of the retinochoroiditis with overlying white vitreous condensations. This is a rare infection but accounts for many ocular consultations in the NICU in children with systemic involvement. Intravenous therapy with amphotericin B is a common approach, but this medication has relatively poor ocular penetration and can be toxic to the renal system. Less toxic alternative include fluconazole with a usual dose of 200–400 mg/day for Candida species [106]. Intravitreal injection of amphotericin B or voriconazole are a possible adjunctive treatment approach, and vitrectomy may need to be considered if the infection remains unresponsive to treatment [107]. Recommended intravitreal dosage of amphotericin B is 5–10 μg/0.1 mL and Voriconazole 50–100 μg/0.1 mL.
Retinopathy of Prematurity
History of ROP
In 1941, Dr. Paul Chandler encountered the first two cases of retrolental fibroplasia in Boston, Massachusetts. These two cases were the forerunners of the epidemic now referred to as retinopathy of prematurity (ROP) [108]. Between 1942 and 1945, Dr. Theodore L. Terry collected 117 additional cases of ROP and determined that the pathology occurred in premature infants who initially had normal eyes. The changes associated with retrolental fibroplasia occurred a number of weeks after birth [109]. Husband and wife ophthalmologists, William and Ella Owens concluded that postnatal development of the vascular abnormality seen in ROP was caused by neovascularization beginning 2.5–3.5 months after birth [108, 110]. Secondary to these findings, weekly examinations of the interior of the eye soon became routine for ophthalmologists at large research institutions around the world.
Initially, multiple factors were considered in the pathogenesis of ROP including infection, anemia, vitamin deficiency, and oxygen supplementation. In the 1950s, Patz and colleagues reported an association between supplemental oxygen use and the exponential increase of ROP and resulting blindness worldwide [111, 112]. As a result of the Cooperative Study undertaken to determine the effect of oxygen on ROP, neonatal units started limiting the use of oxygen delivered to pre-term newborns and the morbidity and mortality of these infants exponentially increased [113–115]. In the 1970s, advances in medical technology improved the ability to save the most premature and low birth weight infants, and the incidence of ROP again increased exponentially [116]. Over the years, numerous multi-center trials have been performed to study the treatment outcomes and pathogenesis of ROP.
Pathogenesis of ROP
Understanding of the pathogenesis of ROP requires a basic understanding of ocular embryology. The retinal vasculature begins its development around 16 weeks post conception. The vessels begin at the optic nerve and spread circumferentially, reaching the nasal ora serrata around the eighth month of gestation and is completed at the temporal ora serrata at 40 weeks gestation. In full term infants, the retinal vasculature is mature; however, in pre-term infants, the amount of mature retina is highly dependent on how prematurely the infant is born. The risk of normal development of the retinal vasculature depends on the gestational age of the infant: the younger the infant, the higher the risk.
The relationship between ROP and oxygen exposure has been well elucidated over the years. Researchers are now able to explain the relationship of oxygen and ROP on a molecular level. It is known that angiogenesis is controlled by oxygen tension in many tissues; however, the retina appears to be more at risk when a breakdown of oxygen control occurs. The relationship between retinal and choroidal circulations is suspected to be the reason behind this vulnerability [117]. When a premature infant is placed on supplemental oxygen, a rise in oxygen tension occurs, which in turn reduces the level of cytokines, such as vascular endothelial growth factor (VEGF ) and insulin-like growth factor (IGF-1), required for blood vessel formation [118]. Therefore, the formation of normal retinal vasculature stops. Secondary to the inner retina’s interaction with the choroidal circulation, which continuously maintains a high level of oxygen, the retinal vessels constrict and irreversibly close [119]. This is referred to as the hyperoxic stage. The next stage, hypoxia, occurs when the premature infant is taken off supplemental oxygen. Because of the fall in oxygen levels in the retina, anigogenic factors (VEGF and Erythropoietin) are up-regulated and neovascularization occurs [119]. These new vessels may resolve spontaneously with adequate oxygenation; however, in severe cases, contraction of the new vessels places traction on the retina leading to retinal detachment and permanent vision loss.
The angiogenic factors most notable for their role in the pathogenesis of ROP include VEGF and IGF-1. As mentioned previously, VEGF is down regulated during hyperoxia causing retinal blood vessels to become irreversibly obliterated. VEGF is then up-regulated during hypoxia and promotes the formation of new blood vessels that contribute to the pathology seen in ROP [120]. With this knowledge, it is not surprising that anti-VEGF agents, such as bevacizumab, have been used with success in the treatment of ROP [18, 121–123]. This topic is discussed further in the section titled Treatment of ROP.
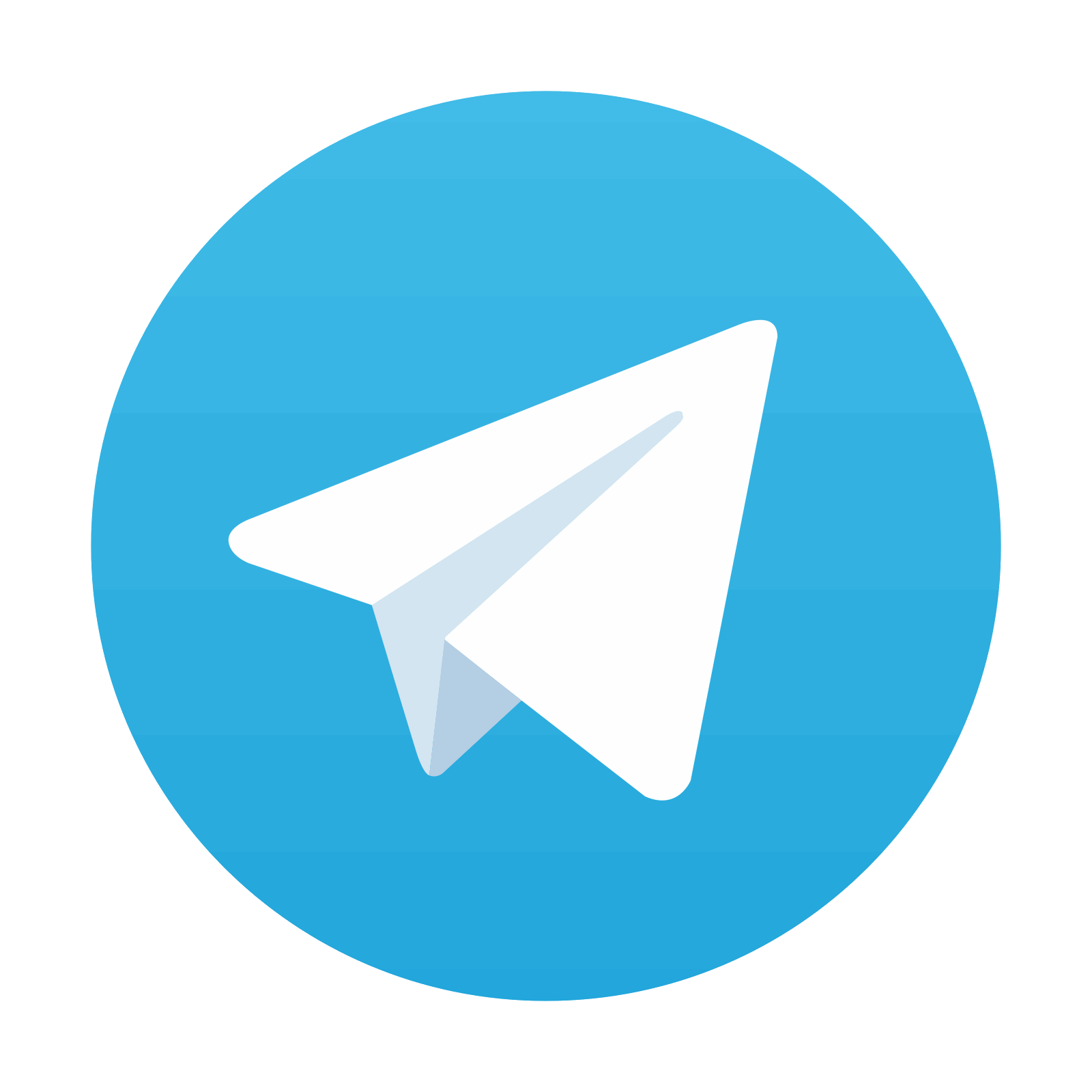
Stay updated, free articles. Join our Telegram channel

Full access? Get Clinical Tree
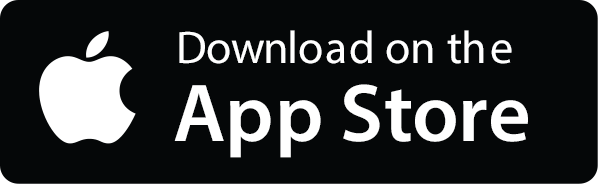
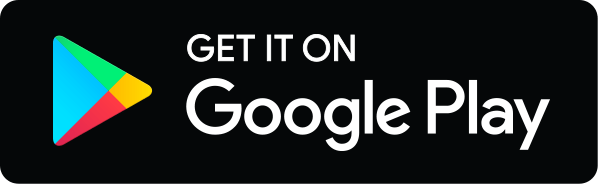