aThe marginal layer free from nuclei (not included) appears between the 4th and 5th wk, after which it disappears. In addition, the transient layer of Chievitz (not included) appears between the 6th wk and 3rd mo and disappears after the 3rd mo.
The Neuroretina
The embryogenesis of the neuroretina occurs during the first month of life. The forebrain consists of a single layer of neuroectodermal cells. The optic vesicle extends laterally from the forebrain and then invaginates to form the optic cup. There is a double layer of neuroectodermal cells in the optic cup; the apices are together and basal aspects apart. The macular area appears at the end of the 4th week.
The inner layer of neuroectoderm becomes the sensory retina posteriorly. Part of the inner limiting membrane is the basement membrane of the sensory retina, next to the vitreous. The foveal pit forms late in embryonic life, and morphologic maturity does not occur until the age when it is possible to obtain 20/20 visual acuity in small children (2). The induction of the foveal pit requires a normal pigment epithelium (3). The inner layer of neuroectoderm is divided into the inner neuroblastic layer (Müller, amacrine, and ganglion cells) and the outer neuroblastic layer (cone and rod, horizontal, and bipolar cells). Between the inner neuroblastic layer and the outer neuroblastic layer is the transient fiber layer of Chievitz; this layer will progressively disappear.
The central and peripheral retinae start to differentiate between the first and third months. The ganglion cell layer becomes thicker, as do the inner plexiform layer and the amacrine cell layer. The cones appear in the 5th month. They are a protoplasmic extension of the outer neuroblastic layer (4–6). The rods appear in the 6th month (5). The macula becomes thinner in the 7th month because the cells of the different layers move laterally, and the foveal pit appears more evident (Fig. 1.1) (7,8).
Figure 1.1 Diagram showing the development of the human fovea. A. Human fovea at birth. B. Human fovea at 45 months. C. Human fovea at 72 years of age. Black lines mark the width of the rod-free foveola; at birth, the foveola is wider (A) than at 45 months (B). Full foveal development is not complete until sometime between 15 and 45 months of age.
The Retinal Pigment Epithelium
The outer layer of neuroectoderm (toward the sclera) becomes the retinal pigment epithelium (RPE), the pigmented ciliary epithelium, and the iris dilator muscle. The basement membrane of the RPE forms the innermost part of the Bruch’s membrane (9).
Pigmentation of the neuroectodermal cells occurs early and is completed during embryonic life (10), whereas pigmentation of the uveal stroma, which is derived from the neural crest, starts much later and is not complete until several weeks after birth (9). The melanin granules of the neuroectoderm are larger and chemically different from those of the uveal stroma (11). Moreover, melanin content of the RPE is similar in all persons, regardless of race, in contrast to the amount of uveal stromal pigmentation, which corresponds to the racial pigmentation of the skin and hair (10).
Vascularization
Vascularization of the retina begins during the 16th week of gestation at the optic nerve head (12) and normally reaches the ora serrata nasally by term. It is not quite complete temporally at term because the distance from the optic nerve head to the ora is greater.
There are differing theories as to how vascularization of the macula occurs. Some have proposed that the capillary-free zone (CFZ) develops by regression of previously formed capillaries, because the retina is thin enough in this area that sufficient oxygen can diffuse inward from the choroidal circulation (12). Others believe that the CFZ forms primarily, with the embryonic vessels gradually encircling the center of the fovea and with no evidence of regression (13).
The Choroid
In the first phase, at 4 weeks, the choroid begins to take form in the undifferentiated mesenchyma surrounding the optic cup. At 5 to 6 weeks, endothelial tubes near the pigment epithelium differentiate into capillaries. Simultaneously, the Bruch’s membrane and the vortex veins appear. With the appearance of the posterior ciliary arteries at 8 weeks, the choriocapillaris is fully established as a discrete layer.
During the growth phase in the 3rd month, the anterior capillaries arrange themselves in a linear and radial pattern. The anterior supply of the choriocapillaris is complete by 3 months, even though the ciliary body and iris are not yet formed. The capillaries drain into the supra- and infraorbital venous plexi.
The second phase of choroidal growth includes the formation of large vessels (Haller’s layer) at 4 months. The third phase begins around the 5th month and is characterized by the formation of medium-sized vessels posterior to the equator. Anterior to the equator, there are only two layers of vessels, the choriocapillaris and medium-sized vessels.
Pigmentation of the choroidal melanocytes commences in the 5th month and continues until after birth, beginning outward near the sclera and progressing inward toward the Bruch’s membrane. The peripapillary, intrascleral vascular circle of Haller and Zinn develops between 3 and 6 months. Between the 6th and 10th month, the major arterial circle of the iris forms recurrent arterial branches that supply the anterior choroid during its rapid growth phase (14).
TOPOGRAPHIC ANATOMY
The terms macula and fovea are used in different ways by the anatomist and the clinician. Anatomically, the macula (macula lutea or central retina) is defined as the portion of the posterior retina that contains xanthophyll and two or more layers of ganglion cells. This region is about 5.5 mm in diameter and is centered approximately 4 mm temporal and 0.8 mm inferior to the center of the optic disk (15). It corresponds clinically to the posterior pole and is approximately bounded by the superior and inferior temporal vascular arcades. On the basis of microscopic anatomy, the macular area can be further subdivided into several zones (Fig. 1.2).
Figure 1.2 Topographic anatomy of the normal macula. On the basis of microscopic anatomy, the macular area can be further subdivided into several zones. I. Fovea containing the foveola (1). II. Parafovea. III. Perifovea.
What is clinically referred to as the macula corresponds to the anatomic fovea. The fovea (fovea centralis) is a depression in the inner retinal surface in the center of the macula. It measures approximately 1.5 mm, or one disk diameter in size, and it is more heavily pigmented than the surrounding retinal tissue. The central floor of the fovea is called the foveola. The anatomic foveola, often referred to clinically as the fovea, measures approximately 0.35 mm in diameter. It lies within the CFZ, which measures approximately 0.5 mm in diameter in most patients. A small depression in the center of the foveola is called the umbo, where the retina is only 0.13 mm thick. A 0.5-mm-wide ring zone where the ganglion cell layer, inner nuclear layer, and outer plexiform layer of Henle are the thickest is called the parafoveal area. This zone is in turn surrounded by a 1.5-mm zone referred to as the perifoveal area (16).
CLINICAL APPEARANCE
The anatomic subdivisions of the macula are ill-defined ophthalmoscopically. The margins of either the 0.35-mm diameter foveola or the 1.5-mm diameter fovea are difficult to define. A poorly defined zone of greater pigmentation (one-fourth to one disk diameter in size) corresponds to the center of the macula, which is maximum in the foveolar area. The foveal reflex is present in most normal eyes, and it lies just in front of the center of the foveola (Fig. 1.3). We can see the foveal depression with the narrow slit lamp beam.
Figure 1.3 Clinical appearance of the normal macula. A. Fundus in a young patient. B. Fundus in an adult patient. C. Fundus in a moderately myopic patient. D. Tessellated fundus in an older patient. Large choroidal vessels are visible in the macular area in (D) because of relative hypopigmentation of the RPE. (B and D courtesy of Dario Fuenmayor-Rivera and Enrique Murcia.)
The central retinal artery supplies the inner half of the retina. It usually divides into a superior and inferior trunk within the optic nerve head. These trunks divide into a nasal branch and a temporal branch. The corresponding retinal venous branches have much the same distribution as the arteries. They give off arteriolar and venular branches that posteriorly occur primarily at right angles to the parent vessel. They divide in a dichotomous way as they course peripherally. The right-angle branches are referred to as first-order arterioles and venules. One or more cilioretinal arteries derived from the ciliary circulation supply the papillomacular area in approximately 20% of patients (17), and occasionally the entire macula (Fig. 1.4). The blood vessel walls are normally transparent (16,18).
Figure 1.4 FA shows a cilioretinal artery. (Courtesy of Dario Fuenmayor-Rivera and Enrique Murcia.)
GROSS ANATOMY
The retina loses its normal transparency within hours after death. Xanthophyll is a yellow pigment made up of two carotenoids (zeaxanthin and lutein) (19,20). It is apparent in the center of the macula and is highly concentrated in the foveolar area. The maximal concentration of xanthophyll pigment is in the outer nuclear and outer plexiform layers. However, we can find xanthophyll within the inner plexiform layer inside the foveal area (19,21–23). The greatest concentration of pigment is in the cones’ central axons (23).
The entrance site for the short and long posterior ciliary arteries can be visualized after removal of the choroid. There is no retinal circulation at the foveola. The short posterior ciliary arteries are concentrated in the macular area along the temporal margin of the fovea and the peripapillary area. The temporal long posterior ciliary artery and ciliary nerve enter about one and one-half disk diameters temporal to the center of the fovea (16).
HISTOLOGY
In the macula, we find the thickest portion of the retina surrounding the thinnest portion, the foveolar area. At the umbo, the neural retina consists only of the inner limiting membrane, the Henle’s fiber layer, the outer nuclear layer, the external limiting membrane, and the photoreceptors’ outer and inner segments (Fig. 1.5). The typical neural–retinal histology is established out of the foveola (Fig. 1.6). The neural retina consists of the inner limiting membrane, the nerve fiber layer (NFL), the ganglion cell layer, the inner plexiform layer (synaptic processes between bipolar cells and ganglion cells), the inner nuclear layer (nuclei of bipolar, horizontal, amacrine, and Müller cells), the outer plexiform layer (synaptic processes between bipolar cells and photoreceptors), the outer nuclear layer (nuclei of the rods and cones), the external limiting membrane (formed by cell junctions between photoreceptors and the terminal optical processes of Müller cells), and the photoreceptor layer (rod and cone cells). The bipolar cells, ganglion cells, fibers of the outer plexiform layer (Henle’s fiber layer), and Müller cells are displaced circumferentially and show an oblique orientation in the macula that causes thickening of the marginal zone and a central thinning of the retina, forming the fovea.
Figure 1.5 Histology of the normal macula. CFZ, capillary-free zone; f, foveola; u, umbo. (Microphotograph courtesy of Dario Savino-Zari.)
Figure 1.6 Schematic representation of the human retina illustrating its organization into discrete layers. AC, amacrine cell; BC, bipolar cell; BM, Bruch’s membrane; C, cone photoreceptor; ELM, external limiting membrane; GC, ganglion cell; HC, horizontal cell; ILM, internal limiting membrane; M, Müller cell; NFL, nerve fiber layer; R, rod photoreceptor; RPE, retinal pigment epithelium.
Müller cells are modified glial cells. They span the region from the internal limiting membrane to the external limiting membrane, and they give support to the neural elements of the retina. The internal limiting membrane consists of a basement membrane, which is a surface modification of the vitreous body, and the expanded vitreal processes of Müller cells. This membrane is relatively thick in the macular region except in the area of the foveola. The internal limiting membrane serves as an anchoring structure for the collagen framework of the vitreous. The outer limiting membrane is formed by junctional complexes between cell membranes of the Müller cells and inner segments of photoreceptors. Müller cells are connected to the visual cells by a system of terminal bars (24,25). These junctional complexes probably provide at least a partial barrier to the passage of large molecules in either direction.
The retinal blood vessels supply the inner half of the retina. The major branches of the retinal arterial system have the structure of small arteries, persisting even beyond the equator (15). Retinal arteries do not have an internal elastic lamina; however, they have a well-developed muscularis (five to seven layers of smooth muscle cells posteriorly; one or two layers peripherally). Near the optic disk, the retinal veins have three to four layers of smooth muscle cells, and after a short distance, the muscle cells are replaced by fibroblasts. There is controversy concerning the pattern of distribution of the capillary network in the retina (diffuse arrangement or a two- or three-tier arrangement) (15,26,27). The superficial network is predominantly postarteriolar and the deep network prevenular. There is a distinct radial peripapillary capillary network; this network richly interconnects with the inner retinal capillary layer (27). The large arterioles and venules of the retinal circulation travel in the NFL and ganglion cell layer. Close to the CFZ (0.4–0.5 mm in diameter), the capillaries form a single layer, but elsewhere, the capillaries are present in two or more layers and extend into the inner nuclear layer. The CFZ is normally vascularized during prenatal development of the retina. This vascularization undergoes spontaneous capillary obliteration just before or shortly after birth, forming the CFZ (28).
The foveola is composed entirely of cones; the central 100 μm of the foveola contains only red and green cones (29). The peak foveal cone density averages nearly 200,000 per mm2 and falls rapidly with increasing eccentricity, such that cone density decreases nearly 10-fold within 1 mm of the umbo. Blue cone density is highest in a zone between 100 and 300 μm from the center of the fovea. The foveal cones take on a more rodlike shape; blue cones in the macula tend to have inner segments 10% taller and a more cylindrical shape than their red and green counterparts (29). Rod cells differ from cones, with their outer segments consisting of stacks of flattened membrane disks that are separate from the plasma membrane.
The RPE is a monolayer of hexagonal cells densely adherent to one another by a system of tight cellular junctions or terminal bars that make up the outer blood-retinal barrier, which maintains the subretinal space in a state of deturgescence. In the fovea, there are 30 cones per RPE cell, while in the periphery, there are 22 rods per RPE cell. Interdigitation of the RPE cells with the rod and cone outer segments provides only a tenuous adhesion of the RPE to the sensory retina. The RPE cells in the macular region are taller and contain increased amounts of melanin pigment than elsewhere (30,31). There is an inverse relationship between melanin and lipofuscin pigment concentration in the RPE. Lipofuscin concentration increases initially during the first two decades of life, and then again in the sixth decade. The concentration of lipofuscin in the RPE is significantly greater in light- than dark-skinned persons, whereas the concentration of melanin in the pigment epithelium is similar in light- and dark-skinned persons. The melanin content of the pigment epithelium and choroidal melanocytes decline with age (Fig. 1.3).
In young and middle-aged individuals, the RPE is tightly adherent to the underlying Bruch’s membrane by means of its own basement membrane. This adherence decreases with advancing age. The Bruch’s membrane consists of the basement membrane of the RPE, the inner collagenous layer, an elastic layer, an outer collagenous layer, and the basement membrane of the choriocapillaris. Because of its porous structure, it probably plays a minimal role in regulating movement of substances across it.
The choroid is the posterior aspect of the uveal tract; it is supplied by the short ciliary arteries, and they are concentrated in the macula and peripapillary region. These arteries form a rich anastomotic network that quickly empties large quantities of blood into the choriocapillaris (sinusoidal network). The choriocapillaris is fenestrated and arranged in a lobular pattern, with a feeding arteriole in the center of each lobule and several venules peripherally (Fig. 1.7) (32–40).
Figure 1.7 Schematic representation of the lobular pattern of the choriocapillaris. Each lobule is supplied by an arteriole. BM, Bruch’s membrane; CA, choroidal artery; CV, choroidal vein; RPE, retinal pigment epithelium.
The prelaminar part of the optic nerve is supplied by the peripapillary branches of the short posterior ciliary arteries. The choriocapillaris does not communicate directly with the optic disk capillaries. The prelaminar capillaries freely anastomose at the disk margin with those of the retina. Both capillary systems drain into the venules leading to the central retinal vein (33,40).
The RPE is an integral part of the visual cycle. It is an important component of photoreceptor renewal; new outer segment disks are continually added proximal to the base, and the oldest disks at the distal end of the outer segments are phagocytized by the RPE cells. The RPE also transports metabolic wastes from the retina into the choriocapillaris. The melanin within the RPE cells absorbs light that has not been captured by the photoreceptors, thus preventing excessive light scattering within the eye. Melanin absorption may also confer protection from photo-oxidative stress (41). The RPE cells are also thought to secrete growth factors essential for proper differentiation of photoreceptors during development (42).
The macula has the highest rate of blood flow of any tissue in the body. It probably functions to stabilize the temperature environment of the retina (43) because blood flow is greatly in excess of that needed to meet the nutritional demands of the retina. The choriocapillaris supplies the RPE and outer retinal layers. The choriocapillaris has an endothelium with a pore size sufficient to allow some larger molecules, including proteins, to escape into the extravascular space. There are no lymphatic channels in the eye; the perivascular and perineural spaces in the sclera probably function as lymphatic channels. Thus, the choriocapillaris endothelium controls the amount of extracellular fluid normally present in the choroid.
BLOOD–RETINAL BARRIERS
Retinal perfusion is accomplished by a nonoverlapping, dual system of blood circulation. For each system, there is a blood–retinal barrier analogous to the blood–brain barrier. Both barriers confine even relatively small molecules, because of a nonleaky tight junction between cells (44).
The outer blood–retinal barrier is constituted by the RPE; it blocks the inward migration of small molecules from the choriocapillaris into the subretinal space. Anatomically, these junctions include a zonula adherens and adjacent zonula occludens of the RPE, both situated near the apex of the cell and encircling it (45). The inner blood–retinal barrier is the retinal vascular endothelium, including the capillary endothelium. The site of the barrier is the specialized tight junctions (zonulae occludentes) between individual endothelial cells (44,46).
NORMAL FLUORESCEIN ANGIOGRAPHIC FINDINGS
Fluorescein angiography (FA) was developed in the 1950s as a means of studying vascular flow. FA is still used for this purpose, but it provides much additional information. Sodium fluorescein is excited by a blue light (465–490 nm), and it emits a fluorescent yellow-green light (peak wavelength of 520–530 nm). Because of its molecular weight (376 kDa), it diffuses freely out of all the body capillaries except the retina. Approximately 80% of the dye is bound to plasma proteins (albumin), and it is the unbound fluorescein that is detected angiographically (16).
FA is performed using a fundus camera. Filters are used to produce the exciting wavelength. The FA is photographed digitally or with black and white film because of its superior resolution and speed. On the positive images, fluorescein appears white and nonfluorescent areas appear black (46). The normal FA shows the dual nature of the retinal circulation. The larger choroidal vessels fill first, with almost immediate filling of the choriocapillaris. Rapid perfusion of the choroid and leakage of the dye from the choriocapillaris give the fairly uniform background fluorescence.
The tight junctions of the retinal pigment epithelial cells (the outer blood–retinal barrier) block the fluorescein that leaks from the choriocapillaris and diffuses through the Bruch’s membrane. Normally, the fluorescein does not gain access to the subsensory retinal space. The retinal vessels, including the capillaries (the inner blood–retinal barrier), normally do not leak fluorescein. Thus, the angiogram evaluates both of the blood–retinal barriers (44).
The normal macular region is hypofluorescent or has a barely visible fluorescence because of the greater density of the RPE in this area and the presence of xanthophyll in the outer retinal layers. In darkly pigmented individuals, the increased density of choroidal melanocytes in the macula helps obscure the background choroidal fluorescence. In addition, the capillaries appear particularly distinct, because there is only one capillary layer surrounding the foveal avascular zone (47). Only in the extramacular area, before the arteriovenous phase, can details of perfusion of the larger choroidal vessels be detected.
The FA consists of five phases (Fig. 1.8):
Figure 1.8 FA of normal fundus. A. Red-free photograph. B. Arterial phase. C. Early arteriovenous phase. D. Intermediate arteriovenous phase. E. Venous phase. F. Recirculation phase. (Courtesy of Dario Fuenmayor-Rivera and Enrique Murcia.)
1.Prearterial phase: The choroid and choriocapillaris fill with dye. A cilioretinal vessel, if present, usually fills at the same time as the choroidal circulation, before fluorescein is detectable in the other retinal vessels, approximately 1 second before that of the proximal branches of the central retinal artery (Fig. 1.4).
2.Arterial phase: Lasts until the arteries are completely filled.
3.Arteriovenous phase: Characterized by complete filling of the arteries and capillaries and the first evidence of laminar flow in the veins.
4.Venous phase: Begins as the arteries are emptying and persists until the veins are filled with dye.
5.Recirculation phase: Follows the venous phase and represents the first return of blood to the eye after fluorescein has passed through the kidneys. During the recirculation phase, the outer edges of the major retinal vessels appear relatively hyperfluorescent because of the greater amount of fluorescein in the tangential section of the plasma cuff near the edge of the blood vessels.
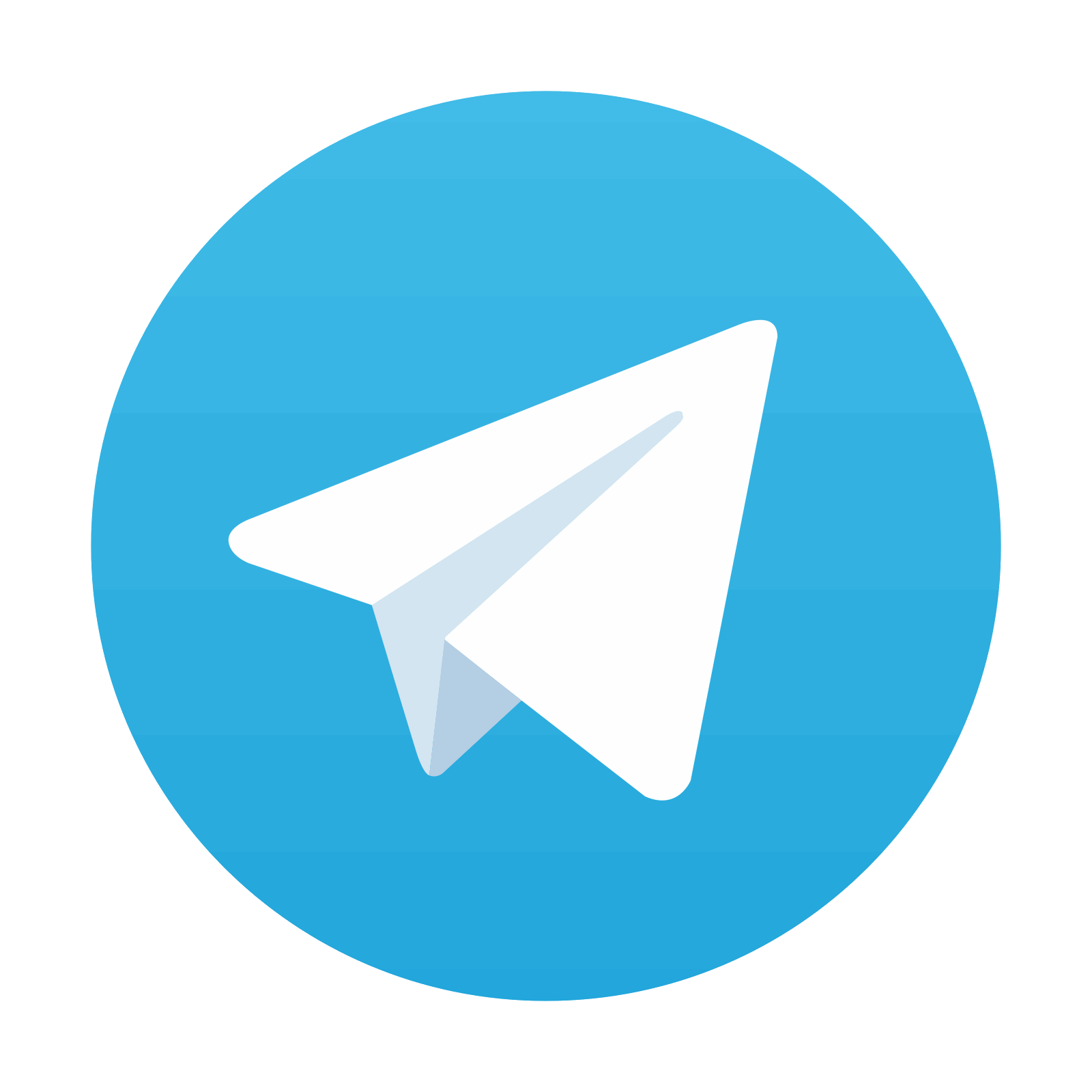
Stay updated, free articles. Join our Telegram channel

Full access? Get Clinical Tree
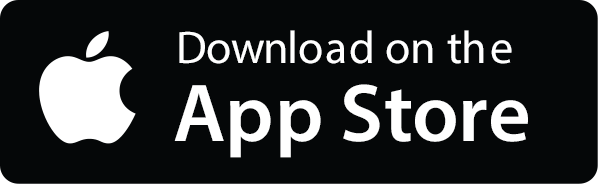
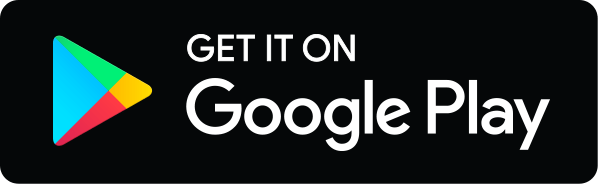