Fig. 23.1
Anatomy of the external nose
The diameter of the valve influences directly the velocity of the airflow. On gentle inspiration, the nasal valve is usually patent. During deep inspiration (exercises or sniffing), the airflow could create a Bernoulli’s effect, which accelerates the flow in this narrow cleft and decreases the pressure on each side of the nasal vestibule leading to the collapse of the nasal wing. Patients suffering from a valve collapse may experience nasal obstruction even during normal breathing.
The congestion of the nasal mucosa varies physiologically, spontaneously and alternatively from side to side with time. One side is blocked, while the other side is patent. This alternates every 3–7 h in adults, leading to a spontaneous cycle phenomenon called nasal cycle. Surprisingly, thanks to this alternation of resistance on each side, the total nasal resistance remains constant (Kennedy et al. 1988).
The paranasal sinus cavities play also a major role in the physiology of the nose. The sinonasal architecture is organised around the ethmoid bone. The perpendicular plate of the ethmoid articulates medially to the septal cartilage, while the outer wall of the ethmoid, including middle concha, articulates laterally with the vertical plate (ascending process of the frontal bone) of the maxilla. On the lateral nasal wall is the osteomeatal complex (OMC). The OMC comprises the middle turbinate, the uncinate process and the bulla ethmoidalis. In this particular anatomical area drain the secretions from the anterior paranasal cavities such as the anterior ethmoid cells, the frontal sinus and the maxillary sinus. Anatomical variations of the different structures of the OMC have been described in the literature such as concha bullosa, paradoxically bent middle turbinate and medially bent uncinate process. In the past ones believed that these anatomical variations were associated to chronic rhinosinusitis. Now most authors do not consider these variations to be responsible of the pathogenesis of chronic sinusitis by themselves.
23.3 Nose Pathologies
All pathologies causing nasal obstruction can cause or worsen SDB (Rappai et al. 2003). The reasons for nasal obstruction are complex and varied, but the causes can be simplified as nonreversible factors, such as anatomic deformities, and reversible factors, such as mucosal oedema and congestion (Table 23.1).
Table 23.1
Causes of nasal obstruction
Nonreversible | Internal/external valve collapse |
Septal deviation, hematoma, perforation | |
Other malformation of the nasal framework | |
Vestibular synechiae or scars | |
Concha hypertrophy | |
Nasal polyposis, antrochoanal polyp | |
Foreign body, nasal packing | |
Benign tumours: angiofibroma, inverted papilloma | |
Malignant tumours: squamous cell carcinoma, adenocarcinoma, melanoma | |
Meningocele | |
Choanal atresia and other craniofacial anomalies | |
Reversible | Allergic/nonallergic rhinitis: NARES-NANIPER |
Acute or chronic rhinosinusitis with or without polyps | |
Drug-induced or occupational rhinitis | |
Atrophic rhinitis | |
Pregnancy | |
Wegener or other granulomatosis |
23.3.1 Nonreversible Factors
Deformity of the nasal septum and/or the nasal pyramid can obviously be associated with uni- or bilateral persistent nasal obstruction. In case of nasal septum deviation, the patient can complain of a uni- or bilateral nasal obstruction depending on the shape, type and location of the deviation (Mladina et al. 2008). Anterior nasal septum deviation is more commonly responsible of nasal obstruction than posterior septal deviation (Grymer et al. 1997). The patient can also complain of a contralateral nasal obstruction, explained by a compensatory hypertrophy of the mucosa of the inferior turbinate. Nasal collapse is another cause of nasal obstruction that is underrated and underestimated by numerous ENT doctors. Nasal obstruction can be revealed during effort, sport or exercises or can be present in a normal and calm breathing. Patients with previous facial nerve palsy or post-traumatic or postsurgical adhesions developed at the level of the nasal vestibule or the columella can present a unilateral nasal collapse. The diagnosis is made by the Cottle manoeuvre or by an anterior and posterior active rhinomanometry and acoustic rhinometry.
23.3.2 Reversible Factors
Nasal obstruction can be caused by a rhinitis. Allergic rhinitis is a very common condition. Bauchau and Durham reported a high heterogeneity of allergic rhinitis incidence among the different European countries and a maximal incidence in Belgium with 29.5 % of the population (Bauchau and Durham 2004). According to ARIA guidelines, the rhinitis can be intermittent or persistent, mild, moderate or severe (Brozek et al. 2010). Indoor allergens can cause symptoms during sleep such as house dust mites, animal danders or fungi.
NARES (nonallergic rhinitis with eosinophils) is another type of rhinitis; the eosinophils are present in the nasal smears, and the patient dramatically improves when he uses a nasal topical steroids. There is no sensitisation to any aeroallergens. Loss of smell is a common symptom. This disease can be a precursor of a true nasal polyposis.
NANIPER (nonallergic noninfectious perennial rhinitis) was called in the past vasomotor rhinitis. The aetiology is unknown, the treatment often disappointing except for nasal obstruction.
Rhinitis medicamentosa is a typical cause of nasal obstruction in a patient who (mis)uses nasal topical decongestant. With time the patient consumes more and more nasal drops. Typically nasal obstruction increases during the night.
Acute and chronic rhinosinusitis with and without polyps are associated with nasal obstruction. Acute rhinosinusitis gives symptoms for a maximum of 6 weeks, whereas chronic rhinosinusitis is symptomatic for more than 12 weeks (Fokkens et al. 2012). Nasal polyposis affects 9 % of the general population. It can be restricted to the nose and sinuses or be associated with asthma and aspirin intolerance. Major symptoms in nasal polyposis are nasal obstruction and loss of smell. There are different classifications used to categorise the polyps. In the Caucasian population, nasal polyposis is associated with a chronic inflammatory infiltrate rich in eosinophils. Oedema, epithelial shedding, pseudocyst formation and changes in the extracellular matrix are some histological characteristics of the common nasal polyposis.
23.4 Physiopathology of Nose Obstruction and SDB
23.4.1 Starling Resistor Model
Nasal obstruction produces collapsing forces that are manifest downstream in the collapsible pharynx (Georgalas 2011; McNicholas 2008). In the respiratory model based on a Starling resistor, the nose is a key determinant of upper airway resistance (Fig. 23.2) (Farre et al. 2008; Horner 2012). Nasal pressure (P N) is zero (atmosphere reference value) in normal conditions. Nasal resistance (R N) determines the maximum flow (V max) in the downstream collapsible pharynx. In the pharynx, P crit is the critical value of airway pressure leading to complete collapse and stop of airflow. P crit depends on transmural pressure and external pressure applied by respiratory muscles. The maximum airflow is defined by V max = (P N − P crit)/R N. This equation implies that increase in nasal resistance (R N) leads to decrease in upper airway flow (V max). Conversely, increase in nasal pressure (P N) by continuous positive airway pressure (CPAP) device improves upper airway flow (V max) (Gold and Schwartz 1996).
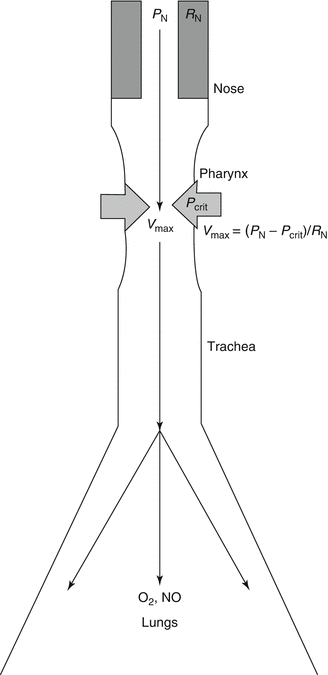
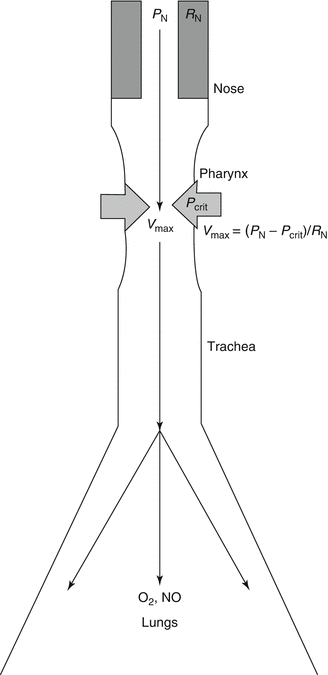
Fig. 23.2
Model of the lungs and upper airway compartments of breathing. The upper airway behaves like a Starling resistor in that obstruction at the inlet produces collapsing forces that are manifest downstream in the collapsible segment, the pharynx. Airflow ceases in the pharynx at a critical value of airway pressure (P crit). Maximum flow (V max) in the pharynx is determined by nasal pressure (P N) and resistance (R N) from the equation V max = (P N − P crit)/R N (Drawing adapted from Ferris et al. (1964))
23.4.2 Oral Breathing
Nasal obstruction may lead to mouth breathing and mouth opening, which, in turn, results in inferior movement of the mandible with associated decrease in pharyngeal diameter. The base of the tongue may also fall backwards reducing the posterior pharyngeal space.
Although the precise mechanisms are not fully understood, oral breathing could be an adaptive response once a particular threshold of nasal airflow resistance is exceeded. Combined recording of oral and nasal breathing during sleep indicates that normal subjects partition flow between nasal and oral routes, with the majority of airflow occurring through the nasal route (Fitzpatrick et al. 2003a). The route of breathing has profound influence on upper airway resistance during sleep. Oral breathing results in an unstable airway and increases total airway resistance. Oscillation of the soft palate, posterior movement of the jaw angle and posterior retraction of the tongue during mouth opening compromise oral-breathing airflow (Georgalas 2011; Fitzpatrick et al. 2003b).
23.4.3 Nasal Receptors
A few studies suggest nasal airflow has a stimulant effect on ventilation, probably via nasal mechanoreceptors maintaining respiratory pacing. Application of local anaesthetics to the nasal mucosa increases the episodes of airway occlusion (McNicholas et al. 1993; White et al. 1985) and impairs the arousal response to airway occlusion (Berry et al. 1995). The parasympathetic nervous system may play a role in the control of breathing and in the hyperpneic responses associated with airflow obstruction. The parasympathetic nervous system component includes neural receptors in the airways as well as afferent and efferent pathways that travel in the vagus nerves (Ko et al. 2008).
23.4.4 Nitric Oxide
Another item playing a major role in snoring and OSA is the nitric oxide (NO). Airborne NO is largely produced in the epithelium of the paranasal sinuses and is involved in the regulation of pulmonary function (Lundberg 2008; Lundberg and Weitzberg 1999). During inspiration through the nose, high levels of NO follow the airstream to the lower airways and the lungs. Nasally derived NO increases arterial oxygen tension and reduces pulmonary vascular resistance. NO enhances therefore blood flow preferentially in well-ventilated areas of the lung, thus optimising ventilation/perfusion matching (Lundberg 1996; Blitzer et al. 1996). In obstructive sleep breathing disease, nasal NO fails partly to reach the lungs, resulting in ventilation/perfusion mismatch (Haight and Djupesland 2003). Lack of NO could also participate in incoordination of pharyngeal and thoracic muscles and in sleep fragmentation. Furthermore, long-term complications of OSA might be due to the repeated temporary dearth of NO in the tissues, secondary to a lack of oxygen (Haight and Djupesland 2003). After their passage to the alveoli in the inspired air, both oxygen and NO are removed by haemoglobin and are transmitted to the tissues. Repetitive hypoxia/reoxygenation adversely impacts endothelial function by promoting oxidative stress and inflammation and reducing NO availability. This vicious spiral mediates the cardiovascular manifestations of OSA (Atkeson and Jelic 2008).
23.5 Craniofacial Development
23.5.1 Morphogenic Perspective
Nose function not only has a direct role in upper and lower airway breathing in adults but also has a long-term impact on the development of the anterior skull base and the maxilla. The influence of nasal patency on the development of the anterior skull base and the maxillary bone has been previously demonstrated in mammals (Paludetti et al. 1995; Scarano et al. 1998). Experimental blockage of rat nostrils resulted after 2–4 months in anatomical changes of the superior maxilla, the skull base and the jaw (Paludetti et al. 1995; Scarano et al. 1998). Nasal obstruction in monkeys resulted in downward and backward rotation of the mandible and changes in dental occlusion (Yamada et al. 1997). Likewise, oral breathing may modify craniofacial growth in children (Peltomaki 2007). Predominant oral breathing during critical growth periods in children could be inscribed in the bones and lead to breathing disorders (Principato 1991). Cephalometric control studies have shown that mouth-breathing children have a higher tendency for clockwise rotation of the growing mandible (Harari et al. 2010). Because of mouth breathing, tongue position in the oral cavity is low, and the balance between forces from the cheeks and tongue is different compared with healthy children. This leads to a lower mandibular position and extended head posture. Malocclusion and skeletal discrepancy may be partially corrected after adenotonsillectomy (Peltomaki 2007). Similarities in cephalometric studies from OSA adults and mouth-breathing children suggest that the apnoeic pattern develops early in the clinical history of patients with OSA (Juliano et al. 2009). However, OSA in children differs that in adults. The involvement of nasal resistance is greater in children, with serious consequences for growth and development (Erler and Paditz 2004). In adults, cephalometric measurements of normal subjects and patients have shown a relationship between OSA and transverse dimensions of nasal cavities, limited laterally by the vertical plates of both maxillae. OSA patients have narrower nasal framework and maxillary bone proportions (Poirrier et al. 2012). Craniofacial features in the pathophysiology of OSA could explain ethnic differences in OSA prevalence and severity for a given level of obesity (Cakirer et al. 2001; Ip et al. 2001).
23.5.2 Phylogenic Perspective
Researchers have speculated that the outer nose may have an evolutionary benefit in human. In addition to an ornamental role for sexual selection, it may play a role in creating a curvilinear airflow pattern (Stupak 2010). During the course of human evolutionary development, the midface is shortened, and the upper airway is narrowed to form a collapsible and distensible tube. This evolution permits the production of spoken language but also results in a predisposition toward upper airway collapse during sleep (Davidson 2003; Davidson et al. 2005; Shprintzen 2003). The development of the human outer nose could be assumed as a compensatory development. The curvilinear airflow pattern provided by the nose adjusts the “angle of attack” of airflow hitting the palate, thus contributing to the pharyngeal opening (Stupak 2010). From this hypothesis, the external nose could provide an evolutionary benefit in the protection against OSA (Fig. 23.3).
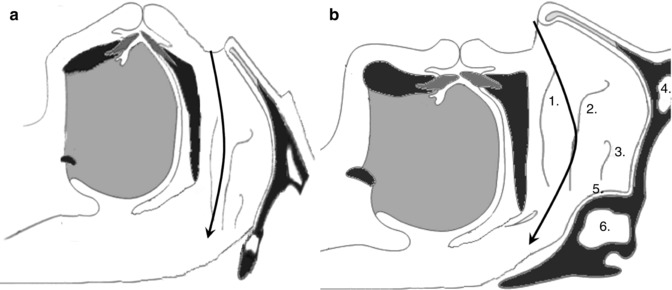
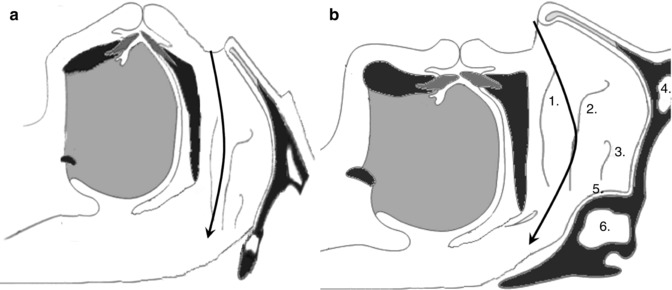
Fig. 23.3
(a) In chimpanzee, the upper airway is larger, which lowers the risk of collapse. The nasal airflow is horizontal (arrow). (b) In normal human, airflow is directed upward through the nasal valve. The outer nose creates a curvilinear airflow pattern (arrow). The latter adjusts the “angle of attack” of airflow hitting the palate, thus contributing to the pharyngeal opening. Anatomy of the internal nose: 1 Inferior turbinate, 2 Middle turbinate, 3 Superior turbinate, 4 Frontal sinus, 5 Spheno-ethmoidal recess, 6 Sphenoid sinus (Drawing adapted from McNicholas et al. (1993) and White et al. (1985))
23.6 Patient Evaluation
23.6.1 Clinical Examination
In case of snoring associated or not to obstructive apnoea, a thorough and complete examination of the nose is mandatory. The nasal pyramid must be evaluated, particularly the dorsum, the lateral cartilages and the columella. A nasal valve collapse must be ruled out by the inspection of the external nose and the Cottle manoeuvre.
Then an anterior rhinoscopy evaluates the nasal septum, the shape and colour of the mucosa of the inferior turbinates and the presence of crust, blood, secretions or polyps (Mladina 1987). Mladina and colleagues defined seven types of septal deviation in a cohort of 2,589 adults. They identified three types with vertical crests, one type with a bilateral deformity, two types with horizontal deformities and another type with atypical deformities (Mladina et al. 2008). Each type may be associated to some degree of nasal obstruction.
Eventually nasal endoscopy must examine the middle meatus, the olfactory cleft and the posterior aspect of the nasal cavity. Nasal polyposis can sometimes be diagnosed with nasal endoscopy only.
23.6.2 Investigations and Functional Testing
Besides the history taking, the patient self-assessment and the anterior rhinoscopy, some investigations must be performed to evaluate the nasal obstruction.
Rhinomanometry and acoustic rhinometry allow for indirect evaluation of nasal anatomy and function (Cole 2000). When these are performed in a supine position, these investigations have more value in assessing nasal breathing of patients with sleep disorders (Virkkula et al. 2003b). Rhinomanometry uses an intranasal closed loop system to measure nasal airway resistance. Acoustic rhinometry uses acoustic reflections to provide information about cross-sectional area and nasal volumes within a given distance. Acoustic rhinometry gives an anatomic description of a nasal passage, whereas rhinomanometry gives a functional measure of the pressure/flow relationships during the respiratory cycle. Both techniques are proposed to assess the efficacy of different treatments and for assessment of the patient prior to nasal surgery. Rhinomanometry and acoustic rhinometry provide “snap-shot” measurements, which may not be representative of a more chronic condition, since nasal turbinate size and function are dynamic processes that may change considerably over a few hours. It is also important to point out that rhinomanometry and acoustic rhinometry tests do not correlate well with a patient’s subjective perception of nasal obstruction. The patient’s subjective perception of the degree of nasal obstruction has been shown to be a more sensitive predictor of positive outcome from medical/surgical management than objective anatomic or physiologic measurements alone. Nasal values measured by acoustic rhinometry and rhinomanometry are correlated inversely with polysomnographic values (apnoea-hypopnoea index, oxygen desaturation index) in nonobese patients (Virkkula et al. 2003a, b; Yahyavi et al. 2008). The association of nasal obstruction measured by posterior rhinomanometry and Mallampati score >3 is predictive of OSA (Liistro et al. 2003). Acoustic rhinometry is also important to measure the nasal valve area (Cakmak et al. 2003).
Nasal inspiratory peak flow gives a measure of bilateral nasal airflow at maximum effort, but does not reflect a physiological measure of nasal airflow. It is however a validated technique to assess the responsiveness of a clinical intervention (Wilson et al. 2003). It should be associated to lung function evaluation as it is influenced by lower airway as well as upper airway function (Nathan et al. 2005).
The levels of NO in the nose can easily be measured noninvasively. NO is altered in several airway disorders, including allergic rhinitis, ciliary dysfunction and sinusitis. The NO value measured is a sum of NO from the sinus via the ostia and the nasal mucosa. NO measurement is mainly valuable for sinonasal disease (Lundberg 2008). Its significance to sleep disorders is currently experimental (Haight and Djupesland 2003).
While they provide objective outcome, the measures of nasal function reflect only one aspect of the disease and may thus not encompass all the other aspects. In recent years, there has been a great expansion in the number and use of quality-of-life questionnaires and other patient-based outcomes in health care. Nasal Obstruction Symptom Evaluation (NOSE) score, Sleep Outcomes Survey (SOS), Visual Analogue Scales (VAS), Sino-Nasal Outcome Test (SNOT) and other surveys have been applied to objectify outcomes in nose and sinus surgery (Lindsay 2012; Hopkins et al. 2009; Piccirillo et al. 2002). Though subjective, they correlate with objective measurements and integrate general health issues, sleep perception and emotional aspects. They include a cluster of interconnected symptoms associated to the nose function. Septorhinoplasty is remarkably effective in improving sleep-related items of the SNOT-22 questionnaire (Poirrier et al. 2013). Beyond the nasal airflow, questionnaires reflect the patient’s perception, suffering and hope. They could help the physician to meet the patient expectations and to provide a reliable follow-up.
Nasal endoscopy and CT scan are two other tools to evaluate the anatomy of the nose and paranasal sinuses. These two examinations are routinely done in all rhinologic work-up.
As obstructive SDB is the consequence of multilevel airway obstruction, nasal evaluation should be integrated with a careful anatomical assessment involving in some cases sleep nasendoscopy, MRI or cephalometry. Lastly, polysomnography remains the gold standard to assess the quality of sleep and to calculate the sleep parameters, including apnoea index, hypopnoea index, apnoea-hypopnoea index, snoring time, amount of REM sleep and sleep latency. Breathing flow can be recorded overnight by means of a thermistor placed at the airway opening (nose and mouth). Inspiratory pressure is indirectly measured by means of chest and abdominal inductance plethysmography belts. Additional devices have been designed to measure nasal pressure (Grover and Pittman 2008) or to record mandible movement (Senny et al. 2012; Maury et al. 2013) during sleep.
23.7 Patient Management
23.7.1 Rationale
The rationale to treat nasal obstruction is to improve nasal patency, re-establishing physiological breathing and minimising oral breathing during sleep. The aim of the treatment is also to reduce nasal resistance and improve the negative intraluminal pressure which generates upper airway collapse. Nasal obstruction can be relieved medically or surgically.
23.7.2 Medical Treatment
Only reversible causes of nasal obstruction can be treated with medications. The commonest causes of inflammation of the mucosa of the upper respiratory tract are allergic rhinitis, acute and chronic rhinosinusitis and nasal polyposis.
23.7.2.1 General Treatment of Allergic Rhinitis
As allergic rhinitis (AR) is the best documented disease, we will focus the following paragraph on it. AR is a very common hereditary health problem. It affects 20–40 million US people, approximately 26 % of the United Kingdom population, 29.6 % of the Belgian population and approximately 10–25 % of the population worldwide (Storms 2008). It is characterised by inflammation of the upper airway mucous membranes mediated by binding of antigens to specific immunoglobulin E (IgE). The patients suffer from nasal symptoms (itching, sneezing, rhinorrhea and nasal congestion), ocular symptoms (red itchy eyes) and headache. AR has a negative impact on the patient’s quality of life. The patient usually suffers from an impairment of the quality of sleep, daytime fatigue, impaired cognitive function and reduced work productivity and performance (Marshall et al. 2000; Wilken et al. 2002; Kessler et al. 2001). AR represents a heavy burden in terms of direct and indirect costs for the patient and the community. There are many drugs on the market to treat it. ARIA proposed some guidelines to use them in a more effective way (Bousquet et al. 2010). H1-antihistamines are certainly the best-known medications to treat AR in adults and children. There are actually two generations of H1-antihistamines: the older ones (the first generation) and the newer ones (the second generation).
First-generation H1-antihistamines are in many countries over-the-counter drugs. A GA(2)LEN position paper recommends to forbid their use over the counter in particular in patients with SDB because they are all sedating and have poor receptor selectivity (Church et al. 2010). They penetrate the blood–brain barrier. Their proclivity to interfere with neurotransmission by histamine at central nervous system H1 receptors potentially leads to drowsiness, sedation, somnolence and fatigue resulting in impairment of cognitive function, memory and psychomotor performance. In addition, the central H1-antihistaminic effects are primarily responsible for the potentially life-threatening toxicity of first-generation H1-antihistamines overdose. They have been implicated in civil aviation, motor vehicle and boating accidents, deaths from accidental or intentional overdosing in infants and young children and suicide in teenagers and adults. Finally, they exacerbate daytime somnolence because they decrease the quality of sleep and reduce rapid eye movement (REM) sleep. Moreover, they have anticholinergic properties, which can cause dry mouth and make mouth breathing even more uncomfortable in the allergic individual with nasal obstruction (Ferguson 2004). The first generation of H1-antihistamines should therefore be avoided in SDB patients.
The second generation of H1-antihistamines is not associated with fatigue, sedation and dizziness even at high dose. They do not change the structure of the sleep because they have more affinity to the H1 receptor, do not pass the blood–brain barrier and do not have anticholinergic properties (Church et al. 2010). The H1-antihistamines are effective drugs: they improve significantly itching, sneezing and rhinorrhea, but they are not so effective on nasal congestion. The recommended indications to prescribe an H1-antihistamine are mild to moderate intermittent AR and mild persistent AR. Azelastine, a topical H1-antihistamine, significantly reduces rhinorrhea and improves subjective sleep, but evidence is lacking on its effects on daytime sleepiness and nasal congestion (Golden et al. 2000).
Topical intranasal glucocorticoids are considered the gold standard for the treatment of all forms of AR. For the most recent molecules, they have a low systemic bioavailability and a high affinity to the receptors. They have a long-lasting effect with minor adverse events. They are active on sneezing, rhinorrhea and nasal congestion. A meta-analysis published in 1998 confirmed the place of the intranasal steroids in the treatment of AR (Weiner et al. 1998). One position paper of the Joint Task Force for the American Academy of Allergy, Asthma and Immunology does not recommend their use over the counter because of the side effects observed in the past with the older generations of topical glucocorticoids (Passalacqua et al. 2000). The plasma concentrations of intranasal fluticasone and mometasone are low due to extensive metabolism and clearance by cytochrome P450 enzyme 3A4. Caution is recommended when co-administered with potent CYTP3A4 inhibitors, especially in HIV population. Current antiretroviral regimens often contain the HIV protease inhibitor ritonavir, and co-administration with topical fluticasone results in a dramatic increase in the latter bioavailability. This may result in iatrogenic Cushing’s syndrome as alerted in increasing number of case reports (Mahlab-Guri et al. 2011; Kedem et al. 2010; Valin et al. 2009; Samaras et al. 2005). Ironically, in patients treated by ritonavir, older generations of topical glucocorticoids appear to be safer options (Foisy et al. 2008). Apart from these particular cases, second-generation topical glucocorticoids (fluticasone, mometasone) remain the first-line and safest treatment for main patients with allergic rhinitis. Intranasal corticosteroids have broad anti-inflammatory activities. They are the most potent long-term pharmacologic treatment of congestion associated with allergic rhinitis and show some congestion relief in rhinosinusitis and nasal polyposis (Table 23.2).
Table 23.2
Effect of medical treatment on sleep-related breathing disorders
Reference | Study | Medication | n | Symptoms improvement | PSG improvement |
---|---|---|---|---|---|
Kerr et al. (1992) | Controlled, prospective | Xylometazoline + nasal dilator | 10 | Yes | No |
Craig et al. (1998) | Controlled, prospective | Fluticasone | 20 | Yes | – |
Hughes et al. (2003) | Controlled, prospective | Budesonide | 22 | Yes | – |
Ratner et al. (2003) | Controlled, prospective | Fluticasone vs. montelukast | 705 | Yes | – |
Craig et al. (2003) | Controlled, prospective | Fluticasone | 32 | Yes | No |
Kiely et al. (2004) | Controlled, prospective | Fluticasone | 24 | Yes | Yes |
Craig et al. (2005) | Controlled, prospective (pooled study) | Fluticasone/budesonide/flunisolide | 42 | Yes | No |
McLean et al. (2005) | Controlled, prospective | Xylometazoline + dilator strip | 10 | No | Yes |
Gurevich et al. (2005) | Controlled, prospective | Budesonide | 26 | Yes | – |
Topical decongestants reduce congestion associated with allergic rhinitis, but because of the risk of rhinitis medicamentosa, they should not be used for prolonged periods. Oral decongestants reduce nasal congestion but may have adverse effects on sleep, even insomnia, because of their stimulatory effects and their association with systemic side effects.
Oral leukotriene receptor antagonists can be of some help in the management of patients unresponsive to the conventional medications. They effectively reduce rhinorrhea, congestion and inflammatory mediators (Ferguson 2004).
Anticholinergic ipratropium bromide is not considered effective in relieving nasal congestion; however, limited data suggest that sleep and quality of life may be minimally improved with this treatment (Rabasseda 2012).
23.7.2.2 Management of SDB in Rhinitis Patients
Patients with perennial allergic rhinitis often present with nasal congestion, poor sleep quality, daytime fatigue and loss of productivity. Pharmacologic therapy that reduces nasal congestion should improve these symptoms. In the literature there are a lot of publications related to the management of allergic rhinitis and the impact on sleep (Table 23.2). These studies often demonstrate positive effects of the medical treatment on the SDB. However, the majority of these papers are based on subjective assessment (disease-specific quality-of-life measures, quality-of-life questionnaires, general questionnaires, Epworth Sleepiness Scale, Pittsburgh Sleep Quality Index, etc.). Only a few studies have objectively assessed sleep (using polysomnography) in allergic rhinitis. In 20 patients with allergic rhinitis and symptoms of daytime sleepiness, flunisolide significantly improved sleep quality and congestion but not daytime sleepiness (Kakumanu et al. 2002). A similar study with fluticasone propionate showed improvement in subjective sleep parameters, but there was no significant change in objective sleep measurements recorded on polysomnography (Craig et al. 2003). On the other hand, Kiely et al. have demonstrated a slight decrease in the AHI in snorers with rhinitis treated with fluticasone propionate compared with placebo (Kiely et al. 2004). Nasal obstruction secondary to allergic inflammation has an impact on sleep quality, and topical corticoid therapy seems to have a positive effect on sleep quality (Rombaux et al. 2005). In one study, 25 patients with seasonal AR and 25 healthy volunteers underwent two consecutive nights of PSG before and during the pollen season (Stuck et al. 2004). There were statistically significant differences between the two groups in sleep parameters, including increases in the apnoea index, hypopnoea index, apnoea-hypopnoea index, snoring time, amount of REM sleep and sleep latency. Nevertheless, the changes were not considered clinically relevant, as values remained within normal limits. Further research involving objective measures is thus still necessary.
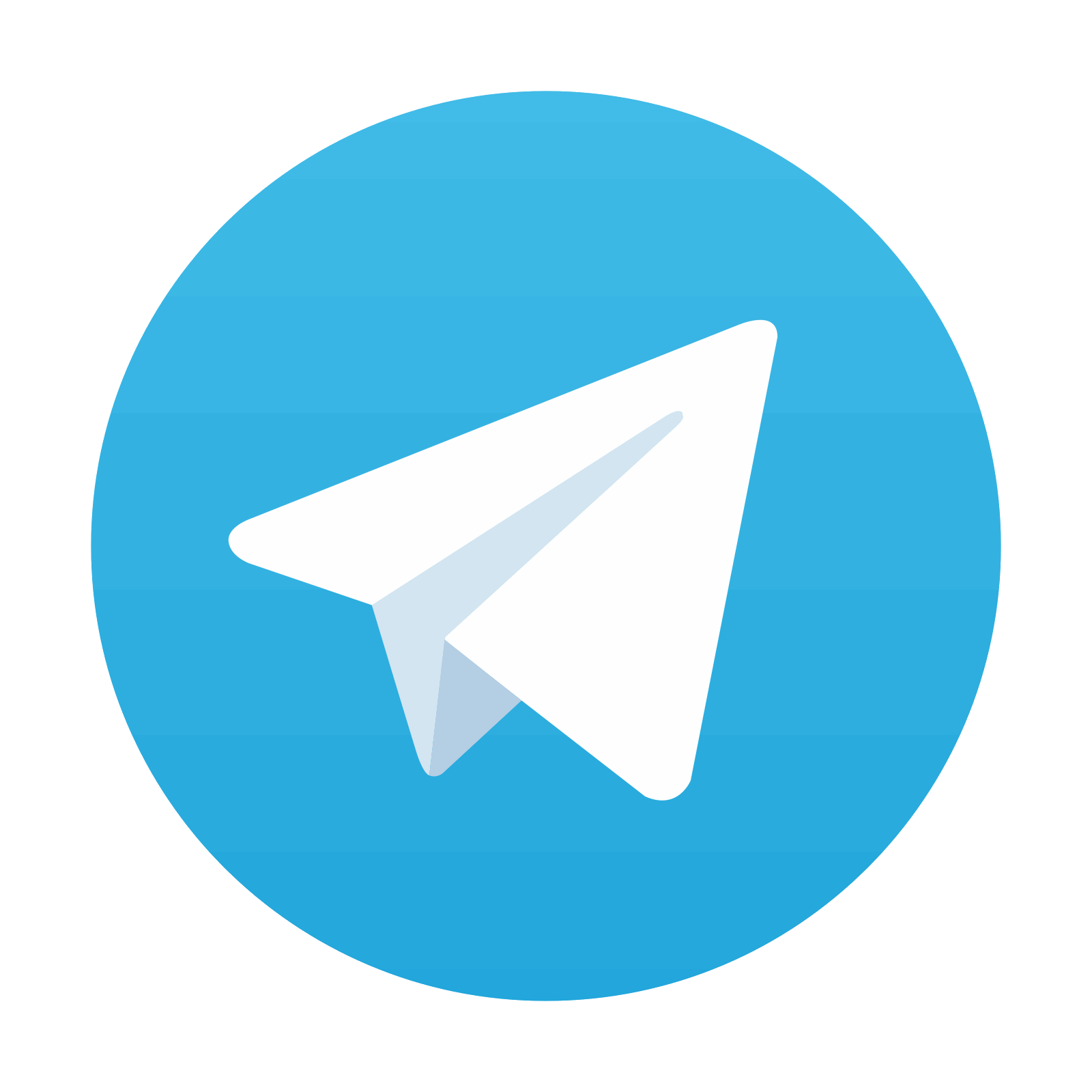
Stay updated, free articles. Join our Telegram channel

Full access? Get Clinical Tree
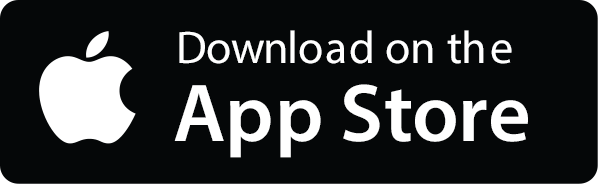
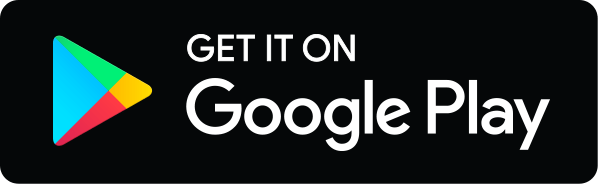