Malformation/hypoplasia
Renal-coloboma syndrome
Wilms tumor-aniridia (WAGR contiguous deletion syndrome)
CHARGE syndrome
Alagille syndrome
Branchio-oto-renal syndrome (BOR)
Fraser syndrome
Ciliopathies
Nephronophthisis (NPHP) syndromes (see Table 18.3) including:
Senior-Løken syndrome
Bardet-Biedl syndrome
Joubert syndrome
Mainzer-Saldino syndrome
Common structural defects
Alport syndrome
Nail-patella syndrome
Pierson syndrome
Williams-Beuren syndrome
Marfan syndrome
Alagille syndrome
IFAP ± BRESHAK syndrome
Zellweger syndrome
Metabolic
Lowe syndrome
Oxalosis
Cystinosis
Lecithin cholesterol acyltransferase (LCAT) deficiency/Norum syndrome
Osteopetrosis with tubular dysfunction
Galactosemia
α-galactosidase A deficiency/Fabry disease
Familial hypercalciuric hypomagnesemia with nephrocalcinosis (FHHNC)
Phakomatosis
von Hippel-Lindau disease
Tuberous sclerosis complex
Vascular
Diabetes mellitus
Hypertension
Inflammatory
Tubulointerstitial nephritis and uveitis syndrome
Sjögren syndrome
Granulomatosis with polyangiitis (formerly Wegener’s granulomatosis)
Systemic lupus erythematosus
The oculorenal disorders exist for a variety of reasons. A systemic anomaly may result in deposition of abnormal material in both the eye and the kidney (e.g., Fabry disease). A second mechanism of disease occurs when there is a similarity between antigens in both the eye and the kidney (e.g., tubulointerstitial nephritis and uveitis syndrome ). Thirdly, systemic effects may injure both the eye and the kidney, as in the case of hypertension. Fourthly, both organs may be affected by a chromosomal abnormality (e.g., Wilms tumor and aniridia ). Fifthly, the renal abnormality may secondarily impact the eye as in the cases of dialysis and kidney transplantation . Lastly, the kidneys and eyes develop at similar times embryologically, and hence, developmental defects may coexist (e.g., Alagille syndrome).
Renal Failure, Dialysis, and Transplantation
Definition
Renal failure is divided into acute kidney injury (AKI) and chronic kidney disease (CKD). AKI is a relatively sudden decline in glomerular filtration rate (GFR) . Etiologies fall into three general categories: (1) pre-renal disease, reflecting inadequate renal perfusion; (2) intrinsic renal disease, including acute tubular necrosis, glomerulonephritis, and interstitial nephritis; and (3) post-renal disease secondary to an obstruction of urinary flow.
Chronic kidney disease signifies irreversibility. Recently revised guidelines define CKD as abnormalities of kidney structure or function present for greater than 3 months with some implication for health. CKD is further staged for those over the age of 2 years by GFR ranging from > 90 mL/min/1.73 m2 to < 15 mL/min/1.73 m2 [5]. End-stage renal disease (ESRD) defines those with GFR < 15 mL/min/1.73 m2 [6]. The caveat made for those less than 2 years old reflects the age at which children’s GFR begins to approximate adult norms. Etiologies for pediatric CKD vary. In younger children the most common causes are congenital anomalies of the kidney and urinary tract (CAKUT), while glomerulonephritides predominate in adolescents [7, 8].
History
While aspects of CKD have been noted since ancient Greek times, the first to associate blindness with renal disease was Bright in 1836. Subsequently, Liebreich was the first to report funduscopic changes associated with uremia in 1859 and named it albuminuric retinitis [9]. The advent of chronic dialysis and renal transplantation in the 1950s has allowed people with renal failure to extend their lives and consequently develop ocular complications that were not previously observed.
Epidemiology
AKI occurs in 33–50 % of acutely ill, hospitalized children while the need for renal replacement therapy (RRT) is almost 25 per 100,000 person-years [10]. With respect to CKD, epidemiologic data is somewhat limited for various reasons, including inconsistent definitions and a relatively asymptomatic early course of the disease [11]. European data reflect an incidence of 11–12 per million of the age-related population for CKD stages 3–5 and 8 per million of the age-related population for CKD stages 4–5 [8]. The mode of RRT varies dependent on the underlying disease process, age (e.g., in the US and Europe, children younger than 14 years tend to obtain peritoneal dialysis, while older adolescents tend to obtain hemodialysis), and location (e.g., Japan and Turkey prefer peritoneal dialysis). Approximately 60–85 % of pediatric patients on RRT undergo kidney transplantation [8].
Systemic Manifestations
Systemic findings of CKD are multi-organ in nature. Fluid retention in the form of edema can be seen in those with a glomerular etiology of CKD. Anemia results from decreased erythropoietin production [12], iron deficiency, and a state of chronic inflammation . Additionally, red blood cell lifespan is shortened in those with CKD further contributing to the anemic state [13]. Metabolic acidosis results from diminished ability to effectively excrete the generated daily acid load [14]. Metabolic bone disease is another common systemic sequel of CKD, resulting from both the impaired renal excretion of phosphorus as well as the decreased synthesis of calcitriol—the active form of vitamin D. The combined effects lead to secondary hyperparathyroidism. Growth failure is a multifactorial manifestation of CKD with average height falling 1.5 standard deviations below the norm [15]. In addition to the aforementioned metabolic bone disease, metabolic acidosis, malnutrition, and a disturbed growth hormone-insulin-like growth factor axis all play a part [11].
The most important systemic features of CKD that impact the eyes are: (1) phosphate retention and decreased 1,25-dihydroxycholecalciferol synthesis with consequent hypocalcemia and secondary hyperparathyroidism; (2) salt and fluid retention resulting in edema and hypertension; and (3) consequences of drug therapy, including immunosuppression and steroid-induced side effects.
Drug therapy may include medications such as steroids , tacrolimus , cyclosporine , mycophenolate mofetil , azathioprine , and antithymocyte globulin . Complications of cyclosporine use include nephrotoxicity, neurologic disorders ranging from tremor to seizure, hirsutism, gingival hyperplasia, hypertension, hyperkalemia, and hepatotoxicity. The principle side effect of azathioprine is suppression of the hematologic system . Antithymocyte globulin , which is a monoclonal antibody to lymphocytes, has many acute side effects including pulmonary edema. None of these treatments, however, have any known direct ocular toxicity. On the other hand, steroids have a broad range of systemic side effects such as adrenal insufficiency, gastric ulceration, impaired glycemic control, mood instability, osteoporosis, elevated intracranial pressure, and hormonal changes to name a few. There are also direct ophthalmic complications, including cataract formation, ocular hypertension and glaucoma, delayed corneal epithelial healing, and reactivation of latent herpetic/fungal infection.
Ophthalmic Manifestations
The effects of ESRD on the eye have been more regularly reported since the 1960s. Symptomatology can vary between mild ocular hyperemia and irritation to significant eye pain and vision loss, depending on the etiology. While difficult to document, there is a belief that maintaining patients in a more normal metabolic balance has decreased the number of ophthalmic complications. The following discussion illustrates the importance of the ophthalmologist in the care of patients with CKD regardless of the modality of support.
Ocular calcifications are usually asymptomatic and typically seen in dialysis patients since it takes time to develop the calcium abnormality. Band keratopathy , similar to that seen in primary hyperparathyroidism, may occur [16, 17]. Brown tumors of the orbit as a manifestation of dysregulation in the calcium-parathyroid axis have also been reported [18, 19]. The frequency of cataracts has been reported to be up to 50 %, and punctate stippling of the lens cortex appears to correlate with hypocalcemia (calcium < 8 mg/dL) [20]. The deposition of calcium phosphate and apatite crystals occurs frequently in the cornea and conjunctiva with reports ranging from 28 to 100 % of the time [21, 22]. One individual has been reported with calcified eyelid margins [23]. Pathologically, the calcium phosphate deposits are seen primarily in the subepithelial and, to a lesser degree, in the basal epithelial layers. The precipitation of calcium phosphate in the conjunctiva may cause acute inflammation and consequent red eye with concomitant, inadequate tearing playing a role [22]. The propensity for calcification in the cornea and conjunctiva is secondary to: (1) alkalinity that results from decreased carbon dioxide concentration from diffusion at the exposed eye surface; (2) the calcium × phosphate product being at least 70 mmol2/L2 in many cases (although some suggest a lack of association with serum calcium and phosphorus levels) [24]; and (3) local degenerative changes [25]. The predisposition to calcium precipitation improves with reduction of the calcium × phosphate product [26–28].
Exudative or serous retinal detachment may occur in renal failure apart from the presence of a retinal hole, tear, or break [29]. The pathogenesis is believed to be related to hyponatremia, osmotic disequilibrium, and hypertension—which may lead to obstruction at the choriocapillaris level and subsequent leakage of fluid into the subretinal space potentially causing secondary retinal detachment [17]. Chorioretinal degeneration is found in approximately 18 % of cases and is manifested by peripapillary, segmental, or triangular patches. Localized retinal pigment epithelial alterations on fluorescein angiography may also be seen [30]. Spontaneous retinal hemorrhages, retinal vascular accidents, macular edema, and papilledema may also occur [21, 31].
The marked fluid shifts and accompanying hypotension that occur with dialysis can also impact the eyes through potential complications such as central retinal vein occlusion, cortical blindness, and anterior ischemic optic neuropathy [32–35]. Optic neuropathy has also been linked to the uremic milieu in several cases [36]. Patients with CKD have an accelerated diffuse vasculopathy that impairs the optic nerve from handling blood pressure fluctuations as seen in dialysis and renal hypertension. This can lead to an increased risk of optic disc ischemia [37]. Irregular macular pigmentation has been described; however, whether it is an actual consequence of dialysis or merely scarring from retinal pigment epithelial changes secondary to prior hypertension and choroidopathy is subject to discussion [38]. Fluorescein angiography (which can be safely utilized in dialysis patients and, in fact, has improved fluorescence in the setting of anemia) demonstrates choroidal sclerotic changes with simultaneous filling of the retinal and choroidal vessels [39]. Some of the signs of hypertension may improve or even resolve if volume status is adequately controlled, but changes associated with arteriosclerosis will persist.
Intraocular pressure (IOP) and intracranial pressure (ICP) tend to rise with dialysis with IOP even increasing by 13.5 mmHg during hemodialysis [40–42]. Since both organs have semipermeable membranes that limit the diffusion of urea, levels of urea will be lowered in most of the body while they are initially maintained in the aqueous humor and cerebrospinal fluid. This osmotic disequilibrium results in water diffusion into the aqueous humor and cerebrospinal fluid, and consequently, the IOP increases [43, 44]. The IOP elevation is more problematic if there is an underlying history of glaucoma. In fact, there is a case report of acute glaucoma precipitated by hemodialysis [45]. However, others have disputed the findings of the above authors and contend that there is an insignificant rise in IOP—or even a decrease in IOP—during dialysis with improved technique and better uremia control [46, 47]. Regardless, this potential problem could be addressed through less rapid urea removal (e.g., lower dialysis clearance rates, increased frequency of dialysis, and less efficient artificial kidneys), use of an alternative osmotic agent (e.g., mannitol), or symptomatic glaucoma treatment with agents other than acetazolamide—which may precipitate metabolic acidosis [17].
Dialysis patients can also be exposed to toxic substances such as desferoxamine through the use of phosphate binders [48]. Desferoxamine has been noted to cause cataracts, pigmentary retinal degeneration, color vision disturbances, nyctalopia, visual field defects, and a predisposition to mucormycosis. These may resolve or chronically remain and have further been reported to occur with one or multiple doses of desferoxamine [33, 49, 50].
In addition to the above, dialysis can be associated with other ocular complications. Conjunctival and retinal hemorrhages are seen in the setting of both CKD and hemodialysis . Their presence may be secondary to uremic bleeding diathesis, heparinization during hemodialysis , or may be coincidental [17]. Unilateral exophthalmos has been reported in one instance related to an occluded arteriovenous fistula and subsequent orbital swelling with retro-orbital edema [51].
Renal transplantation has reportedly stabilized some of the ocular abnormalities that occur with dialysis, including corneal and conjunctival calcification; however, it has been shown to neither reverse nor prevent the progression of diabetic retinopathy [52, 53]. Steroid-related complications are noted elsewhere in this book; however, it needs to be emphasized that glucocorticoid therapy enhances the possibilities of cataract formation and glaucoma [54]. The incidence of posterior subcapsular cataracts has been stated as 17–58 % in the transplant population [54, 55]. The posterior subcapsular cataract of renal transplantation reportedly can be distinguished from age-related posterior subcapsular cataract. The axial thickness of the lens is larger than normal, and the opacity lies in the superficial cortex at a depth proportional to the time from transplant with the lens maintaining a normal anterior clear zone [56]. In several series, 17–19 % of affected individuals ultimately required cataract extraction; a third of those were bilateral procedures [21]. Several authors believe that the cataracts are related to steroid use in the peritransplant period and stabilize or even regress when the steroid doses are reduced [56, 57]. Anterior subcapsular opacities are seen in approximately 18 % of cases [58]. Unlike the posterior cataracts, these subcapsular opacities are non-progressive and may be related to underlying renal failure rather than immunosuppressive treatment. They may also represent glaukomflecken i.e., signs of lens epithelial necrosis secondary to elevated IOP. Alterations in IOP attributable to glucocorticoids have been noted in 4–33 % of patients with 2–30 % of those patients requiring IOP-lowering agents [59]. Patients generally were noted to have increased ocular tension between 2 and 6 months post-transplant with normalization that allowed discontinuation of therapy anywhere from 2 months to 3 years after therapy was initiated. Finally, dendritic ulcerations of the cornea have been reported in approximately 2 % of renal transplant patients [21]. The infections with ocular involvement that are seen post-transplant include cytomegalovirus, herpes simplex keratitis and retinitis, Staphylococcus , Pseudomonas , Candida , Nocardia , coccidiomycosis , aspergillosis , toxoplasmosis , and Pneumocystis [54, 55, 60, 61]. Squamous cell carcinoma of the eyelid, malakoplakia of the eyelid, keratoacanthoma, ocular lymphoma, and reactivation of malignant melanoma have all been reported, as well [62–65].
Diagnosis
Renal failure may be diagnosed under varied circumstances. Signs and symptoms such as high blood pressure, edema, or gross hematuria may prompt an evaluation. Alternatively, the diagnosis may be made incidentally either through routine screenings or when evaluating other conditions. Ultimately, it is the finding of elevated serum creatinine (translating to reduced GFR), abnormal urinalysis, and/or radiographic evaluation that confirms the diagnosis. Once renal failure has been identified, the distinction should be made between acute and chronic conditions .
Management
With regards to AKI, determining the underlying cause (i.e., pre-renal, intrinsic renal, post-renal) can help guide the management both of the etiology as well as secondary effects (e.g., edema, hypertension). A discussion of therapeutic options for the varied etiologies of AKI is beyond the scope of this chapter.
Medical therapy can be utilized to mitigate the systemic effects of CKD. Anemia is managed with iron supplementation and erythropoietin. Metabolic acidosis is treated with systemic bicarbonate therapy. Mineral bone disease requires phosphate control—either through diet alone or with the assistance of phosphate binders, repletion of 25-hydroxyvitamin D, and provision of active vitamin D. Growth failure necessitates adequate calorie intake and management of secondary hyperparathyroidism before consideration of growth hormone therapy.
Renal replacement therapy encompasses both dialysis and transplantation. The decision to pursue dialysis occurs when diet and medical therapy no longer suffice to manage the sequelae of CKD or when symptomatic uremia develops. Typically, this occurs when GFR falls below 15 mL/min/1.73 m2. Chronic dialysis modalities include hemodialysis (HD) and peritoneal dialysis (PD). Regardless of modality, the goals of dialysis are the same: namely, to purify the blood of accumulated toxins (clearance) and to remove excess fluid (ultrafiltration) in order to maintain a state of euvolemia. HD necessitates creation of vascular access (e.g., central venous catheter, arteriovenous graft, or fistula). Heparin is utilized to minimize the risk of blood clotting in the extracorporeal dialyzer. PD requires the placement of an intraperitoneal catheter.
Renal transplantation is the preferred RRT given reduced morbidity and mortality as compared to dialysis. In some instances, pre-emptive transplantation occurs without time spent on dialysis in those with ESRD whom medical therapy suffices. Transplant-related ocular abnormalities are relatively more common in children.
From an ophthalmologic perspective, treatment of underlying kidney disease is the most crucial aspect of management. Complications of steroid and RRT (e.g., cataracts and glaucoma) should be monitored and addressed accordingly with medical and surgical intervention as needed. Furthermore, due to the impact of renal failure and transplant on one’s immune status, ocular infections should be closely evaluated and treated aggressively to avoid potentially serious ocular morbidity.
Alport Syndrome
Definition
Alport syndrome (AS) is a hereditary renal disease that is clinically and genetically heterogeneous with frequent sensorineural hearing loss (SNHL) and occasional ocular findings. It is best defined as a progressive hereditary renal dysfunction with a high risk of progression towards end-stage renal disease (ESRD) .
History
Initially reported in a kindred in 1927 [66], Alport syndrome is characterized by hematuria, proteinuria, renal failure, and progressive deafness with a higher preponderance in males [67]. Prior to Alport’s report, there were multiple other case reports of hereditary nephritis; however, Alport was awarded the eponym for recognizing the association of progressive sensorineural deafness with familial hemorrhagic nephritis. The cause of the syndrome was largely unknown [68] until the discovery of mutations in type IV collagen [69, 70].
Epidemiology
AS is the underlying cause of ESRD in up to 3 % of children and 0.2 % of adults [71]. It appears to have a gene frequency of 1 in 5000 [4] without a racial predilection [72]. At its basis AS is a disease of type IV collagen. Six genes (COL4A1–COL4A6) encode six distinct chains (α1–α6), which arrange to form three major heterotrimers expressed in a variety of tissues [73] (see Table 19.2).
Gene | Chromosome | Chain | Heterotrimers | Expression |
---|---|---|---|---|
COL4A1 | 1 | α1 | α1α1α2 | All BM |
COL4A2 | α2 | |||
COL4A3 | 2 | α3 | α3α4α5 | Glomerular BM |
COL4A4 | α4 | Bowman’s capsule | ||
Distal tubule BM | ||||
Ocular BM | ||||
Cochlear BM | ||||
COL4A5 | X | α5 | α3α4α5 | Glomerular BM |
COL4A6 | α6 | Bowman’s capsule | ||
Distal tubule BM | ||||
Ocular BM | ||||
Cochlear BM | ||||
α5α5α6 | Bowman’s capsule | |||
Distal tubule BM | ||||
Collecting duct BM | ||||
Epidermal BM |
The majority of cases of AS arise from an X-linked inheritance pattern. Though there is inevitable progression of X-linked affected males to ESRD, the rate appears at least somewhat influenced by the type of mutation. Those with large deletions, nonsense mutations, and small mutations altering the reading frame of COL4A5 have a 90 % probability of progression towards ESRD before 30 years of age. This is in contrast to splice-site mutations that confer a 70 % risk and missense mutations that confer a 50 % risk of development of ESRD in the same time frame [74].
The ophthalmologist may assist in prognosticating given that the retinal changes correlate with earlier onset of renal failure. As noted earlier, male gender has a negative impact on renal survival as does increasing proteinuria. The mode of inheritance also plays a role with X-linkage having a median renal survival of 25 years in males versus 51 years in autosomal dominant inheritance patterns [67].
Systemic Manifestations
Though the clinical manifestations of AS vary somewhat depending on the mode of transmission, the classic findings are largely based on males affected by the X-linked mode of inheritance. In early childhood, affected males present with isolate, persistent microscopic hematuria though renal function is usually well preserved. Episodes of gross hematuria can occur, as well—notably in conjunction with respiratory illness [75]. With time, progressive renal dysfunction develops with rising serum creatinine, worsening proteinuria, and hypertension.
The progression to ESRD largely depends on the mode of inheritance. ESRD is unavoidable in males with X-linked AS with 50 % progressing by age 25 and 100 % by age 60 [74]. Females with X-linked AS also have a significant risk of progression with 12 % reaching ESRD by the age of 45 [76]. Both genders with the autosomal recessive inheritance pattern follow a similar course. Autosomal dominant inheritance, however, tends to follow a much slower rate of progression [67].
Auditory defects are present in the majority of those with both X-linked and autosomal recessive disease though better characterized in the X-linked population with 90 % of X-linked males affected by age 40 and only 10 % of X-linked females affected in the same time frame [74, 76]. SNHL may be initially subtle—starting in the second decade of life—but tends to be bilateral, symmetric, and progress with time in a manner proportional to the progression of kidney disease [77]. The nerve deafness is felt to be due to degeneration of the stria vascularis and the hair cells of the organ of Corti [78] that causes inappropriately functioning hair cells, which still allow for generally good speech discrimination [77].
Ophthalmic Manifestations
Ocular abnormalities occur in 15–30 % of patients with AS [75, 79, 80]. The most common findings are a perimacular dot-and-fleck retinopathy (85 %) and anterior lenticonus (25 %) [79] while posterior polymorphous corneal dystrophy is a reportedly rare but possibly often overlooked manifestation [81].
Anterior lenticonus is most typically associated with AS and is bilateral in 75 % of patients with a preponderance in males [4]. It is a highly specific finding and is an almost pathognomonic suggestion of AS [79, 82–84]. Anterior lenticonus may be difficult to diagnose, even by slit-lamp exam (see Fig. 19.1). The lens may be normal at birth [84], while the lenticonus develops during the second decade of life, displaying an oil-droplet reflex on retinoscopy. Electron microscopy and histopathologic examination of lenses with anterior lenticonus has revealed thinning of the lens capsule, decreased number of epithelial cells, bulging of the lens substance, and capsular dehiscences with fibrillar deposition and vacuolation [82, 86]. The defective nature of the α(IV) collagen in AS has been shown to lead to the fragility of the lens capsule, causing progressive weakening, lenticonus, and risk for cataract formation or lens rupture [87–89]. With respect to lens opacities, anterior polar cataracts are more commonly noted, but cases of posterior subcapsular cataracts have been reported. Anterior polar cataracts are not of specific diagnostic value and can be difficult to differentiate from anterior pyramidal cataracts in the setting of lenticonus [4, 83, 84, 90]. Other anterior findings include calcium crystals in the conjunctiva [91], partial atrophy of the iris [92], flat lenses [93], spherophakia [79], angle-closure glaucoma, strabismus [94], antimongoloid palpebral slant [92], and nystagmus [92, 93].


Fig. 19.1
Anterior lenticonus in Alport syndrome [85] (Reprinted from The Hospital for Sick Children’s Atlas of Pediatric Ophthalmology, Levin AV, Wilson TW, Buncic JR: Metabolic. In: Levin AV, Wilson TW, eds, 2007, © Wolters Kluwer, with permission from Wolters Kluwer)
Macular and perimacular lesions are more common than lenticonus—again, affecting males more commonly than females; and, in the context of familial hematuria, are diagnostic for AS [79, 95]. The appearance can range from scattered yellow-white dots and flecks, typically in the temporal macula, to a more consolidated ring in the perifoveal region that causes a duller macular reflex [90, 96] (see Fig. 19.2). Visual acuity is unaffected by the retinal lesions [97]. Electroretinography (ERG) is typically normal; however, some authors have found the ERG to have a reduced b-wave while the electro-oculogram (EOG) tracings are normal [98, 99]. Other posterior segment findings include pseudopapilledema [100], retinal drusen [100], retinal detachment [72, 101], macular hole [102, 103], coloboma [104], abnormal macular reflex, hypopigmentation of the retina, tortuosity of the retinal vessels, peripheral pigmentary alterations [93, 98, 105], vitelliform macular lesions [106], bull’s eye maculopathy [106], and macular degeneration [107].


Fig. 19.2
Maculopathy in Alport syndrome demonstrating circumferential exudates in the perifoveal region and clustered pigmentary migration within the fovea [85] (Reprinted from The Hospital for Sick Children’s Atlas of Pediatric Ophthalmology, Levin AV, Wilson TW, Buncic JR: Metabolic. In: Levin AV, Wilson TW, eds, 2007, © Wolters Kluwer, with permission from Wolters Kluwer)
Given that α(IV) collagen is also present in the cornea, it is of no surprise that Alport-related mutations have resulted in corneal anomalies . The most common association is a posterior polymorphous corneal dystrophy (PPCD or PPMD) where aberrant endothelium grows in an epithelial-like manner with abnormal basement membrane formation [79, 81, 84, 108–110]. One particular gene, TCF8, has been implicated in the development of PPMD and has been found to interact with the promoter region of COL4A3, which encodes the α3 subunit of type IV collagen [111]. Other corneal findings include arcus cornealis [90], fine punctate corneal opacities [92], megalocornea [93], progressive bilateral keratoconus [112], microcornea [79], ring abscesses of the cornea [99], and endothelial pigmentation [98].
Diagnosis
The diagnosis of AS is clinically made in those with glomerular hematuria and a family history of the disease. If family history is absent or unknown, the role of either renal or skin biopsy or molecular genetic testing can be discussed.
At its basis, both glomerular and interstitial abnormalities occur due to a defect in the collagen heterotrimer α3α4α5(IV), which is a major component of the basement membrane in the glomerulus (GBM), cochlea (stria vascularis), and eye (e.g., lens capsule) [113]. The α3(IV)–α5(IV) collagen chains have also been found within the internal limiting membrane (ILM) of the retina and basement membrane of the retinal pigment epithelium (RPE) —accounting for the fundus findings in AS [96]. About 85 % of cases are X-linked mutations in the α5(IV) chain (COL4A5 on Xq22.3) with the majority of the remaining kindreds showing autosomal recessive inheritance from defects in the α3(IV) or α4(IV) chains (COL4A3 or COL4A4 on 2q36.3) [4]. Autosomal dominant inheritance due to heterozygous mutations in COL4A3 or COL4A4 remains less common and tends to be less pathogenic given that some normal α3(IV) or α4(IV) components are still being formed [114].
Renal biopsy can be quite useful in diagnosing X-linked and autosomal recessive AS. The characteristic electron microscopy finding of GBM lamellation (longitudinal splitting of the lamina densa) is pathognomonic for the disease though the percentage of glomeruli affected in males with X-linked AS is largely influenced by age with split basement membrane present more frequently in older versus younger males. This is not so, however, with either gender affected by autosomal recessive AS [115].
Immunofluorescence can help confirm the diagnosis, especially in young patients not yet displaying the characteristic GBM findings [116]. Males with X-linked AS have an absence of staining for α3(IV), α4(IV), and α5(IV) collagen chains throughout the kidney while heterozygote females show an interrupted expression. Both genders with autosomal recessive disease will show an absence of the α3(IV), α4(IV), and α5(IV) collagen chains in the GBM; however, α5(IV) staining in Bowman’s capsule and the distal tubule is maintained [117].
Skin biopsy is a less invasive means of diagnosing X-linked AS given the epidermal expression of α5(IV) collagen chain (but not α3(IV) or α4(IV) chains) [118]. Molecular genetic testing is available to assist in both making the diagnosis of Alport syndrome as well as a means of screening family members at risk [119].
Management
There is no targeted therapy or cure for AS. Therefore, treatment is largely aimed at mitigating the sequelae of CKD. Angiotensin-converting-enzyme (ACE) inhibitors can reduce urinary protein excretion and delay onset of ESRD [120]. In those progressing to ESRD, renal transplantation poses no risk of disease recurrence; however, both X-linked males and both genders with autosomal recessive AS can develop an anti-GBM-like disorder [121–123]. Genetic counseling is important given the hereditary nature of the disease.
The treatment of ocular abnormalities is not warranted historically since the development of significant lenticonus or cataract in the pediatric age is rare; however, reports suggest lensectomy with implantation of an intraocular lens may have a role by the second and third decades of life [124–126]. Certainly, visually significant lens changes should be surgically addressed via pediatric cataract extraction techniques. Subsequent visual rehabilitation with aphakic spectacles, contact lens, or intraocular lens implantation then has merit.
Lowe Syndrome
Definition
Lowe syndrome (LS) an X-linked recessive, multisystem disorder that is characterized by cataracts, mental retardation, and proximal tubule dysfunction, leading to a renal Fanconi-type syndrome [127]. Oculocerebrorenal (OCRL) syndrome is its alternate name owing to the primarily affected organ systems.
History
In 1952, Lowe, Terrey, and MacLachlan described the first three cases [128] of what they termed oculocerebrorenal syndrome. Surprisingly, the original three cases were unrelated despite the currently known genetic inheritance pattern. From 1952 through present day, at least 200 cases of Lowe syndrome have been reported in the literature. It was not until 1992, however, that a genetic basis for Lowe syndrome was discovered as an inborn error of inositol phosphate metabolism due to a mutation in OCRL on Xq25–q26 [129].
Epidemiology
Lowe syndrome is a rare diagnosis with an overall prevalence of 1–10 males in 1,000,000 according to the Lowe Syndrome Association (LSA) ; as of 2000, the LSA noted 190 individuals living with the disease [130]. There does not appear to be a racial predilection. Renal failure usually occurs towards the middle of the third decade [131]. Patients frequently survive into the third and fourth decades of life with the metabolic problems typically decreasing after reaching 5–8 years of age. Given the X-linked nature of inheritance, fathers are not carriers, and there are no reports of affected males fathering children [132]. There have been rare reports of females manifesting the full syndrome [133] likely due to X-autosome translocations. In LS as many as one third of patients represent de novo mutations, while 4.5 % are noted to have germline or somatic mosaicism [130].
Systemic Manifestations
Outside of the ocular and renal manifestations, males affected by LS display central hypotonia at birth. Despite the hypotonia, muscle biopsies reveal no abnormalities [134]. Electromyography (EMG) and nerve conduction studies are also normal. The level of muscle enzymes vary with a report of creatinine kinase, aspartate aminotransferase, and lactate dehydrogenase being elevated [131]. Poor development of the anterior fetal brain is one hypothesis accounting for the hypotonia. Central nervous system abnormalities are pronounced and frequently appear in the second 6 months of life [135]. Cognitive deficits are present with only 25 % of patients displaying borderline to normal IQ, resulting in impaired intellectual function [136]. The cognitive deficits are less severe with early systemic intervention and promptly addressing the ophthalmologic deficits. Maladaptive behaviors, including tantrums, stubbornness, and stereotypic mannerisms, are common [136]. Seizures, including infantile spasms, generalized tonic-clonic seizures, and staring spells, may be present in 33–50 % of patients [137, 138]. Anticonvulsant therapy may be required for major motor seizures.
Patients typically have a characteristic phenotypic appearance consisting of microcephaly, frontal bossing, high forehead, hypotonic facies, and short stature [139]. Linear growth decreases after 1 year [131]. Renal failure, rickets, and specific metabolic abnormalities related to the syndrome all may contribute to explain the short stature. Reported musculoskeletal anomalies include joint hypermobility, tenosynovitis, joint effusions, scoliosis, kyphosis, platyspondyly, subluxed or dislocated hips, and cervical spine abnormalities [140, 141].
Renal abnormalities can appear as early as the neonatal period [142] with the urinary excretion of low molecular weight protein reflecting impaired proximal tubular reabsorption. Over the first few years of life, additional proximal tubular wasting can be seen including aminoaciduria, hypercalciuria, kaliuria, phosphaturia, and renal tubular acidosis [131, 139], leading to metabolic acidosis, hypokalemia, and hypophosphatemia. The tubulopathy that develops over time is not consistent with a typical Fanconi syndrome given the absence of glycosuria [143]. Chronic kidney disease generally occurs, which may progress to end-stage renal disease (ESRD) and dialysis dependency. Glomerular filtration rate progressively decreases with age, making ESRD common by early adulthood [144]. Renal biopsies performed early in the disease (less than 10 years old) reflect interstitial fibrosis and tubular dilatation without glomerular involvement—while those biopsied after 10 years of age display a secondary glomerulosclerosis and a more pronounced interstitial disease [131].
Ophthalmic Manifestations
Pathognomonic ocular findings are among the earliest and most consistent clinical observations in LS. The prominent signs are congenital cataracts and congenital glaucoma. Therefore, LS should be considered in any male infant with both of these presenting signs.
Dense bilateral nuclear or posterior cortical cataracts are present in nearly 100 % of cases of affected males at birth [145]. Microspherophakia is common with lenses that are often malformed and discoid in appearance from reduced anterior-posterior and equatorial dimensions. The cataracts occur in small, thin lenses, which may assist with the differential diagnosis [146]. Further findings include an absence of demarcation between the nucleus and cortex, retention of lens nuclei, and irregularities in the thickness of the lens capsule. The lens changes in LS appear to be caused by a defect in early embryogenesis that involves the primary lens fibers [146, 147] and have even been detected prenatally by fetal ultrasound [148].
Female carriers may have opacities of the lens and can be diagnosed in their teenage years although the absence of lens opacities does not exclude the carrier state. The typical appearance is punctate and plaque-like opacities in wedge-shaped aggregates outside the nucleus. The superficial opacities and subcapsular plaque-like cataracts continue to develop into adulthood (see Fig. 19.3). Posterior lenticonus is seen frequently. Excrescences are seen on the anterior and equatorial aspects of the lens capsule [149, 150]. These characteristic lens changes permit accurate genetic counseling for female carriers in families with LS.


Fig. 19.3
Characteristic lens findings in a female carrier of Lowe syndrome highlighting peripheral, wedge-shaped clusters of punctate and plaque-like subcapsular opacities [85] (Reprinted from The Hospital for Sick Children’s Atlas of Pediatric Ophthalmology, Levin AV, Wilson TW, Buncic JR: Metabolic. In: Levin AV, Wilson TW, eds, 2007, © Wolters Kluwer, with permission from Wolters Kluwer)
Glaucoma with or without buphthalmos occurs in over half of cases by 6 years of age [145] and demonstrate histologic anomalies of the anterior chamber [151]. The anatomically abnormal angle of the anterior chamber in LS is indistinguishable from congenital glaucoma. The iris root inserts high on the trabecular meshwork. Other findings include segmental hypoplasia of the iris dilator muscle with adhesions to the anterior lens surface, anteriorly displaced rudimentary ciliary processes, and fenestrations of the iris blood vessels [151]. Strabismus, amblyopia, and pendular nystagmus have all been reported and are most likely secondary to poor vision.
Another typical finding in LS is the formation of corneal keloids that extend through the full thickness of the cornea. In those patients who are at least 6 years old and who have had successful management of their cataracts and/or glaucoma, corneal keloids are the major cause of visual impairment [152]. The pathogenesis of these keloids is unknown although there is a belief, based on several reports of keloid formation, that amino acids may leak into the cornea after an injury or inflammatory reaction, resulting in exuberant fibrovascular proliferation [153, 154].
Diagnosis
Lowe syndrome should be suspected in any male child born with cataracts [128], especially in the setting of hypotonia. Lowe syndrome is an X-linked recessive disease [128] attributed to mutations in the OCRL gene at Xq.25–26 [155]. Wadelius et al. initially utilized DNA examination to make carrier detection and prenatal diagnosis feasible [150]. OCRL-1, the enzyme product of the gene, is an inositol 5-phosphatase [129]. Diagnosis is made through identification of reduced function (less than 10 %) in inositol polyphosphate 5-phosphatase activity of OCRL-1 in cultured skin fibroblasts [156]. The Lyon hypothesis accounts for the finding of intermediate levels of inositol 5-phosphatase as well as the lens findings in female carriers [157]. Each cell in the lens with the mutated X chromosome causes a punctate or placoid cataract.
Management
Treatment is largely supportive. Renal tubular acidosis is treated with bicarbonate therapy. Supplementation of phosphate and vitamin D are necessary to counteract urinary losses. Renal replacement therapy in the form of dialysis and kidney transplantation is offered to those who progress to ESRD. There is no reported risk of disease recurrence in the transplanted kidney [158, 159]. Speech therapy, physical therapy, and occupational therapy are all disciplines that should be consulted to allow patients to reach their full potential. Additional information on support groups and the disease may be obtained from the Lowe Syndrome Association, PO Box 864346, Plano, Texas, 75086-4346.
Prompt ophthalmologic evaluation and treatment is required to minimize the visual impairment and maximize the potential of these developmentally delayed children. The cataracts are most commonly bilateral and very dense, necessitating early removal. In a series of 14 eyes, Kruger et al. reported cataract surgery at a mean age of 1.25 months [160]. Lensectomy and anterior vitrectomy may be performed via a pars plicata or limbal approach. The former may be beneficial to minimize surgical trauma to the anterior chamber and corneal endothelium given the milieu of goniodysgenesis . One report highlighted intracameral hemorrhage after the removal of an anterior chamber maintainer for a limbal-based lensectomy, which was hypothesized to be from iris vessels in the abnormal angle [161]. However, Kruger et al. noted a limbal approach in their 14 eyes without intraoperative complications [160]. Another consideration in these patients is the commonly associated iris dilator muscle hypoplasia that may require the use of intraoperative iris hooks. The poor mydriasis also makes satisfactory postoperative retinoscopy and funduscopy a challenge. Given the high risk of glaucoma that may be a result of underlying anterior chamber dysgenesis and post-lensectomy environs, primary intraocular lens (IOL) implantation is ill-advised. The axial length growth these glaucomatous eyes undergo make initial IOL power selection difficult [160].
The glaucoma of LS is best treated surgically by either goniotomy or trabeculotomy even though the angle may appear open or not respond to angle surgery in a manner similar to congenital glaucoma [162]. Correction of surgical aphakia, most often bilateral, is best corrected with aphakic spectacles. Furthermore, since inflammation and/or minor trauma may play a role in corneal keloid formation [153], aphakic contact lenses are relatively contraindicated. And because corneal keloids involve the full thickness of the cornea with risk of regrowth, lamellar keratoplasty is not a viable option. Thus, keloid resection should be limited to those cases with significant visual impairment [153].
Strabismus, amblyopia, and nystagmus occur commonly in LS as a result of aphakia, poor vision, and defective binocularity. Standard treatment is appropriate. Aggressive ophthalmologic treatment is warranted since these children do not necessarily have severe mental retardation. Those who are visually rehabilitated do better than children suffering from visual deprivation [146]. However, despite early intervention, nystagmus and poor visual outcomes may still occur with postoperative care limited by difficulty in vision assessment and funduscopic exam. Finally, conscientious perioperative anesthesia is an important aspect of ophthalmologic care as these patients are likely to have multiple ocular surgeries and exams under anesthesia, which necessitate control of their metabolic and electrolyte abnormalities [163].
Tubulointerstitial Nephritis and Uveitis Syndrome
Definition
Tubulointerstitial nephritis and uveitis (TINU) syndrome is a disorder characterized by tubulointerstitial nephritis with uveitis preceding, concurrent to, or following the development of renal disease. As the name suggests, this syndrome comprises the involvement of the renal tubules and interstitium by an acute process and an associated anterior uveitis without evidence of other predisposing conditions [164].
History
The association of uveitis with tubulointerstitial nephritis may occur in a variety of situations including infection, immunologic dysfunction, and drug-related [165, 166]. The first case of TINU syndrome was described by Dobrin et al. in 1975 [167]. They presented two patients who had anterior uveitis, renal failure, bone marrow granulomas, hypergammaglobulinemia , and increased erythrocyte sedimentation rates (ESR) while excluding other known inciting causes. Since their initial report, multiple case reports and series have been published with increased detection due to a better understanding of the presentation and features of this disease .
Epidemiology
The incidence of TINU syndrome is uncertain given its rarity and likely underdiagnosis; however, Rosenbaum [168] previously stated it made up 2 % of his general uveitis clinic. A large review of the world medical literature highlighted 133 cases by 2001 [164], and another review in 2009 found 237 published cases [169]. More recently, there was a mention of at least 250 described cases with more than 60 % of those presenting in children [170]. The disease spans a range of ages from 11 to 59 years with a preponderance of teenage subjects. TINU has been reported in all ethnicities with a particularly high prevalence in Japan where it was found to be the second most common cause of pediatric uveitis after sarcoidosis [171]. Although previously believed to be more common in females [164], a lack of gender predilection may be the case. Recently, Mackensen et al. described 33 patients with TINU syndrome of whom 60 % were male overall and 70 % were male in the subgroup younger than 20 years old [172].
The prognosis is for universal improvement with complete resolution in most cases. Mild proteinuria or pyuria rarely persists. The ophthalmologic manifestations also resolve; however, recurrences of uveitis are frequent for several months.
Systemic Manifestations
The primary sites of involvement are recognizably the eyes and the kidneys. The signs and symptoms of this syndrome, however, suggest a systemic disease. Of 129 identified cases, 53 % presented with fever, 47 % with weight loss, and 44 % with malaise. Additional noteworthy signs and symptoms included anorexia, weakness, myalgias, and headache [164]. In addition to elevated serum creatinine and urinary abnormalities noted below, laboratory studies reflected generalized inflammation, including elevated erythrocyte sedimentation rate (ESR) and anemia [164]. Other laboratory findings of note include several case reports of bone marrow positivity for granulomatous disease [167, 173]. However, more cases reported normal bone marrow [174–178], suggesting the presence of bone marrow granuloma may represent a different, as yet unrecognized, disorder. The differential diagnosis includes a wide range of entities constituting autoimmune/inflammatory disorders (e.g., sarcoidosis, Behçet disease, HLA-B27-related disease, juvenile idiopathic arthritis, Sjögren syndrome , granulomatosis with polyangiitis [formerly Wegener granulomatosis], and systemic lupus erythematosus); infections (e.g., toxoplasmosis, histoplasmosis, syphilis, leptospirosis, tuberculosis, typhoid fever, brucellosis, streptococcal, infectious mononucleosis, and other viral infections); lymphoma; and drug exposure. The entries that have particular relevance to children include streptococcal infections, toxoplasmosis, infectious mononucleosis, other viral infections, and drug-related.
The renal manifestations of TINU syndrome are consistent with acute interstitial nephritis (AIN) as seen in other etiologies. Findings are quite variable, ranging from isolated, asymptomatic urinary abnormalities such as pyuria and white-blood-cell casts to both non-oliguric and oliguric renal failure [179]. The primary site of injury is the renal tubule epithelium with laboratory abnormalities reflective of which tubular segment is damaged [180]. For example, proximal tubular damage is evidenced by aminoaciduria , glycosuria , phosphaturia , renal tubular acidosis , and can encompass a complete Fanconi syndrome [181]. Although the presence of urinary eosinophils had been associated with AIN in the past, it has ultimately been found not to reliably distinguish AIN from other etiologies of AKI [182]. As the clinical picture of AIN is fairly nonspecific, renal biopsy may be warranted to confirm the diagnosis.
Ophthalmic Manifestations
TINU syndrome essentially presents in a manner similar to anterior uveitis of any other etiology. Symptoms include pain, photophobia, redness, and less commonly diminished vision. The uveitis is characteristically bilateral, anterior, and of acute onset. In a large review of 1985 uveitis patients, Mackensen et al. noted that TINU-related uveitis represented a small fraction (1.6 %), but when viewed in the context of sudden-onset bilateral acute anterior uveitis (10 %) and in patients younger than 20 years (32 %), this prevalence was much higher [172]. Some have noted posterior uveitis or panuveitis in TINU [164, 183], while others suggest this is due to spillover disease or secondary posterior involvement from a primarily anterior uveitis [172]. There have been, however, rare reports of associated unilateral neuroretinitis [184], unilateral posterior uveitis with papillitis [185], bilateral vitritis [186], and focal chorioretinitis [187]. Posterior segment involvement may represent atypical presentations along the spectrum of TINU syndrome or may be complications such as with a report of two cases with choroidal neovascularization [188] and a report of one case with retinal pigment epithelium detachments [186].
The timing of ocular inflammation is variable. Onset of uveitis can sometimes precede the renal disease and at other times occur concurrently or several months after renal manifestations. Duration may also be variable from a short course up to a few months to more chronic courses that may be refractory to typical anti-inflammatory therapy. Recurrences are not the norm, but may occur [189]. There does not appear to be a correlation between age of onset and duration of uveitis [172].
Diagnosis
The diagnosis of TINU is made by the presence of uveitis and renal histology consistent with interstitial nephritis. Light microscopy demonstrates interstitial edema with inflammatory cell infiltration predominantly by T cells [190]. Given that the uveitis may not present concurrent to the renal manifestations, a high level of clinical suspicion must be maintained [164]. Noninvasive testing may play a role in the pediatric population. Hettinga et al. looked at a Dutch population of 45 patients with uveitis in TINU syndrome under the age of 23 years and found a good sensitivity and high positive predictive value when assessing for elevated urine β2-microglobulin and serum creatinine in these patients [191].
The pathogenesis of TINU syndrome is currently unknown. Some authors believe it is cell mediated due to the finding of decreased T cells. A cell-mediated mechanism is further supported by the fact that (1) several other types of acute interstitial nephritis are cell mediated; (2) there is a cellular reaction in the kidney while immunofluorescence staining for immunoglobulins has been found to be negative in 18 of 19 cases with TINU; and (3) anterior uveitis is frequently thought to be cell mediated [173, 174]. Other authors, however, believe that the pathogenesis is more likely related to a humoral mechanism based on (1) the increase in serum immunoglobulins seen in most patients; (2) uveitis and interstitial nephritis have occurred in both experimental and clinical diseases [192–194]; and (3) circulating immune complexes have been reported in several cases of TINU syndrome [195].
The basic etiology remains unidentified. Despite this, there has been a strong association noted with specific human leukocyte antigen (HLA) alleles such as HLA-DRB1*01, HLA-DQA1*01, and HLA-DQB1*05, which may prove helpful in diagnosis and better understanding of the pathogenesis of this syndrome in the future [183]. Mackensen et al. also showed a higher incidence of HLA-DRB1*0102 allelic frequency in patients that had bilateral acute anterior uveitis in the setting of TINU syndrome compared to normals , whereas those with isolated tubulointerstitial nephritis did not show that specific HLA subtype [196].
Management
The management of TINU syndrome is best separated into therapy for the kidneys or the eyes since the manifestations in these organ systems may occur at different times and require different treatments. Renal involvement is largely addressed through supportive care. Rarely, patients require renal replacement therapy, while the overall renal outcome is excellent for the majority of patients [164, 197]. Given the lack of randomized control trials to date, the role of systemic steroids remains unclear. Most patients with TINU syndrome who have been reported, however, received moderate amounts of oral prednisone therapy for variable lengths of time to treat the renal manifestations.
The anterior uveitis is generally self-limited and well addressed with standard therapy (e.g., topical ophthalmic steroids and cycloplegics). Generally, those patients that are refractory to local therapy do well with oral corticosteroids [171, 172]. Treatment is directed at minimizing the secondary complications of uveitis and its treatment, namely: glaucoma, cataracts, posterior synechiae, macular edema, and optic disc edema. If a patient persists with anterior uveitis and systemic symptoms, it would be prudent to check serum creatinine and a urinalysis for evidence of renal involvement.
Wilms Tumor
Definition
Wilms tumor (nephroblastoma ) is an embryonal renal malignancy typically arising in children. The principal differential diagnosis is nephroblastomatosis , hydronephrosis , polycystic kidney disease , and neuroblastoma . Wilms tumor (WT) may occur as part of the WAGR continuous deletion syndrome (WT, aniridia, genitourinary anomalies, and retardation).
History
Max Wilms first described nephroblastoma in 1899 [198]. In 1964, Miller reviewed 440 cases of WT and found six patients with aniridia [199]. Miller et al. also described other malformations such as hemihypertrophy in their cohort. DiGeorge and Horley brought the syndrome to the ophthalmic literature in 1967 [200].
Epidemiology
Roughly 7 % of childhood malignancies originate from the kidney, and in those younger than 15 years, the majority are WT [201]. There is a male:female ratio of 0.89:1 [202]. Median age at diagnosis is 38 months though affected females tend to present later, while those with bilateral disease present earlier [203]. Bilateral disease is present in 4–13 % of patients [201]. The prognosis of WT is 90 % 5-year survival in well-differentiated tumors, which constitute 85–90 % of cases [204, 205]. WAGR continuous deletion syndrome has a prevalence of approximately 0.75–1 % in patients with WT [199, 206].
The presence of metastatic disease does not have a major negative impact on prognosis in those with favorable histology; however, anaplastic histology confers a poorer prognosis, especially with more diffuse involvement [207]. Marked atypia or sarcomatous pattern is unfavorable with 25 % cure rate although it only makes up 10–15 % of tumors. See Table 19.3 for information on tumor staging from the National Wilms Tumor Study (NWTS) with associated survival rates [207–210].
Table 19.3
National Wilms Tumor Study (NWTS)-3 staging of Wilms tumors with associated survival rates [208]
Stage | Description | Overall survival (favorable histology/anaplastic histology) (%) |
---|---|---|
I | Limited to one kidney and completely excised with intact renal capsule and no tumor rupture; clean surgical margins | 92–97/83 |
II | Local extension outside kidney (including from previous biopsy or tumor spillage) but completely removed; clean surgical margins | 90–95/81 |
III | Residual non-hematogenous, intra-abdominal involvement (including regional lymph nodes, diffuse peritoneal contamination, extension beyond surgical margins, or not completely resectable) | 86/58–72 |
IV | Hematogenous metastases (e.g., lung, liver, bone, brain) | 82/36–56 |
V | Bilateral renal involvement at diagnosis—each side staged separately as above | –/55 |
Aniridia occurs in 1 % of WT as opposed to the aniridic incidence of 1 in 50,000–100,000 in the general population. Conversely, 25–33 % of aniridic patients will develop WT before age three. The patients affected by both aniridia and WT frequently have other systemic malformations [211]. There are a couple of reported cases with a family history of aniridia in whom WT was not also present [212, 213]. Aniridia is severe if associated with other congenital abnormalities. Patients less than 5 years old with aniridia should be screened with a physical exam and ultrasound of the kidneys every 6 months for the presence of WT [214].
Systemic Manifestations
Wilms tumor classically presents as incidental detection of an asymptomatic abdominal mass, although pain can be present in up to 40 % of cases [201]. In up to 24 % of affected children, hematuria, more commonly microscopic, has been noted, as well [215]. Furthermore, hypertension is a common symptom though the true incidence is difficult to discern due to questionable accuracy of blood pressure determination in some children; one series reported a 63 % incidence [216]. The hypertension may be secondary to tumor production of renin [217], local compression of renal parenchyma by the tumor that causes ischemia, or involvement of the renal artery. Systemic symptoms, including fatigue, fever, and weight loss, are late findings in cases of nephroblastoma.
The nephroblastoma-aniridia patients have severe mental retardation (75 % of cases), craniofacial dysmorphism (75 % of cases), genitourinary abnormalities (66 % of cases), frequent growth retardation, and occasional microcephaly. Hemihypertrophy of the body and urogenital malformations such as cryptorchidism, hypospadias, and pseudohermaphroditism are also seen in association with WT. In conjunction with these abnormalities, WT typically presents earlier.
Ophthalmic Manifestations
Aniridia is a panocular disorder that not only involves the iris, but also the cornea, angle of the anterior chamber, lens, retina, and optic nerve [218]. Aniridia is actually a misnomer since, although a large portion of the iris is missing, the iris root is always present. However, the name is still appropriate since the iris appears to be missing on examination by non-ophthalmologic clinicians (see Fig. 19.4). Absence of a well-formed pupillary aperture can lead to visually significant and symptomatic photophobia, glare, and refractive errors.


Fig. 19.4
Aniridia in a non-dilated patient, which allows for visibility of the entire lens and scattered ciliary processes on retro-illumination [85] (Reprinted from The Hospital for Sick Children’s Atlas of Pediatric Ophthalmology, Levin AV, Wilson TW, Buncic JR: Metabolic. In: Levin AV, Wilson TW, eds, 2007, © Wolters Kluwer, with permission from Wolters Kluwer)
While hypoplasia of the iris is the most notable finding, it is not the major impediment of visual function [219]. A survey of 83 aniridic patients in 2011 showed associated issues with nystagmus (83 %), cataract (71 %), dry eye (53 %), glaucoma (46 %), keratopathy (45 %), foveal hypoplasia (45 %), strabismus (31 %), and retinal disease (5 %) [220]. Diminished vision correlates more closely with the presence of cataracts, glaucoma, foveal hypoplasia, and optic nerve hypoplasia.
Aniridia is often associated with anterior chamber dysgenesis and glaucoma. Corneal development is highly dependent on PAX6 (located on 11p13), and thus, abnormalities such as thinned corneal epithelium with poor cellular adhesion and migration during wound healing, infiltration of goblet cells, impaired limbal stem cells, and absence of Bowman’s layer can lead to aniridia-associated keratopathy [221]. Symptoms can vary from mild to severe based on the level of limbal stem cell insufficiency. Some patients may suffer from significant photophobia, tearing, recurrent erosions and scarring of the cornea, and visual disability [222].
Glaucoma is felt to be an outcome of progressive angle closure within a milieu of abnormal angle development. On gonioscopic exam, broad areas of peripheral anterior synechiae and less commonly anterior rotation of the iris root can be seen [223]. Monitoring via tonometry should be assessed in the context of the increased central corneal thickness that can often be found in aniridic patients [224], highlighting the importance of evaluating the optic nerve, angle, and visual field changes in following these patients.
Lens abnormalities may include ectopia lentis, lens coloboma, and cataract with a notably fragile lens capsule. Reduction in vision can further be compounded by macular and optic nerve hypoplasia. Optic nerve hypoplasia has been found in 10 % of aniridic patients, and foveal hypoplasia is more commonly found in those patients with hypoplastic optic nerves, although it can also be independently observed [225]. Sensory nystagmus often develops as a consequence of the reduced vision [221, 226].
Diagnosis
Evaluation begins with ultrasonography given ease and availability. Associated Doppler interrogation can assess for infiltration into the inferior vena cava (IVC) or renal vein [227]. Commonly, contrast-enhanced computed tomography (CT) is the subsequent imaging study of choice by better delineating the mass as well as sites of metastases. Other than local extension, WT may also metastasize to the lymph nodes, lungs, and liver [228]. Involvement of the bone marrow suggests an alternative diagnosis (e.g., neuroblastoma). Definitive diagnosis is made via histologic confirmation.
Seven percent of patients diagnosed with WT have an associated syndrome such as WAGR, Beckwith-Wiedemann, or Denys-Drash. Therefore, close attention should be paid to any associated anomalies suggestive of syndromic diagnoses [201]. A definite genetic basis has been identified in WAGR continuous deletion syndrome. Hittner et al. found a deletion of the short arm of chromosome 11 (11p13) [229]. This region was later found to contain the transcription factor, Wilms tumor 1 gene (WT1) [230]. Nearby to WT1 in the 11p13 location is the paired box 6 gene (PAX6), which is associated with the majority of aniridia cases and the previously mentioned panocular abnormalities. There have been reports of familial aniridia without WT in which case a single break in 11p13 due to a translocation was hypothesized to cause the isolated aniridia phenotype but a full 11p13 deletion was required for associated WT to occur [212, 213]. Moreover, there have been cases of aniridia with no identified PAX6 mutation, or deletion [221].
Management
Management is largely driven by research protocols. Two varying approaches are taken by the National Wilms Tumor Study (NWTS) group and the International Society of Pediatric Oncology (SIOP) with one distinction being the timing of chemotherapy versus pre-emptive surgical resection at diagnosis [231]. Partial nephrectomy and nephron sparing surgery (NSS) are being utilized in an attempt to lower morbidity, especially in cases of Stage V Wilms tumor, solitary kidneys, or underlying renal dysfunction [232, 233]. As noted above, radiation and chemotherapy are adjunctive treatments to surgery.
Given the background of an abnormally developed eye that has a propensity towards progressive scarring and the potential of further fibrotic changes with intervention, there are potential avenues of management, but the prognosis can be guarded [221, 234]. Children with significant foveal hypoplasia should be rehabilitated with low vision aids. Visually significant cataracts should be extracted, and a pars plana approach is a reasonable option to avoid further damage to limbal stem cells. Given that cataracts are often bilateral, correction with aphakic spectacles or contact lenses is indicated. Furthermore, abnormalities in the lens capsule and iris can make lensectomy challenging and preclude the use of a standard intraocular lens (IOL). This, in addition to the symptomatology of photophobia and glare, which may not sufficiently be addressed with colored contact lenses, corneal tattooing, and artificial irides, has led to the potential use of a black iris diaphragm IOL to manage both the congenital aniridia and surgical aphakia [221, 235, 236].
Management of aniridic glaucoma has been a challenge. Medical and laser therapy have not shown good control while goniotomy/trabeculotomy appears to have a better effect when done prophylactically to angle closure rather as a therapeutic option down the road [237]. Others have advocated trabeculectomy as first-line therapy [238] while tube shunt implantation has also shown evidence of good intraocular pressure control [221]. Despite aggressive intervention—or possibly even due to it—long-term visual prognosis in the setting of aniridic glaucoma is guarded. Risk factors towards poorer visual outcome include familial aniridia, higher intraocular pressure at baseline, and increased number of intraocular surgeries [239].
Keratopathy can be treated with ocular surface lubrication but may require more intense management with limbal stem cell transplants, lamellar keratoplasty, or the use of the Boston keratoprosthesis, which has shown promising results in vision improvement but can have variable outcomes [240–243]. In conclusion, the treatment of WT and aniridia can have successes but requires a multidisciplinary approach.
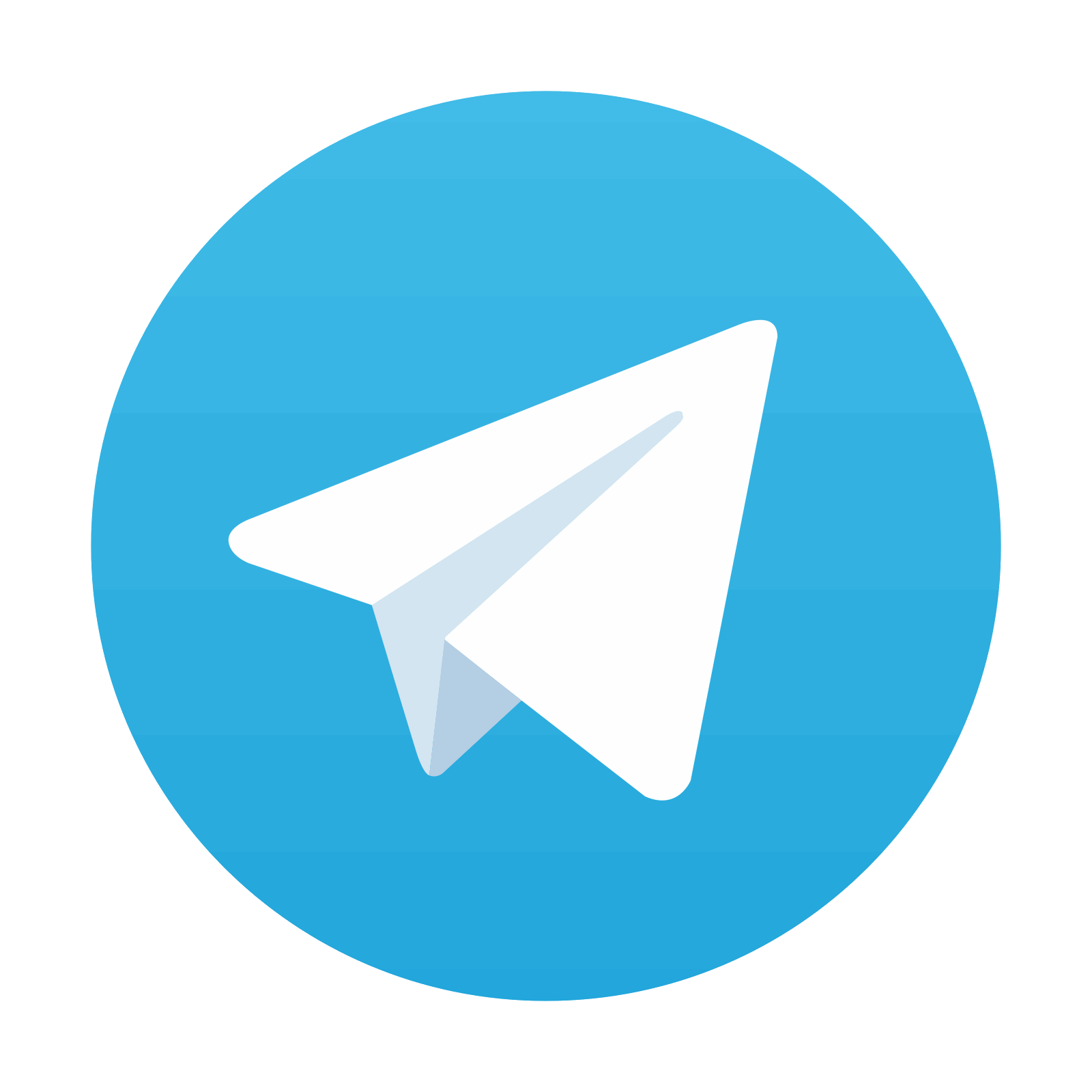
Stay updated, free articles. Join our Telegram channel

Full access? Get Clinical Tree
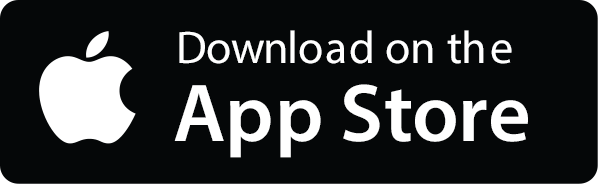
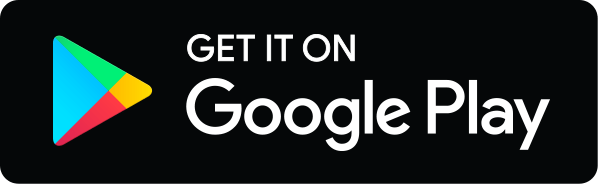