System
Feature
Cardiovascular
Aortic root dilation
Greater propensity to aneurysm rupture
Mitral and tricuspid valve prolapse
Aortic and pulmonary valve insufficiency
Congestive heart failure
Dysrhythmia
Calcification of mitral annulus
Musculoskeletal
Dolichostenomelia
Arachnodactyly
Hindfoot and Forefoot abnormalities
Craniofacial abnormalities
Ligament/tendon laxity
Osteopenia
Spine and chest deformities
Hypotonia
Osteoarthritis
Limb length discrepancy
Muscle wasting
Respiratory
Spontaneous pneumothorax
Restrictive lung disease
Bullae
Central Nervous System
Dural ectasia
Neurodevelopmental abnormalities
Integument
Inguinal hernias
Stria atrophica
In the absence of genetic testing, it has been suggested that the 1996 revision of the Ghent nosology offers greater clinical diagnostic sensitivity and there is still some debate as to which revision should be preferred [9–11]. Despite the high penetrance of FBN1, recent work in patients referred with suspected MFS1 found that around 10 % of patients with a positive genotype failed to be diagnosed by either Ghent nosology [10]. Due to the development of clinical manifestations over time, follow up in this positive genotype group of patients is appropriate [12].
The revised Ghent nosology provides for the exclusion of a diagnosis of MFS1 in the light of genetic mutations consistent with other syndromes [8]. There is an increasing list of mutations which affect TGF-β and fibrillin-1 homeostasis, and consequently produce a constellation of symptoms which is very similar to those observed in MFS1 [4]. These include Loeys-Dietz syndrome, autosomal dominant Weill-Marchesani syndrome and ectopia lentis syndrome [8].
History
MFS was first described in 1875 by Williams at the 11th annual meeting of the American Ophthalmological Society [13], but was more fully realised by a report of a 5 year old girl in 1896 by the French physician Antoine Bernard Jean Marfan [14]. Retrospective review of this particular case indicate that the young girl likely had a mutation of the FBN2 gene consistent with Beals’ congenital contractural arachnodactyly syndrome, but Marfan’s continued reports of similar conditions established his eponym [15].
Epidemiology
MFS1 affects approximately 1 in 4000 people with no obvious propensity for gender or race [1, 16]. This figure may be an underestimate, as mild phenotypes are likely to go undiagnosed. Around 80 % of MFS1 patients have cardiovascular abnormalities which are responsible for the majority of disease specific mortality, with 10 % suffering aortic dissection [3, 16]. However, developments in treatment have greatly improved prognosis and, with modern management, life expectancy is only marginally decreased in MFS1 [17, 18].
Systemic Manifestations
Individuals with MFS1 can have abnormalities of the cardiovascular, respiratory, musculoskeletal and central nervous systems (Table 14.2). It is beyond the scope of this chapter to detail all systemic manifestations, but some attention should be afforded to the key features.
Table 14.2
Non-ocular systemic manifestations of Marfan syndrome
Systemic feature | Score |
---|---|
Wrist and thumb sign (wrist or thumb sign) | 3(1) |
Pectus carinatum deformity (pectus excavatum or chest deformity) | 2(1) |
Hindfoot deformity (plan pes planus) | 2(1) |
Pneumothorax | 2 |
Dural ectasia | 2 |
Protrusio acetabuli | 2 |
Reduced upper/lower segment ratio and increased arm length/height ration in the absence of severe scoliosis | 1 |
Scoliosis or thoracolumbar kyphosis | 1 |
Reduced elbow extension | 1 |
>2 of the following facial features; dolichocephaly, enophthalmos, down-slanting palpebral fissure, malar hypoplasia and retrognathia | 1 |
Skin striae | 1 |
Myopia >3 dioptres | 1 |
Mitral valve prolapse | 1 |
Aortic root dilatation is almost a requirement for diagnosis of MFS1 [8]. Ninety-six percent of patients fulfilling the revised Ghent nosology have an aortic diameter meeting the diagnostic cut off for pathological aortic root dilatation, measured at the level of the sinuses of Valsalva by echocardiography [19]. The diagnostic cut off is drawn at two standard deviations above the mean, which is described as a Z-score [20, 21]. A Z-score >2 means that the patient is in the upper 2.3 % for aortic root diameter in patients of their age, gender and body surface area. There is evidence to suggest that the development of aneurysms, and subsequent dissection, observed in MFS1 is derived from the high availability of TGF-β [6, 22]. TGF-β upregulates MMPs and elastase, which are responsible for the breakdown of elastic fibres which is postulated to lead to a weakening of the aorta [4]. Work in a mouse model of abdominal aortic aneurysms, outside of the context of MFS1, demonstrates a protective role for TGF-β against the progression of aneurysms and so the pathophysiological details of this pathway remain unclear [23].
The musculoskeletal manifestations of MFS1 are multiple and varied, and are often the most overt suggestion of the diagnosis. The most readily identifiable aspect of MFS1 is the excessive growth of long bones (Fig. 14.1) (dolichostenomelia ) and arachnodactyly (Fig. 14.2). With reports of up to 96 % of MFS1 patients complaining of pain in at least one part of the body, these manifestations are an important element of the disease [24]. Scoliosis is one of the key features as it is present in 62 % of MFS1 cases (though only requiring intervention in 12 %) and adult back pain is three times as common with MFS1 than in the general population [24]. The scoliosis observed in MFS1 is particularly challenging as it progresses quicker, responds less well to bracing, and has higher risk surgical solutions than other forms of scoliosis, due to greater risks of bleeding and fixation failure [24]. The pathophysiology of these facets of MFS1 are far from clear, but the effects of TGF-β on the activity of BMPs is likely to play a role [5].



Fig. 14.1
Increased wing span in Marfan syndrome

Fig. 14.2
Marfan syndrome arachnodactyly
Ophthalmic Manifestations
Fibrillin is located in many structures within the eye leading to a great breadth of ocular pathology associated with MFS1 [25, 26]. Fluctuating blurring of vision, headaches and diplopia are among the most common symptoms. On examination tremulousness of the lens and iris (phacodonesis and iridodonesis ) may be noted, as well as visible zonules or lens equator in the pupillary aperture in cases of lens dislocation. A full mydriasis for examination may also be difficult to achieve due to hypoplasia or absence of the pupillary dilator muscle [27]. Glaucoma and retinal detachment are also associated.
Lens
Lens dislocation , or ectopia lentis (EL) , is almost always bilateral in MFS1 though it may be subtle and asymmetrical. The lens may even remain central if the zonular laxity is symmetric or 360° with the only manifestation being increasing myopia and a clinically apparent increase in the anterior-posterior lens thickness with or without phakodenesis. This causes the lens to be smaller but true microspherophakia rarely occurs with MFS1 [28] although it is a feature of autosomal dominant Weill-Marchesani syndrome which is also associated with FBN1 mutations. The pathophysiology is thought to be related to the aberrant fibrillin of the lens zonules and ciliary processes [26, 28]. EL is the most common ocular pathology in MFS1, with reported prevalence ranging from 45 to 87 % of eyes. The most recent of these studies describes the majority of EL in the vertical or horizontal direction (classically superotemporal) whilst the remaining 40 % demonstrated backwards movement [29–31]. Contrary to some teaching, it is important to recognize that the lens may also deviate inferiorly. EL occurs most commonly before 10 years of age [32]. The presence of EL was also shown to correlate with a longer axial length, poorer visual acuity and higher intraocular pressure (IOP), though EL has been associated with lower IOP elsewhere [29, 33]. Cataract is expected earlier and has a higher prevalence amongst MFS1 patients than in the normal population, perhaps due to lens dislocation [31]. Lens notching may occur in the area of greatest zonular laxity, but true ocular coloboma involving the posterior pole or iris, although rare, has been reported in MFS1 and may be linked to increased levels of TGFβ [34, 35]. Complete dislocation of the lens into the anterior chamber is uncommon in Marfan syndrome. Over time, dislocation into the posterior segment may occur.
Refractive Error
A subluxed lens causes myopia and astigmatism until the point where the visual axis becomes aphakic [29]. Patients with MFS1 also have axial myopia. Amblyopia may also occur as a result of EL [36]. Corneal curvature is reduced in MFS1 which can partially compensate for the increased axial length [37, 38]. Consequently, although myopia is the second most common ocular manifestation of MFS1 and >3 diopters of myopia features in the Ghent criteria, it is only found to have a prevalence of approximately 30 % in the MFS1 compared to 11 % in the general population [29, 37, 39]. Corneal astigmatism is also greater in MFS1, and those with EL have greater levels of astigmatism which has been suggested to be due to the presence of dysfunctional connective tissue in the zonules and cornea [38, 40].
Cornea
As well as displaying a lesser curvature the cornea is thinner both centrally and locally in MFS1 [41]. There is conflicting evidence as to whether either of these differences are associated with the presence of EL [38, 41]. Despite occasional case reports no association between MFS1 and keratoconus has been fully established [42].
Retina
Due to greater liquefaction of the vitreous, forces transmitted to the vitreous from a dislocated lens, and a larger globe, MFS1 eyes have a propensity to retinal breaks. Giant retinal tears have been reported to be 600 times more common in MFS1 than in the general population [28]. Retinal detachment has been reported to have prevalence between 5 and 25.6 % in MFS1 with the risk increased by EL, young age, high axial length and lens extraction surgery [28]. Although retinal reattachment in MFS1 used to have a high rate of re-detachment, modern techniques have given the procedure comparable outcomes to when it is performed in the general population [28]. Bilateral detachment is also common, perhaps raising consideration of prophylactic surgery in the fellow eye.
Diagnosis
Due to the variability in the onset of features, and nature of presentation, MFS1 is often challenging to diagnose, and there is substantial intra- and inter-familial phenotype variability. Fortunately, molecular genetic diagnosis has become readily available. FBN1 is located at 15q21.1. The variety of mutations in this very large gene have made mutations relatively difficult to identify [15]. Mutations of the FBN1 gene are also part of various mutation constellations in other connective tissue diseases [4, 8]. Prenatal diagnosis and pre-implantation genetic diagnosis are both possible when the parental mutation is known, although there is controversy over its appropriateness and the relevant legislation varies between countries [46, 47]. A handful of antenatal ultrasound diagnoses have been described in Neonatal Marfan Syndrome , a syndrome similar to, but more severe than, classical MFS1, but this is not a sensitive test [32, 48]. Diagnosis is important to identify the need for regular follow-up to ensure timely intervention, and also for genetic counselling and offering investigation to first degree family. Although isolated EL has been described due to FBN1 mutation, these patients should be screened periodically for the later onset of aortic root dilation [49].
Management
Although management should be through a multidisciplinary approach, in approximately 50 % of cases, the diagnosis of MFS1 is made by an ophthalmologist and so appropriate referral is often their responsibility [28]. Once diagnosed, patients require at least annual ophthalmic review with particular caution to be exercised in young children who are at risk of developing amblyopia . Whether patients should be advised to avoid strenuous exercise and impact sports in the presence of EL remains controversial. Lens dislocation into the anterior chamber is an emergency, as it can cause an acute rise in intraocular pressure. Treatment is by attempted repositioning with maximal pupillary dilatation and posturing, followed by lensectomy to prevent recurrence. The most common intervention necessary is refractive correction, on account of the frequent myopia and astigmatism observed. Refractive corneal surgery is not advised due to the corneal abnormalities often seen. Lensectomy is commonly indicated because of EL where the equator or near-equator of the lens bisects the pupil, anterior lens displacement causes secondary glaucoma, posterior displacement into the vitreous, and cataract formation. The latter usually does not occur until 30–60 years old [28]. Acceptable visual outcomes have been described with all techniques of lens surgery but there is little consensus as to which methodology is superior, and outcomes of both aphakic and pseudophakic procedures seem similar [50–54].
Management also requires input from the specialties of genetics, orthopedics and cardiology [55]. The orthopedic input is required for the surgical stabilization of scoliosis and to treat pectus deformity, which can be on cosmetic grounds but also to relieve restrictive lung disease [55]. Cardiovascular management involves regular echocardiographic screening, on an annual basis, which is crucial due to the unpredictable rate of aneurysm progression. Pediatric cases and those who have a high rate of aortic expansion should be imaged twice annually [3]. Medical management has previously focused on long term beta-blocker or calcium channel blocker therapy to lower blood pressure and subsequent risk of cardiac complications. There is evidence to suggest the value of angiotensin converting enzyme inhibitors and angiotensin receptor blockers, though a randomized controlled trial comparing atenolol and losartan found no significant difference in the rate of aortic dilation over 3 years [56–58]. Any benefit derived from losartan is thought to be due to its inhibition of TGF-β signalling. During potential episodes of bacteremia, antibiotic prophylaxis is recommended in MFS1 patients with documented valve regurgitation, to minimise the risk of endocarditis. This is not required for intraocular surgery. Surgical intervention for cardiac disease may be needed.
Ehlers Danlos Syndrome
Definition
Ehlers Danlos syndrome (EDS) is an inherited heterogeneous group of connective tissue disorders which is characterised by the presence of joint hypermobility, skin extensibility and tissue fragility. There are six types which have been defined by their signs and symptoms. Each type is distinct and does not alter when inherited (Table 14.3) [59].
Table 14.3
Description of the subtypes of Ehlers Danlos Syndrome taken from the British Medical Journal’s ‘Best Practice’ [60]
Type | Clinical signs | Inheritance | Genetic mutation | |
---|---|---|---|---|
Major criteria | Minor criteria | |||
Classic—Type I/II | – Skin hyperextensibility | – Easy bruising | Autosomal | COL5A1 |
– Widened atrophic scarring | – Smooth and velvety skin | Dominant | COL5A2 | |
– Joint hypermobility | – Molluscoid pseudotumours | |||
– Subcutaneous spheroids | ||||
– Muscular hypotonia | ||||
– Complications of joint hypermobility | ||||
– Surgical complication | ||||
– Positive family history | ||||
Hypermobility—Type III | – General joint hypermobility | – Recurring joint dislocations | Autosomal | TNX—B |
– Mild skin involvement | – Chronic joint pain | Dominant | ||
– Positive family history | ||||
Vascular—Type IV | – Excessive bruising | – Acrogeria | Autosomal | COL3A1 |
– Thin translucent skin | – Early onset varicose veins | Dominant | ||
– Arterial, intestinal and uterine | – Hypermobility of small joints | |||
– Fragility which are prone to rupture | – Tendon and muscle rupture | |||
– Characteristic facial appearance | – Carotid cavernous fistula | |||
– Pneumothorax | ||||
– Positive family history—inparticular sudden death | ||||
Kyphoscoliotic—Type VI | – Severe muscular hypotonia at birth | – Tissue fragility, atrophic scars | Autosomal | LH1—PLOD1 |
– Generalised joint laxity | – Easy bruising | Recessive | ||
– Kyphoscoliosis at birth | – Arterial rupture | |||
– Sclera weakness and globe rupture | – Marfans features | |||
– Microcornea | ||||
– Osteopenia | ||||
Arthrochalasia—Type VIIA | – Severe generalised joint hypermobility | – Skin hyper extensibility | Autosomal | COL1A1 |
Type VIIB | – Recurrent subluxations | – Tissue fragility—Atrophic scars | Dominant | COL1A2 |
– Congenital hip dislocations | – Easy bruising | |||
– Muscular hypotonia | ||||
– Kyphoscoliosis | ||||
– Osteopenia | ||||
Dermatosparaxis | – Skin fragility | – Soft doughy skin texture | Autosomal | ADAMTS-2 |
Type VIIC | – Sagging redundant skin | – Premature rupture of membranes | Recessive | |
– Excessive bruising | – Large hernia |
History
Ehlers Danlos syndrome was named by Fredrick Parkes Weber an English physician in 1936 [61]. The name commemorated the work of Danish dermatologist Edvard Ehlers (1863–1937) and French dermatologist Henri-Alexandre Danlos (1844–1912).
Epidemiology
The combined prevalence of all types of Ehlers Danlos syndrome is estimated to be 1 in 5000 [59]. Initially this was thought to be higher however it has become apparent that the disorder is more common than initially hypothesised. Elher Danlos III or hypermobility type is the most prevalent form of the disease. It is important to consider that hypermobility is thought to be present in around 10–30 % of the population, most commonly in young Asian and African females [62]. The classic type is the next most common and the other forms of Ehlers Danlos syndrome are extremely rare. EDS affects both males and females and there does not appear to be an ethnic predisposition .
Systemic Manifestation
Ehlers Danlos syndrome affects the body systemically in multiple ways:
Joint Hypermobility (Common)
This is a feature of all EDS, most notably classic, however it is less obvious in the vascular types. Hypermobility can be assessed on examination utilising the Beighton 9 point score (Table 14.4) or indirectly via the 5 question questionnaire [63, 64]. It is important to also examine joints not included in the Beighton score for further evidence of hypermobility.
Joint Findings | Negative | Unilateral | Bilateral |
---|---|---|---|
Passive dorsiflexion of the fifth finger >90° | 0 | 1 | 2 |
Passive flexion of the thumbs to the forearm | 0 | 1 | 2 |
Hyperextension of the elbows beyond 10° | 0 | 1 | 2 |
Hyperextension of the knee beyond 10° | 0 | 1 | 2 |
Forward flexion of the trunk with knees fully extended and Palms resting on the floor | 0 | 1 | 1 |
Skin
EDS patients typically have extendable skin which is smooth or silky to touch (Fig. 14.3). It is delicate and in some cases described as semi-transparent when vasculature and tendons are visible [65]. Minor trauma can result in splitting of the dermis which most commonly occurs on pressure points such as elbows. Wound healing is often delayed as it involves the laying down of collagen to induce scar (Fig. 14.3) [66]. Scar tissue may also vary over time with stretching and widening frequently occurring due to the lack of collagen. Patient with EDS may also bruise easily.


Fig. 14.3
(a, b) EDS showing skin laxity/skin scars
Pain and Subluxation
EDS patients commonly describe multiple joint aches including the spine. These pains can be induced by light exercise and typically patients do not display the signs of an inflammatory arthropathy with a lack of erythema and visible joint swelling. Even though patients may be in pain the joints commonly move well and retain their hyperflexibility. It is also thought that EDS patients may have a resistance to local anaesthetics in around a two thirds of cases [67].
In children with EDS motor delay can be apparent and children may have delayed walking [68]. Children may not crawl and bottom shuffling maybe seen as a substitute. Once walking has commenced the child’s motor delay typically resolves however chronic pain and tiredness can ensue and children may demand to be carried.
Ophthalmic Manifestations
EDS can affect the supporting connective tissues around the eye resulting in drooping of the lid [72]. Typically this does not extend into true ptosis but maybe an early subtle sign. Patients have also been described as having an anti-mongoloid slant [73]. In this condition the nasal corners of the palpebral fissure are higher than the temporal corners, the opposite of the typical mongoloid slant.
EDS patients can also be described as having a blue sclera. This is due to thinning or transparency of the scleral collagen which exposes the uvea. Their thin sclera also puts patients at significant risk of globe rupture from mild trauma.
Multiple case reports have also described a link between microcornea and EDS [74, 75]. The cornea may also become keratoconic and cases of keratoglobus have also been described [76].
EDS is a disorder of collagen which can therefore result in ectopia lentis, myopia and an increased risk of retinal detachment [77].
Diagnosis
It is import to establish if a patient has risk factors which include a family history of hypermobility or EDS and predisposing genetic mutations.
Classic EDS
This is inherited in an autosomal dominant fashion with mutations commonly found on the COL5A1 and COL5A2 collagen genes [68]. These mutations are positive in around 90 % of patients with a clinical diagnosis of classic EDS. The altered genes encode for collagen type V and mutation can result in a deficiency of this collagen. Disordered collagen fibres can result in ineffective connective tissue partially in the skin and joints.
Hypermobility EDS
This condition results in joints which move further than the expected range for age and sex. The diagnosis of hypermobility EDS is made when a patient has further systemic associations such as gastrointestinal, skin or autonomic issues. It is most commonly autosomal dominant inheritance however the genetic mutation has not been discovered. It is hypothesised that a genetic mutation results in less effective connective tissue.
Vascular EDS
This is considered a more serious form of EDS due to the risk of vessel rupture. It has autosomal dominant inheritance due to a mutation in the COL3A1 gene [78]. This encodes for collagen III and the alteration of the gene results in disordered collagen and ineffective connective tissue. Patients with this condition may exhibit a characteristic facial appearance which includes large eyes, small chin, and lobeless ears. Their skin is commonly translucent and thin with visible veins on the chest and abdomen.
Kyphoscoliosis EDS
This is a rare condition which is autosomal recessive and caused by a mutation in the PLOD1 gene [79]. This results in deficiency of the enzyme lysylhydroxylase and progressive scoliosis. Features also include thin conjunctiva, blue sclera and keratoconus.
Arthrochalasia EDS
This is a rare autosomal dominant condition which effects type I collagen. It occurs due to a mutation in either COL1A1 or COL1A2 resulting in abnormally weak collagen and loose joints which may recurrently subluxate [80].
Dermatosparaxis EDS
This is a rare autosomal recessive condition due to a mutation in the ADAMTS2 gene. It results in a deficiency of collagen and is characterised by fragile, saggy redundant skin [81]. A positive combination of medical history, genetic testing and the Villefrance criteria are highly specific for the diagnosis of EDS.
Management
There is unfortunately no specific treatment for EDS. Symptoms should be managed and treatment provided to reduce the risk of known complications. As the condition is inheritable, genetic counselling should be offered to help prepare and inform families for the future.
Celiprolol, a beta 1 adreniceptor antagonist and a beta 2 adrenoceptor agonist, has been used in an attempt to reduce the risk of arterial dissections in vascular EDS [82]. It is hypothesised that these medications can reduce cardiac stress and hence lower complications. If complications do occur then surgical intervention may well be necessary.
EDS patients are known to have fragile skin with atypical scarring properties. Patients should therefore be encouraged to minimise the risk of trauma to themselves and this may well include wearing protective clothing. It is important to consider the fragility and scarring nature of EDS skin during surgery. Sutures should be placed under minimal tension and it maybe worth considering further deep sutures, or late removal of skin sutures to ensure the wound has completely healed.
Children with delayed motor skills and associated hypotonia may well benefit from physiotherapy. As patients grow older physiotherapy has also been shown to be beneficial [83]. This could be in the form of aquatic therapy to promote muscle development or manual therapies to aid mobilisation through a safe range of motion. Physiotherapist and occupational therapist play an important role in teaching patients how to use and preserve their joints.
Multiple publications have stressed the benefit of ascorbic acid in EDS type IV and VI [69, 84]. It is thought that the ascorbic acid can improve wound healing and reduce bruising in those even without a vitamin C deficiency. The dosage ranges from 1 to 4 g/day of ascorbic acid (vitamin C).
Regarding EDS patients’ eyes it is important to ensure that patients are refracted due to their known myopic predisposition. Myopic patients typically have a longer than average axial length increasing the risk of retinal detachment. It is therefore advisable that EDS patients have a fundal review every year especially if they are symptomatic with flashes and floaters, to rule out retinal detachment. Parents of children with EDS should be warned about the increased risk of globe rupture and the risk associated with contact sports especially if the sclera is blue.
Osteogenesis Imperfecta
Definition
OI is a condition which is often referred to as brittle bone disease. It is an inherited disorder of connective tissue characterised by bones that break easily.
History
OI has been known my multiple names over the years. In 1835 Jean Lobstein described the disease and called it osteopsathyrosis idiopathica [85]. In 1849 Vrolik expanded upon this describing the congenital form of the disease and naming it osteogenesis imperfect [86]. In 1906 Looser concluded through histological analysis that both Lobstein and Vrolik had described the same condition with different severity [87]. Currently OI is differentiated into four types and this was proposed by Sillence in 1979 [88].
Epidemiology
Studies have shown that the incidence of osteogenesis imperfecta is approximately 1 in 20,000 [89]. It must be recognised that the condition is under diagnosed and prevalence may therefore be higher. Frequency is typically similar amongst ethnic groups except for the Shona and Ndebele tribes in Zimbabwe where an increased rate has been observed [90]. The condition appears to affect males and females equally.
Systemic Manifestations
OI was initially classified by Sillence in 1979. He based his types on familial history, clinical presentation as well as radiological findings. His classification was later modified by Glorieux and Rauch who utilised advancements in genetics to add further details and types to OI as a diagnosis [91].
Type I
This is the most common form of OI which has autosomal dominant inheritance and accounts for around 50 % of all of cases [92]. It typically presents at preschool age with mild bone fragility resulting in fractures and minimal limb deformities. There is great variety amongst presentations and some children may only have minimal limb deformities with few fractures whereas others may illicit multiple long bone fractures with chronic pain. The systemic effects occur due to collagen which is of sufficient quality but not quantity. This is due to a lack of COL1A1 allele resulting in loose joints, poor muscle tone, frequent fractures and blue sclera [93].
Type I can be further subdivided into type IA or type IB based on absence or presence of dentiogenesis imperfecta which is opalescent teeth which are absent in type IA but present in IB. After growth is complete the incidence of fractures significantly reduces. Type I OI typically does not significantly affect height and children are commonly within the normal range for their age.
There is a known association with hearing loss and patients typically become symptomatic as young adults [94]. The diagnosis of OI type I can be a significant burden for patients. To an observer patients may appear to have normal bone structure however the patient may struggle with the psychological burden and stress associated with accommodating their increased bone fragility. Parents of children with OI type I are encouraged to carry documentation of the diagnosis to ensure that fractures are not mistaken for non-accidental injuries.
Type II
This is known as the most severe form of OI [95]. The condition is often lethal in the perinatal period and is due to an autosomal dominant mutation in COL1A1 and COL1A2 effecting type 1 collagen. Patients born with the condition have very short limbs with small chests, soft skulls and their legs may be in a frog position. These children commonly develop respiratory failure due to poorly developed lungs and intracerebral haemorrhage leading to a life expectancy of under 1.
Type II can be further subsided into A, B and C by the radiological appearance of the long bones and ribs. Patients with IIA have broad ribs associated with multiple fractures and under modelling of the femur. In type IIB patients present with normal or thin ribs with fractures and some under modelling of the femur. In type IIC patients there is variable thickness of the ribs with malformed scapulae and slender twisted long bones [96].
Parents with the type II genetic mutation should be offered genetic counselling with the severity of the condition being clearly explained.
Type III
OI type III is severe in those children who survive the neonatal period. The condition is again due to mutation in the COL1A1 or COL1A2 genes resulting in defective collagen type 1. There is associated bone fragility and the number of fractures present in children can vary widely. Children at birth may have shortened bowed limbs with a small chest and soft calvarium. They frequently suffer respiratory problems with difficulties swallowing [97].
As the child develops there may be multiple long bone fractures with increasing bowing and further deformity. Adults are typically short in stature, under 102 cm which is partly due to scoliosis and vertebral compression fractures. Patient may have a triangular face shape due to overdevelopment of the head and underdevelopment of the facial bone. Dentinogenesis imperfecta is common and patients’ sclera is often blue or grey.
Families should be offered genetic counselling and guidance regarding the monitoring of scoliosis and respiratory function.
Type IV
This form of OI can range between type I and type III in severity. It is again typically autosomal dominant and due to a mutation in the COL1A1 and COL1A2 genes. Bone fragility is again common with most fractures occurring before puberty. Patients are often shorter than average distinguishing them from type 1 and scoliosis and barrel chest are commonly associated. They may have a distinctive triangular face and hearing loss however their sclera is often white or normal in colour [98].
Long bone fracture as well as vertebral compression and ligament laxity may also be present. Type IV like type I can be further subsided into ‘A’ and ‘B’. ‘A’ represents the absence of the dentinogenesis imperfecta and ‘B’ the presence.
Advancements in microscopic studies have allowed for a more detailed review of type IV bone. This has resulted in two further types of OI being added to the Sillence classification. They have been labelled type V and VI and are clinically similar to type IV however there are distinctive patterns to their bone structure [99]. Therefore types V and VI can only be diagnosed through radiographic and bone studies and they do not involve deficits in type 1 collagen.
Type V
Type V has the same clinical features as type IV OI and has autosomal dominant inheritance. It is distinguished by its ‘mesh’ like bone appearance on histology. Patients may have large hypertrophic calluses due to fractures of long bones and calcification of the interosseous membrane at the forearm resulting in restriction of rotation and subsequent dislocation.
As with all OI patients should be offered genetic counselling and women can be screened for hypertrophic callus of the iliac bone.
Type VI
This is an extremely rare form of OI and the inheritance mode is yet to be identified [100]. It results in moderate to severe brittle bones and clinically is similar to type IV except there is an increased fracture rate. It can be distinguished from type IV by its characteristic build-up of osteoid caused by a mineralization defect, identifiable in biopsied bone. Patients’ sclera are generally white and dentinogenesis imperfecta is typically absent.
Type VII
Type VII is recessively inherited and has been localised to chromosome 3p22–24.1 and not the collagen type 1 gene [101]. The mutation occurs in the CRTAP gene which codes for a protein called cartilage associated protein which has a role in bone development. Partial function of CRTAP typically results in moderate symptoms however absence was lethal amongst published cases. Patients suffer with fractures from birth with lower limb deformities, proximal limb shortening (rhizomelia ) and coxa vara is common.
Type VIII
This type of OI is due to a mutation in the LEPRE1 gene resulting in a deficiency of P3H1 [102]. Type VIII patients are similar in appearance to types II or III however their sclera is white. They also are severely growth restricted with reduced skeletal mineralisation.
Ophthalmic Manifestations
OI is a condition which effects collagen. Type 1 collagen is an important structural component of the sclera [103]. OI patients tend to have a thin sclera which results in a blue discolouration due to visualisation of the choroid. The reduced thickness also increases OI patients’ risk of traumatic and spontaneous scleral rupture [104].
Type I OI patients have the most apparent blue sclera. Type III patients also have blue sclera however it commonly fades over time. Type IV patients often have a grey coloured sclera. Type VI, VII and VIII typically have a white sclera.
There have also been published cases showing an association between OI and reduced central corneal thickness with subsequent reduced ocular rigidity [105]. The reduction in central corneal thickness increases the risk of cornea perforation in OI patients. Both corneal and sclera perforations require repair and cyanoacrylate glue can be utilised however a patch graft is thought to provide a more reliable repair [106].
OI patients also develop progressive myopia which can result in staphyloma formation [107]. With this they are also at increased risk of retinal detachment and case reports have also highlighted an increased risk of glaucoma [108].
In children and young adults it is important to consider that patients are at increased risk of vitreous haemorrhage and fracture which may complicate cases of non-accidental injury. Another condition which may present in early adulthood is keratoconus as the cornea relies up collagen to maintain its shape [109].
Diagnosis
OI is primarily diagnosed on its clinical presentation. As the condition is rare it is important to involve a clinician who is familiar with the various presentations. It is often an inheritable condition and it is therefore important to examine family members.
Classic clinical features include frequent fractures, reduced growth, blue sclera, dentinogeneis imperfecta and progressive hearing loss.
Patients should be investigated with imaging looking specifically for Wormian bones which are extra bone which occur within a suture in the cranium [110]. Laboratory tests include genetic testing for mutations and DNA sequencing for COL1A1 and COL1A2. This allows the identification of the collagen type 1 gene mutation responsible for the majority of OI. These genetic tests have sensitivity of around 90 % in patients with OI. In individuals where the genetic tests are negative for both COL1A1 and COL1A2, yet the clinical signs are present for OI, further investigations should be completed. These include genetic tests of other collagen genes such as CRTAP and P3H1 which are responsible for the rarer types of OI. Skin biopsies may also be useful in identifying collagen to analyse.
Management
OI is an inheritable genetic condition which has no cure. Management is therefore multi-disciplinary and focuses upon improving the patients’ symptoms. Surgical correction has been described for joint deformities and frequent fractures must clearly be managed appropriately [111]. Physiotherapy plays an important role in maintaining joint mobility and orthotics can be used to help stabilise lax joints. Some patients with poor mobility will benefit from mobility aids and occupational therapists input.
Over the years our genetic understanding of OI has developed resulting in management focusing on the molecular causes of the condition. Evidence now shows that improving bone mass and strength can reduce the associated risk of fracture in OI patients. Bisphosphonates which are typically used to treat osteoporosis by slowing the loss of bone have been found to be beneficial by reducing the amount of vertebral compressions along with some long bone fractures [112].
It is also important to consider that patients may need treatment of conditions related to their OI. These include hearing aid for reduced vision, dental work and in some cases oxygen administration for associated respiratory problems.
Ocular Treatments
OI patients are at increased risk of myopia and therefore patient should be refracted every year and any errors corrected. It is important to consider the known association with keratoconus during optician visits. If there is progressive irregular astigmatism then the patient should be referred to a corneal specialist for consideration of collagen cross linking.
Patients may also require scleral or cornea repairs due to their increased risk of perforation.
When completing any ocular surgery on OI patients it is important to consider that their abnormal collagen results in less forgiving tissue. Scleral perforation during strabismus surgery has been published and scleral incisions created by ports utilised during vitreoretinal surgery, due to patients increased risk of retinal detachment, may need to be sutured [113].
Ensure that OI patients also have an ocular pressure check due to the known associated risk of glaucoma. Patients with evidence of glaucoma can be treated as appropriate with medications and surgeries.
Type II Collagenopathies
Definition
Aberrations of the COL2A1 gene, located on the long arm of chromosome 12, cause a spectrum of bone dysplasias with a range of phenotypes and severities frequently exhibiting ocular manifestations [114]. These diseases have often been considered in a spectrum of severity ranging from perinatally lethal conditions such as achondrogenesis , to those causing congenital dwarfism such as spondyloepiphyseal dysplasia congenita (SEDC) to Stickler syndrome type I (STD-1) [115]. Other non-lethal phenotypes include Kniest dysplasia (KND) and spondyloepimetaphyseal dysplasia Strudwick type (SEMD-ST) [115, 116]. In recent years the literature has moved towards considering these pathologies as a spectrum of disease with variable phenotypes, which is much more consistent with their genetic origins [115, 117]. Type 2 collagenopathies are autosomal dominant, though mutations are frequently de novo, and are often characterised by bone abnormalities, vitreoretinal pathology and early onset deafness [115, 117].
Type 2 collagen is a molecule composed of 3 α1(II) chains [118]. COL2A1 codes for procollagen α1(II) [119]. There are 2 alternative splicing isoforms depending on the inclusion or exclusion of exon 2 of the COL2A1 gene during transcription [115, 120]. The longer procollagen α1(II) isoform, known as the IIA isoform, is only found in the vitreous [118]. Therefore mutations in exon 2 will only have ocular manifestations.
Each of these helical polypeptides has glycine in every third amino acid position which is a key contributing factor to collagen’s stability and structure [115]. Though COL2A1 mutations are firmly established as the cause of this group of diseases, the process by which the clinical phenotype is predicted by the exact mutation observed is unclear [117, 121–123]. No mutational hotspots within COL2A1 have been described and there is great heterogeneity amongst the reported mutations [121]. A recent study found that the majority of patients with SEDC or SEMD-ST had a missense mutation causing a glycine substitution in the helical domain [117]. There were however a small number of splicing mutations as well as C-terminal mutations which question the fidelity of this genotype-phenotype relationship [117]. Exon skipping splice mutations and loss of function mutations have been associated with KND and STD-1 respectively, though exceptions to these relationships have also been described [117, 121, 124, 125].
History
The German pediatrician Wilhelm Kniest first reported his eponymous bone dysplasia in 1952 in a 3½ year old girl [126]. Stickler syndrome was characterised by the German born American pediatrician Gunnar Stickler [127]. Spondyloepiphyseal dysplasia congenital was first proposed as a separate diagnosis from Morquio syndrome and Spondyloepiphysial Dysplasia Tarda by German pediatricians Jürgen Spranger and Hans Wiedemann in 1966 with a report of 6 neonatal cases [128]. SMED-ST was first reported by Murdoch and Walker and the name of the patient described was later attributed to the condition, though it has also been known as dappled metaphysis syndrome after its distinctive radiologic appearance [129, 130].
Epidemiology
Systemic Manifestations
Type II collagen accounts for 90 % of the collagens found in adult hyaline cartilage, and its dysfunction consequently has extensive musculoskeletal effects [120]. The physiological development of cartilage is dependent on both the extracellular matrix and the ability of chondrocytes to organize themselves within it. In a histopathological report of KND, the microscopic structure of the articular cartilage was found to be disorganised with cystic areas giving a “Swiss cheese” appearance [133]. Similar cartilaginous disorganisation with growth plate abnormalities was reported in a mouse model, and electron microscopy from both studies revealed a congested endoplasmic reticulum, which has been suggested as a cause for chondrocyte apoptosis [122, 123, 133]. A transgenic mouse model confirmed these findings but also demonstrated a COL2A1 mutation-dependent shortening and disorganization of chondrocyte sensory cilia and disorganization of the collagen II in the extra cellular matrix [134]. These findings suggest that a mutation of COL2A1 leads to dysfunction of both the protein framework in which chondrocytes organise themselves and also the intracellular means by which they navigate that framework.
Whilst there is considerable overlap between the systemic features of the type 2 collagenopathies discussed here we consider them separately (Table 14.5).
System | SEDC/SEMD-ST/KND | STD-1 |
---|---|---|
Skeletal | Short-trunked dwarfism | Normal stature |
Early onset osteoarthritis | Early onset osteoarthritis | |
Delayed endochondral ossification of appendicular skeleton | ||
Respiratory | Neonatal respiratory distress | |
Orofacial | Cleft palate | Micrognathia (Pierre-Robin sequence) |
Flat mid-face | Flat mid-face | |
Bifid uvula (Fig. 14.5) | ||
Cleft palate (Fig. 14.5) | ||
CNS | Paralysis from atlanto-axial instability | Hearing loss |
Infantile hypotonia | ||
Hearing loss |
Stickler Syndrome
Stickler syndrome is distinct from the other type 2 collagenopathies, in that it does not result in short stature. Although STD-1 is derived from abnormalities of COL2A1 and is the most common variant of Stickler syndrome, there are other forms of the disease due to mutations of the genes which encode type 9 or 11 collagen (Table 14.6). These subgroups express similar systemic features to a variable extent with the exception of the ‘ocular only’ type, where mutations occur in exon 2 of COL2A1 which is only expressed in the vitreous [118, 136].
Table 14.6
Distinguishing features and genetic aetiology of the known types of Stickler’s disease as summarised by Snead et al. (2011) [137]
Type | Affected gene | Distinguishing features |
---|---|---|
Type 1 | COL2A1 | Membranous congenital vitreous anomaly, congenital megalophthalmia, deafness, arthropathy, cleft palate |
Type 2 | COL11A1 | Beaded congenital vitreous anomaly, congenital megalophthalmia, deafness, arthropathy, cleft palate |
Type 3 | COL11A2 | Normal vitreous and ocular phenotype, deafness, arthropathy, cleft palate. |
Type 4 | COL9A1 | Recessive inheritance, sensorineural deafness, myopia, vitreoretinopathy, epiphyseal dysplasia |
COL9A2 | ||
Ocular only | COL2A1 exon 2 | Membranous congenital vitreous anomaly (usually), congenital exorbitism. No systemic features |
Other | unknown | Hypoplastic vitreous, deafness, arthropathy, cleft palate |
Deformity of both hard and soft palate can be observed in all forms of Stickler syndrome, though notably cleft lip is not a feature (Fig. 14.5) [137]. Pierre Robin sequence with micrognathia is common. Although not always present, auditory defects are common and can be sensorineural or conductive in nature [138]. Conductive loss is thought to be due to the presence of defective type II collagen in the articular interfaces of the middle ear bones and the tympanic membrane [138, 139]. Expression of COL11A1 and COL11A2 has been described in the developing cochlea and so aberrations of these genes are thought to lead to sensorineural hearing deficits [137]. Recurrent otitis media, more common with the altered facial anatomy which can be observed in Stickler syndrome, characterized by midfacial hypoplasia and the appearance of exorbitism, may also play a role in conductive hearing loss [140]. A range of non-specific musculoskeletal symptoms have also been described and affect more than 80 % of patients , including hypermobility and chronic back and joint pain [141].


Fig. 14.5
(a, b) Stickler bifid uvula/U shaped cleft palate/facies
Spondoepiphyseal Dysplasia Congenita
SEDC presents at birth, or following antenatal screening, with short stature with or without pectus deformity, cleft palate, lumbar lordosis, talipes equinovarus, short neck and hip flexion contracture [132, 142]. Through infancy and early childhood, abnormal gait due to coxa vara and kyphoscoliosis may become apparent. The appearance of these features and their severity helps divide SEDC into mild and severe phenotypes, though such a distinction can only be made around 4 years old [143]. SEDC is also characterised by its radiological features. Axially, a flattening of the vertebral bodies known as platyspondydly is common, along with atlanto-axial instability due to odontoid hypoplasia [132]. The latter of these features can cause spinal cord compression leading to lasting neurological disability or even sudden death and so is important to identify [144]. During intubation it is important to avoid hyperextension. In the appendicular skeleton delayed epiphyseal ossification is the hallmark of SEDC although abnormalities of the metaphyses are also seen [132]. These peri-articular bone abnormalities lead to precocious osteoarthritis. Audiological deficits, as described in Stickler syndrome, are also common [117].
Kniest Dysplasia and Spondyloepimetaphyseal Dysplasia Strudwick Type
These two disorders both exhibit a great degree of overlap with SEDC. In addition to the features shared with SEDC, there are two additional radiological signs which are considered highly diagnostic of KND. Firstly, the long tubular bone metaphyses exhibit exaggerated flaring which creates the impression of a ‘dumbbell’ femur and humerus. Secondly, clefts seen in the coronal plane can be observed in the vertebral bodies [125]. As for SEMD-ST a flocculated appearance of the metaphyses which also exhibit neighbouring regions of osteosclerosis and osteopenia, giving a radiographic ‘dappled’ appearance, are distinguishing features [145].
Ophthalmic Manifestations
COL2A1 is expressed throughout the ocular tissues during development and so the broad anatomical extent of ocular manifestations of the type 2 collagenopathies is to be expected [114, 120]. Due to its greater relative frequency, the ophthalmic manifestations of STD-1 are the best characterised in the literature, although most of the ocular signs are reported in the other type 2 collagenopathies, and mechanistically it seems likely they have a very similar constellation of signs and symptoms [117, 121, 146]. It has been suggested that the most severe ophthalmic manifestations are seen in individuals with a splicing mutation of COL2A1 [117].
Vitreoretinal
Type 2 collagen accounts for 70 % of the total protein content of the vitreous and mutations in the COL2A1 lead it to appear optically empty [146–148]. Vitreous abnormality is one of the key clinical signs suggestive of type 2 collagenopathies, but it also is the key to the differentiation of the aforementioned variants of Stickler disease, and directs genetic investigations to secure the diagnosis [137]. Although not always consistently observed, the nature of the congenitally abnormal vitreous has been suggested to allow for distinction between the two most common variants; STD-1 and STD-2. In STD-1 membranous anomaly of the virteous, also described in other type 2 collagenopathies, represents the visualisation of a retrolental detached posterior hyaloid membrane on slit lamp examination, whilst a beaded vitreous anomaly is more characteristic of STD-2 [137, 146]. Optically empty vitreous spaces may be observed in both STD-1 and STD-2. These anomalies infer a predilection for retinal detachment, with prevalence as high as 70 % and 50 % reported amongst STD-1 and STD-2 respectively, and similar rates have been reported in other type 2 collagenopathies [149–151]. It should also be noted that Stickler syndrome is the most common cause of heritable retinal detachment in childhood [146]. This retinal detachment is often the result of a circumferential separation of the hyaloid membrane near the pars plana. The retina also commonly exhibits thinning, posterior retinal tears, traditional circumferential lattice and perivascular pigmented radial lattice degeneration [146].
Refraction
A moderate or severe congenital myopia was recently reported in 45 % of patients in a report of a large type 2 collagenopathy cohort: for approximately one quarter the myopia was found to be progressive [117]. Myopia has also been suggested to be particularly severe in KND [120]. However, work plotting the association between COL2A1 and high myopia has failed to demonstrate a relationship in Chinese populations suggesting this relationship may be dependent on other factors [114, 152].
Lens
Cataracts have been reported in 24 % of a Stickler syndrome cohort and 16 % in a type 2 collagenopathy cohort mainly consisting of SEDC patients [117, 141]. They are typically described as cortical wedge, fleck or quadrantic lamellar cataracts [146, 153]. They are often visually insignificant. Ectopia lentis is also rarely reported [154].
Glaucoma
Glaucoma has been shown to increase in prevalence with age in Stickler syndrome, with 35 % of patients aged 50–59 reporting glaucoma [141]. Yet congenital and infantile glaucoma are a manifestation of KND [155]. The majority of glaucoma in Stickler syndrome has been noted in the presence of retinal detachment however, so whether the mechanism of raised intraocular pressure relates to the collagen 2 dysfunction or the anatomical alterations from retinal detachment is unclear. The glaucoma of KND is a primary goniodysgenesis.
Diagnosis
Genetic testing is of value to confirm the clinical diagnosis of a type 2 collagenopathy and also may have prognostic value given the developing association between genotype and phenotype [117]. For example, those with splicing errors are thought to be at greater risk of retinal detachment and hearing loss [117]. Differentiation between bone dysplasias is still largely ascertained on clinical and radiological grounds. In cases of suspected type 2 collagenopathy a complete skeletal survey should be performed [156]. The presence of platyspondyly with abnormal epiphyses in the extremities is consistent with SEDC and KND, whilst the addition of metaphyseal involvement is consistent with SEMD [156]. Antenatal radiological diagnosis of skeletal dysplasias is also possible but DNA testing may be more reliable [157].
Management
Regular multidisciplinary review is the basis for management of these diseases. Ophthalmological survey is key for diagnosis but also for ongoing screening of retinal detachment or risk factors such as peripheral lattice, breaks or tears. Adequate refractive correction must also be ensured. Ocular examination is recommended at birth and annually thereafter until adulthood with an additional early check-up at 6 months of age [117]. Should retinal findings or glaucoma be seen, more frequent follow-up is indicated. Neonatal audiology screening is recommended with regular follow-up until speech development and hearing aids should be considered if indicated. To monitor neurological risk in type 2 collagenopathies other than STD-1, flexion/extension cervical spine films should be performed at age 3 as well as before any anaesthesia requiring procedure throughout life [117]. Some ophthalmologists advise patients to avoid contact sports to minimise retinal detachment risk although this practice is not universal.
Prophylactic 360o retinal laser or cryotherapy just posterior to the ora serrata has been suggested to be effective in preventing anterior retinal detachment in STD-1 [149, 158]. Surgical interventions for atlanto-axial instability may also be appropriate; the most common approach in SEDC is decompression and subsequent fusion around the craniovertebral juncture [159].
Sclerosteosis
Definition
Sclerosteosis is a rare autosomal recessive bone sclerosing disorder characterised by tall stature, increased bone density and entrapment of cranial nerves caused by excessive bone growth [160]. It is caused by a mutation of the SOST gene located on 17q, which is responsible for the protein sclerostin , largely produced by osteocytes [161]. A phenotypically similar disorder, known as sclerosteosis 2, has also been reported as a result of heterozygous and homozygous mutation on 11p in the gene which codes for low density lipoprotein-related protein 4 (LRP4), a sclerostin interaction partner [162]. The mutation observed in sclerosteosis leads to an undetectable plasma sclereostin level [161]. The physiological role of sclerostin is to reduce deposition of bone by osteoblasts and osteoclasts [161].
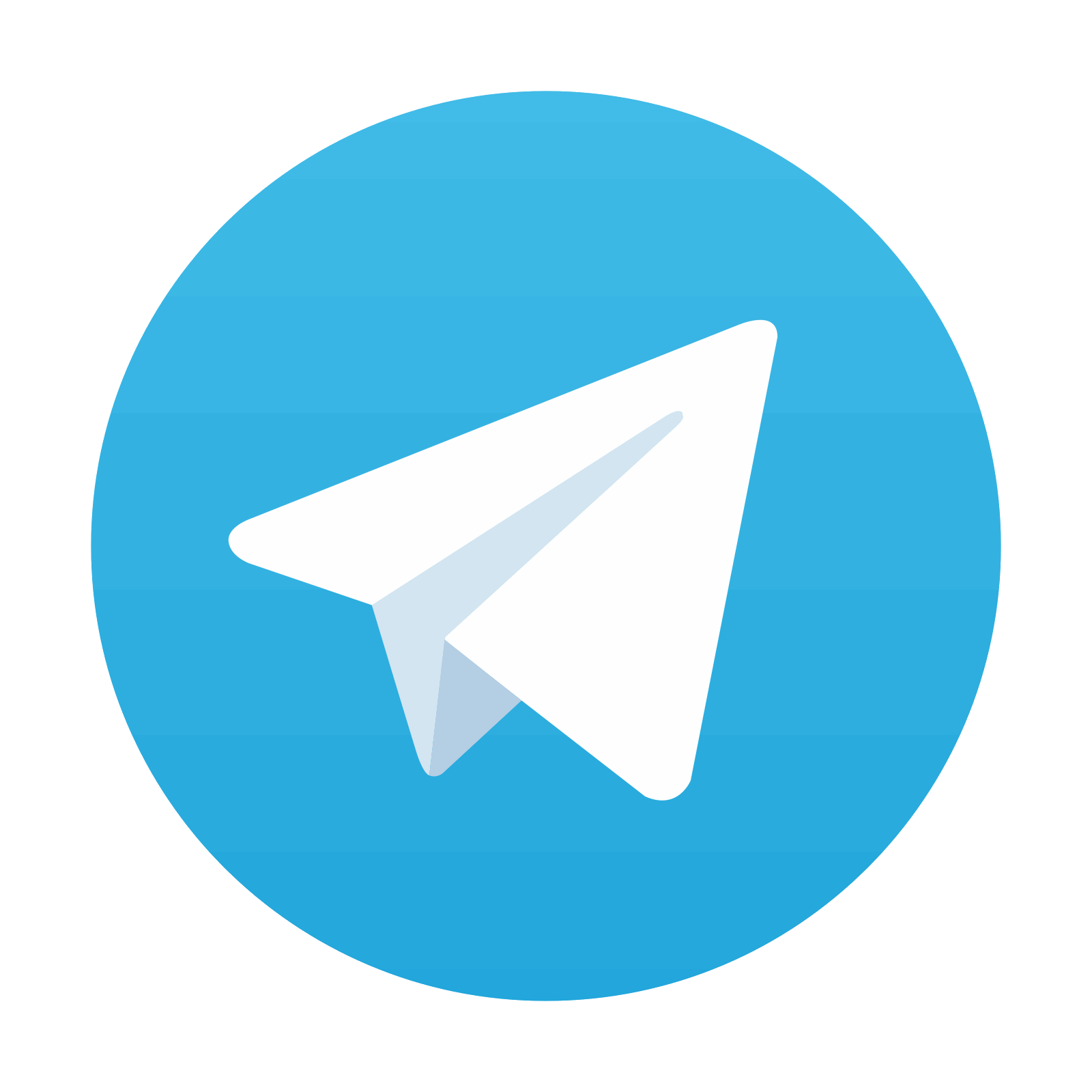
Stay updated, free articles. Join our Telegram channel

Full access? Get Clinical Tree
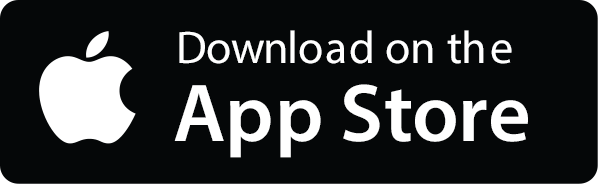
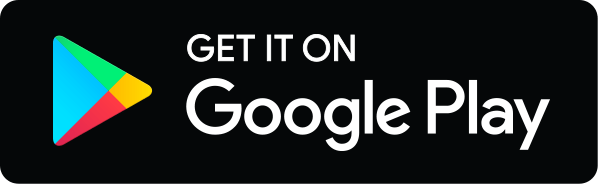