23
Nonhereditary Hearing Impairment
Edwin M. Monsell, Michael T. Teixido, Eric L. Slattery, Mark D. Wilson, Brian Kung, and Gordon B. Hughes
The most common causes of hearing loss in our society today are age and occupational noise exposure.
Presbycusis
Presbycusis, the hearing of old age, encompasses the loss of peripheral auditory sensitivity, a decline in word recognition abilities, and associated psychological problems and communication issues. The auditory system is particularly prone to the effects of aging. The cells of the auditory system, being specialized neural structures, belong to the “fixed postmitotic” group of cells of the body and cannot reproduce.1 Because of this, cells that can no longer function properly due to accumulated errors in DNA transcription,1 inefficient protein syntheses,2 or accumulation of insoluble pigments3 cannot be replaced by new cells.
Many factors have been implicated in the pathogenesis of presbycusis, including genetic predispositions, occupational exposures, diet, cardiovascular disease, smoking, alcohol abuse, and head trauma.4–6 Rosen et al4 coined the term socioacusis to describe the socioeconomic factors that contribute to more severe presbycusis in industrialized societies than in rural Africa. They suggested that diet and noise exposure were more important than socioeconomic factors per se. Rosenhall et al5 studied hearing levels at ages 70 to 85 years in a longitudinal study and found a weak correlation between hearing loss and smoking, alcohol abuse, and head trauma in men, and between hearing loss and intake of pharmaceutical agents (especially salicylates) in women. Gates et al6 found a small but statistically significant association between cardiovascular disease and hearing status in the elderly that is greater for women than for men and more in the low than in the high frequencies. Pearlman7 proposed a working clinical definition of “classic presbycusis” that required (1) bilaterally symmetric sensorineural hearing loss, (2) absent or partial recruitment, (3) a negative history for noise exposure, and (4) poorer speech discrimination than predicted by pure-tone sensitivity. Decline of word recognition out of proportion to pure-tone sensitivity has been termed phonemic regression.8 It is thought to be due to a decline in central auditory function and may be important in auditory rehabilitation. Not all cases of presbycusis show these classic features.
Epidemiologic cross-sectional surveys of elderly subjects have shown that hearing thresholds increase steadily with increased frequency and age, that women hear substantially better than men in higher frequencies, and that thresholds for the right and left ears tend to be similar for the majority of adults.9–12
Jerger et al13 reviewed large-scale surveys of hearing published during the past 50 years and revealed a “gender reversal” phenomenon in the average audiograms of the elderly; above 1 kHz, men showed greater average loss than women, but below 1 kHz, women showed greater average loss than men. The effect increases with both age and degree of hearing loss and remains after persons with a history of noise exposure are excluded from the analysis.
Presbycusis usually reflects a gradual decline in hearing sensitivity but may be highly variable in its age of onset and rate of progression. Among the 1662 subjects in the Framingham cohort, pure-tone thresholds increased with age.14 The rate of change with age did not differ by gender, although men had poorer threshold sensitivity. Word recognition ability declined with age more rapidly in men than in women and was poorer in men than in women at all ages.14
A subclassification of seven types of presbycusis has been suggested, based in part on studies that attempted to relate histopathologic findings and characteristic audiometric shapes. The seven types are sensory, neural, strial, cochlear conductive, indeterminate, central presbycusis, and middle ear aging.1
Sensory Presbycusis
Sensory presbycusis results in a steeply down-sloping audiometric configuration beginning just above the speech frequencies, with slow deterioration of hearing sensitivity beginning in middle age.1,15 Steeply sloping changes in pure-tone thresholds are predominantly an expression of degeneration of the organ of Corti. The lesion is often restricted to the mid-first turn of the cochlea, has sharply defined boundaries, and may involve only the outer hair cells or both the outer and inner hair cells.16 A sensory lesion restricted to the outer hair cells causes a 50-dB threshold elevation for those frequencies having their tonotopic locus within the area of the lesion.16 Advanced lesions show loss of supporting cells, degeneration of the sensory cells, and replacement by epithelioid cells.1,17,18 Typically, the sensory lesion occurs above the speech frequencies, and discrimination scores are well preserved.
Neural Presbycusis
In neural presbycusis, first-order neurons are lost, often with preservation of sensory cells.1,17,18 Although a severe sensory lesion that involves the sustentacular elements of the organ of Corti may be associated with retrograde degeneration of cochlear neurons,19 neuronal degeneration does not further aggravate a sensory hearing loss because the neural loss is obscured by the preexisting hair cell loss.16 The typical audiometric pure-tone pattern in neural presbycusis is a down-sloping high-frequency configuration, relatively more flat than that seen in sensory presbycusis. Speech frequencies are affected, and there exists a decline in speech discrimination scores that is disproportionate to the degree of pure-tone hearing loss. Thus, neural presbycusis may be a cause of phonemic regression. Pathologically, neurons are lost through the cochlea but more so in the basal turn.1,20 In primary neuronal degeneration, the number of primary auditory neurons lost must exceed 50% of the neonatal normal level before word recognition scores are affected.21
Strial Presbycusis
Because the stria vascularis has no tonotopic organization, it cannot cause hearing losses with discrete frequency boundaries. A logical inference has been made that strial atrophy results in a degradation in the quality of endolymph, which results in a uniform elevation of threshold for all frequencies.16 An effect on word discrimination scores would not be expected. A relatively flat pattern of pure-tone thresholds is seen. Speech discrimination scores are well preserved, often not declining until the pure-tone average exceeds 50 dB.1 This type of hearing loss is the only condition in which strial atrophy occurs as an isolated acquired lesion.22 The pattern of atrophy may be patchy or uniform, or may occur predominantly in the apical half of the cochlea. Large intracellular vacuoles, cystic structures, or basophilic deposits may be seen.1,23 Similar patterns of strial damage are also seen in hearing loss due to other causes, such as ototoxicity. The volumetric loss of stria vascularis must exceed 30% before pure-tone thresholds are affected.24
Cochlear Conductive and Indeterminate Presbycusis
Cochlear conductive presbycusis refers to an age-related decrease in pure-tone sensitivity and word discrimination score with no histopathologic findings.22 Hearing loss usually starts in mid-life with slow progression. It is proposed that this hearing loss results from excessive stiffness of the basilar membrane with impaired mechanical-electrical transduction of sound energy.22 The greatest effect on hearing sensitivity would be expected to be at the basal end, where the basilar membrane is shortest. The resulting audiometric configuration would be a down-sloping linear pattern.
In Schuknecht et al’s23 original study, 11.9% of patients had sensory presbycusis, 30.7% had neural presbycusis, 34.6% had strial presbycusis, and 22.8% had conductive presbycusis. In a later study, Schuknecht1 reported that 25% of all cases of presbycusis show no histopathologic correlates of hearing loss. He classified these as cases of indeterminate presbycusis. It has been postulated that the malfunction is caused by impaired cochlear mechanics, altered biochemistry, or histologic changes that cannot be resolved or demonstrated by light microscopy.16,25
Frequency selectivity, which is important in the understanding of human speech, refers to the ability of the ear to discriminate between two simultaneously occurring sounds of different spectral composition. Using psychoacoustic tuning curves, Matschke26 found that frequency selectivity mainly concerns frequencies above 2 kHz and is significantly impaired by progressive loss of outer hair cells in the basal parts of the cochlea in old age. Although pure-tone audiograms show high-frequency hearing loss earlier in life, frequency selectivity is not significantly disturbed before age 60.26 Another study did not demonstrate a statistically significant correlation between speech discrimination and frequency selectivity.27 These studies illustrate the point that explanations of indeterminate presbycusis based on the limitations of light microscopy fail to acknowledge the contribution of central auditory factors to auditory disability. The scheme of sensory, neural, strial, and cochlear conductive or indeterminate presbycusis is conceptually useful, but of limited clinical applicability.
Central Presbycusis
Central presbycusis is the central integrative and synthesizing hearing disability that reflects a progressive deterioration of the central nervous system.28 Although the existence of central presbycusis follows from the aging of the brain, studies of central auditory deficits in aging populations have been confounded by the effects of aging on the auditory periphery, which affects the measurement of central auditory function.29 Nonetheless, several studies suggest a strong relationship between hearing ability and central auditory function.29–31 Fire et al32 found a significant correlation between self-perceived hearing handicap and central auditory nervous system status in 30 elderly adults. Substantial evidence for a biochemical basis for central presbycusis has been presented by Caspary et al,33 who have demonstrated substantial, selective, and age-related loss of the inhibitory neurotransmitter γ-aminobutyric acid (GABA) in the central nucleus of the inferior colliculus of rats. Neurons in the central nucleus of the inferior colliculus are important in the localization of sound in space and are sensitive to differences in interaural time.34,35 Nevertheless, the functional significance of these changes remains unclear. Thus, Kelly-Ballweber and Dobie36 found equivalent performance on central auditory tests in elderly subjects and audiometrically matched young adults.
Middle Ear Aging
Degenerative changes in the middle ear can also influence hearing in the elderly population. Nixon et al37 showed that a mean air—bone gap of 12 dB occurred in a group of elderly patients, typically at 2000 to 4000 Hz. They proposed that hearing loss resulted from loosening of the ligaments and articulations of the ossicles. Belal and Stewart38 also demonstrated arthritis and ankylosis of the ossicles with aging in histopathologic preparations. Old ear canals lose some of their rigidity. The resulting collapse under the audiologist’s headphones may result in a false air—bone gap. This phenomenon may be avoided by placing a short length of rigid plastic tubing in the external ear canal or by using insert earphones.
Hearing loss has been linked to depression and cognitive decline in the elderly,39,40 but the reported relationship may be due to other factors associated with aging and hearing loss.41
It is the responsibility of the otolaryngologist to distinguish presbycusis from hearing loss that can occur from pathologic processes other than aging. Thus, a complete otologic history, physical examination, and audiometric testings are appropriate components of the evaluation. One important treatable cause of hearing loss that is more prevalent in the middle-aged and elderly is Paget’s disease of bone.42 A high-frequency sensory loss and a low-frequency air—bone gap are characteristic when the temporal bone is involved.43,44 The hearing loss may progress at a more rapid rate than presbycusis.45 Histopathologic correlates are lacking.46 Treatment, which is associated with little or no morbidity, may stabilize the process,47 although it is questionable that established Pagetic hearing loss can be reversed. Paget’s disease may cause hearing loss even when its manifestations are not obvious on physical examination. Early detection is important. Patients may be screened for Paget’s disease with a test for serum alkaline phosphatase; the disease may be confirmed by high-resolution computed tomograpy (CT) imaging of the temporal bones.45 There is no known medical or surgical treatment for true presbycusis. When other pathologic processes, such as infection, Meniere’s disease, otosclerosis, Paget’s disease, and so on, have been ruled out or treated, auditory rehabilitation of presbycusis is appropriate (see Chapter 30).
Noise-Induced Hearing Loss
Some important principles emerge from the physics of sound and a few well-established observations. We are more susceptible to noise-induced hearing loss (NIHL) from sound at frequencies within the audible spectrum than from sound outside that spectrum. Since the 1960s, sound level meters have incorporated a weighting standard, called the A-network, that has a relationship of frequency to sound pressure level similar to an inversion of the human audibility curve. Such measurements reflect the risk that measured sounds could damage human hearing and are expressed in dBA.
Damage to human hearing may begin when one is subjected to 8-hour daily exposures of continuous sound at 85 dBA.48 As might be expected, higher sound intensities may be safe if the duration of exposure is shorter. Sound intensity is energy per unit area per unit of time. A 3-dBA increase measured by a sound pressure meter is equivalent to doubling of the sound intensity. Thus, for halving the time of daily exposure from 8 hours to 4 hours, it would be logical that the safe level of sound intensity could be increased by 3-dBA to 88-dBA. However, there is evidence that shorter daily durations of exposure are more likely to be intermittent in the workplace. Intermittent sound exposure may result in less NIHL even if the total intensity of exposure is the same. Thus, the Occupational Safety and Health Administration (OSHA) of the U.S. Department of Labor has adopted a 5-dB trading rule. According to these regulations, for every halving of the duration of noise exposure, the intensity can be increased by 5 dB without increasing risk of NIHL.49 Other groups have used a more conservative 3- or 4-dB trading rule, reflecting that there is no universal agreement that any one rule is best.50,51
A noise-induced temporary threshold shift (TTS) is defined as the reversible increase in the threshold of audibility for an ear at a given frequency following exposure to noise. A true TTS must be distinguished from physiologic phenomena of sensory adaptation, such as residual masking.52 In experimental studies, a TTS is often measured 2 minutes after cessation of the noise.53 OSHA requires hearing conservation programs to test hearing sensitivity at least 14 hours after exposure to noise to establish a worker’s baseline hearing levels.49 In 1930, when the average workday was 16 hours,53 TTSs of 25 to 30 dB were common.53 A daily exposure to sound levels that do not produce a TTS will not result in a permanent threshold shift (PTS) over a worker’s lifetime.54
The NIHL is usually bilaterally symmetrical, but asymmetries of 15 dB or more are not uncommon.55 In pure NIHL, profound hearing loss is rare. Low-frequency thresholds are rarely lower than 40 dB, and high-frequency thresholds are rarely lower than 75 dB. NIHL does not progress after cessation of noise exposure.55 After 15 years of continuous exposure, NIHL may continue to progress, but at a much slower rate.48,56
There are at least several reasons why the 3- to 6-kHz region of the cochlea is most affected when the ear is exposed to high levels of broadband noise. The acoustic resonances of the ear canal and middle ear enhance the sensitivity to sounds from 1 to 4 kHz.57 The acoustic reflex attenuates sound at frequencies below 2 kHz.58 It is possible that mechanical forces may be stronger in the 3- to 6-kHz region due to the geometry of the cochlea, or that its blood supply may be more tenuous there.17,59 The principle of special vulnerability of this region is supported by the fact that hearing loss in head trauma also affects this region preferentially.48
Permanent threshold shift was studied in detail by Passchier-Vermeer, who followed patients after 10 years of noise exposure.60 When data were corrected for presbycusis, 0-dB PTS was seen for noise exposure of 80 dB or less in an 8-hour day. Noise of 85 dB produced 10 dB PTS at 4000 Hz; 90-dB noise produced a 20-dB shift at 4000 Hz but none in the speech range (500, 1000, and 2000 Hz). Noise intensity greater than 90 dB produced shifts greater than 20 dB, including the speech range.60 Laboratory and field studies have resulted in the development of tables that predict levels of noise-induced PTS.60
Impulse noise is more difficult to measure than continuous noise. At a sufficient sound pressure level to produce a threshold shift, the amount of shift is proportional to the number of pulses given.61
There is much interest in factors that might potentiate or reduce the risk of NIHL. Animal experiments have demonstrated that magnesium may have a protective effect.62 This protection is thought to be mediated at the level of the cell membrane by preventing excessive inflow of calcium, which is already great during periods of heavy noise stimulation.63 Excessive intracellular calcium plays an important role in cell death.64 The inverse relationship between NIHL and serum magnesium concentration has also been demonstrated in humans in the range of normal serum magnesium concentrations.65 A placebo-controlled double-blind study involving 320 military recruits demonstrated that magnesium has a protective effect.63 The total group received a drink containing either magnesium aspartate or placebo (sodium aspartate) every working day during a 2-month training period. In the placebo group, the percentages of ears with PTS greater than 25 dB after exposure to firearm noise were twice as high as those in the magnesium group. Long-term follow-up of the study group demonstrated that noise-induced PTS was significantly more frequent and more severe in the placebo group than in the magnesium group.66 Long-term additional intake of a small dose of oral magnesium was not accompanied by any notable side effects in this study group.66
In 1987 the remarkable regenerative powers of hair cells in the chick cochlea following damage from intense sound exposure were demonstrated.67–70 Hair cell regeneration, recovery of the cellular surface areas, and partial restoration of the tectorial membrane were noted to occur during a 12- to 15-day postexposure interval, with substantial recovery of auditory thresholds.69–72 Details of initial structural damage, the associated consequences of hearing loss, and recovery from both noise damage73 and ototoxic drug damage74 have been documented. A study has demonstrated limited regeneration in rat vestibular hair cells in an intensely vitamin A—enriched environment.75 Currently, there is no clinical application of cochlear hair cell regeneration, although this remains an area of intense investigation and development.
Lindgren and Axelsson76 have demonstrated that noise with simultaneous exercise may increase the susceptibility to TTS. Aspirin in mild to moderate doses has not been shown to exacerbate TTS or PTS in chinchillas,77 but neomycin or cisplatin may do so.78 Iron deficiency has been shown to cause predisposition to TTS and PTS in rats.79 Increased inner ear melanin and brown eye color have been demonstrated to decrease the susceptibility to TTS.80,81 The function of melanin in the cochlea has not yet been established, although it may be associated with scavenging of oxygen free radicals. There is no statistically significant evidence that elderly persons or those with preexistent PTS are necessarily more susceptible to further PTS. As noted earlier, NIHL does not progress if there is no further exposure to hazardous noise.
Canlon et al82 demonstrated that prior exposure to a moderate-level acoustic stimulus can reduce damage caused by later exposure to the same stimulus at high intensity. Guinea pigs were preexposed to a low-level acoustic stimulus before exposure to a stimulus known to yield a PTS. This pre-treatment resulted in about 20 dB less TTS than in animals not preexposed and also resulted in complete recovery after 2 months.82 The middle ear muscles do not play an important role in the mediation of this resistance to noise trauma provided by “acoustic conditioning.”83,84
Clinicians have long been concerned about the risk of over-amplification by hearing aids. To ensure that no NIHL occurs from a hearing aid, the output levels from the aid must be such that they would not cause injury to a person with normal hearing.85 This constraint may be difficult to satisfy for users with severe to profound sensorineural hearing loss. In these patients, some risk of hearing damage must be accepted in exchange for the advantage gained from the use of a hearing aid.56,85
Pathology
A primary loss of sensory cochlear cells is evident in the corresponding area of maximal PTS. The earliest changes are usually seen in the outer hair cells and consist of loss of rigidity and fusion of stereocilia. Resolution of a TTS is associated with the regaining of stereociliary rigidity.86 Initially (in guinea pigs) only the tips of the stereocilia are affected.87,88 In the lesions of PTS in the guinea pig, Gao et al87 observed that the base of the stereocilia was always damaged, the continuity of the cuticular plate was broken, and extracuticular cytoplasm was seen. The continuity of the cuticular plate may play an important role in determining the reversibility of threshold shifts. A proliferation of smooth endoplasmic reticulum with mitochondrial swelling can be seen in these early cases.88,89 Further changes include vacuolization of the cells,88 pyknosis of the nuclei,90 depletion of respiratory enzymes,91 and hair cell lysis.88 Lim and Melnick proposed that these changes were caused by metabolic exhaustion. Changes in cochlear blood flow have also been implicated.92
Acoustic Trauma
Acoustic trauma is distinct from other forms of NIHL in that it refers to sudden mechanical disruption of cochlear and other auditory structures from bursts of intense acoustic energy (i.e., impulse noise). Sound intensity levels above 180 dB can rupture or produce hemorrhages within the human tympanic membrane or disrupt or fracture the ossicular chain.93 Impact noise greater than 140-dB peak sound pressure level may result in a PTS from a single exposure. Such damage is termed acoustic trauma. Some early models of cordless telephones incorporated the ringer into the receiver, resulting in cases of acoustic trauma. Because the noise source was in the low-frequency region, a low-frequency NIHL was produced. These devices have been redesigned to eliminate this risk.94–96 Because the rise time of impulse pressure waves is much more rapid than neural response in the auditory system, impulse sounds are seldom accurately perceived and middle ear protective reflexes are ineffective.
Management
Because there is no effective treatment for NIHL, the most effective management is prevention. If noise exposure is unavoidable, ear protectors may at least reduce the level of exposure.97–99 If working properly, the most effective muffs and plugs may attenuate ambient sound by as much as 30 dB.
The Committee on Hearing and Equilibrium of the American Academy of Otolaryngology—Head and Neck Surgery established guidelines for initiating a hearing conservation program.97 These recommendations included difficulty communicating by speech in the presence of noise, the presence of head noises or ringing in the ears after working in noise for several hours, or a temporary loss of hearing that lasts for several hours after exposure to noise. NIHL has prompted otologic referral criteria for workers exposed to industrial noise.100–105 First, noise levels at work must be assessed. Noise should be characterized as steady (turbine), intermittent, or impulse. Eighty-five dBA is the limit for an 8-hour working day of continuous noise exposure.50
Counseling issues may include advice to avoid exposure to noise particularly after exposure to ototoxic drugs. This advice may need to be observed for months after treatment with aminoglycosides, as they may be retained in hair cells for many months. Nonoccupational noise exposure from power tools and recreational use of firearms should be avoided.
Environmental controls to minimize noise include reduction of noise at the source, reduction of noise transmitted through the building structure, and revision of work procedures to minimize duration of noise exposure.97 If hearing loss continues to progress despite appropriate measures, it may be necessary for the physician to recommend job reassignment.
Audiologic and medical referral criteria for occupational hearing conservation programs were established by the Medical Aspects of Noise Committee of the American Council of Otolaryngology.106 Workers should be referred according to the criteria listed below:
If the average hearing level at 500, 1,000, 2,000, and 3,000 Hz is greater than 30 dB; if there is more than 15-dB difference in average hearing between the ears at 500, 1,000, and 2,000 Hz or more than 30 dB at 3,000, 4,000, and 6,000 Hz; if there is a change from previous audiograms of more than 15 dB in the speech range, 20 dB at 3,000 Hz or 30 dB at 4,000-6,000 Hz; or if variable or inconsistent responses are measured.107
Medical criteria for referral include significant, persistent, or recurrent otologic symptoms.106
The percentage hearing handicap is a binaural assessment that may be calculated using guidelines of the Committee on Hearing and Equilibrium of the American Academy of Otolaryngology—Head and Neck Surgery:
The average of the hearing thresholds at 500, 1,000, 2,000, and 3,000 Hz should be calculated for each ear, and the percent impairment for each ear may be then calculated by multiplying by 1.5% the amount by which the above average hearing threshold level exceeds 25 dB (up to a maximum of 92 dB). The hearing handicap may then be calculated by multiplying the smaller percentage (better ear) by 5, adding this figure to the larger percentage, and dividing the total by 6.107
This percentage hearing handicap can then be applied to statutes and rules pertaining to occupational hearing loss.108–110
In evaluating claims for compensation for occupational hearing loss, it is necessary to confirm the presence of hearing loss, its magnitude, and possible etiologies, including functional hearing loss. It is essential to determine whether occupational noise exposure likely exceeded a time-weighted average of 85 dB. One must also allocate the proportion of hearing loss that may be attributed to NIHL.48,111
It is quite easy for use of portable headphone audio devices to exceed current OSHA recommendations. Similarly, sound levels measured at rock concerts often exceed 95 dB, and TTSs have been measured frequently in conjunction with both.112–115 Nonetheless, guidelines for recreational sound exposure have not been established. Much needs to be done to communicate the risks of recreational noise exposure.
Idiopathic Sudden Hearing Loss
Sudden hearing loss (SHL) has been defined as 30 dB or more sensorineural hearing loss occurring in at least three contiguous audiometric frequencies within 3 days or less.116,117 Although this definition is useful for clinical research, in some patients sudden losses of less magnitude may be quite disruptive, especially if there is a preexisting loss or if the loss occurs in the better hearing ear. Slower loss over more than 3 days usually is described as “rapidly progressive” as opposed to “immediate.”118 Although conductive hearing loss also can be sudden, SHL refers only to sensorineural loss. Approximately 4000 new cases of SHL occur annually in the United States119 and 15,000 annually worldwide,120,121 accounting for about 1% of all cases of sensorineural hearing loss.122 The incidence of SHL increases with age,121 with no consistent sexual predominance1 or geographic clustering.123 The disorder may be cyclical or seasonal.124 There is a 4 to 17% incidence of bilaterality during the patient’s lifetime.119
Etiology
Histopathologic studies have demonstrated atrophy of the organ of Corti, stria vascularis, and tectorial membrane.125,126 Changes were most often seen in the basal turn of the cochlea; however, in some specimens, all turns of the cochlea were involved. The degeneration of neural structures appeared significantly less than that in structures of the cochlea, with vascular channels appearing to be open in all specimens. These pathologic findings were not thought uniformly to represent vascular occlusion of the cochlea similar to that in experimental studies,127,128 but rather more closely resembled those changes seen in viral hearing loss such as that occurring with measles, mumps, and rubella.129–131
There is a 5 to 65% prevalence of previous viral illness in patients with SHL,119,132–135 but until recently investigators could not determine whether this finding was statistically significant.136 In 1983 Wilson et al134 found seroconversion to mumps, rubeola, varicella zoster, cytomegalovirus, and influenza B viruses in 63% of 122 patients with SHL and in 40% of controls. The many infectious agents that have been associated with sudden or progressive sensorineural hearing loss are discussed in a review.137 Perhaps the strongest evidence for viral etiology comes from identification of viruses in the inner ear using indirect immunofluorescence. Cytomegalovirus, mumps, and rubeola have been identified in inner ears of patients suffering from SHL.138
Recoverable sensorineural hearing loss can be induced experimentally by temporary vascular occlusion.139 Many well-documented cases of vascular disease have resulted in SHL, including leukemia, sickle cell disease, vasculitis, and embolization during coronary bypass surgery.
Immunologic causes of SHL include primary “autoimmune” inner ear disease,140–143 temporal arteritis,144 Wegener’s granulomatosis,145 Cogan’s syndrome,146 polyarteritis nodosa,147 and delayed contralateral endolymphatic hydrops.148 Immune-mediated SHL can be either primary and localized to the inner ear, or secondary due to generalized systemic immune disease.149
Up to 13% of patients with acoustic neuromas may present with SHL.150 Of these patients, 23% may recover auditory function spontaneously150 or with steroid therapy.150,151 Although the prevalence is low, any patient with sensorineural hearing loss, including SHL, even with recovery, should be suspected of having an acoustic neuroma. The contralateral SHL associated with acoustic neuroma surgery averages 16.5 dB in high frequencies and 19.5 in low frequencies and typically returns to normal within 3 months.152 This SHL may be caused by autoimmunity149 or by postoperative hypotonia of the cerebrospinal fluid (CSF) or labyrinthine fluids.152 The prevalence of acoustic neuroma in SHL is higher when associated with other cranial nerve involvement (ipsilateral facial numbness), papilledema, or ataxia. The diagnosis is confirmed by appropriate radiologic studies of the internal auditory canal.153–156
Other proposed causes of sudden sensorineural hearing loss include diabetes mellitus,157 perilymphatic hypertension,158 autonomic imbalance159 cochlear hydrops, perilymphatic fistula, and operative complications of nonotologic surgery.153 There is anecdotal evidence supporting an association between SHL and manipulation of the cervical spine. Injury to the vertebral artery is a proposed mechanism of injury.160,161
Evaluation
The evaluation and treatment of SHL should be considered a medical urgency, if not an emergency. The first priority is to discover those potential causes for which there is proven effective treatment, such as autoimmune disease. A thorough history may give clues leading to the discovery of a known cause. The circumstances and characteristics of the onset of hearing loss should be determined. Some patients may have preexistent hearing loss that was discovered accidentally. A history of trauma or noise exposure should be reviewed. Associated otologic symptoms should be identified, including vertigo or imbalance, tinnitus, and aural pressure.
Patients generally volunteer pertinent information; however, some patients may not readily speak about certain events. Examples include alcohol intoxication with secondary traumatic injury or childhood activities done without parental permission. Medical conditions that can be associated with SHL include diabetes, syphilis, and chronic renal and cardiovascular disease.
Usually the physical examination is otherwise normal. Baseline testing should include an otoscopic and complete neurotologic examination. Basic audiometry may-provide prognostic clues. A down-sloping or flat moderate to severe sensorineural hearing loss has a poor prognosis.162 Mild low-frequency losses have the best prognosis. Spontaneous improvement is often followed by further recovery. The presence of vertigo is a poor prognostic finding, often corroborated by abnormal vestibular function testing.
Laboratory blood tests generally are not very helpful but can include fluorescent treponemal antibody-absorption (FTA-abs) test. Tests seeking confirmation of autoimmunity, such as sedimentation rate, C-reactive protein, rheumatoid factor, and antinuclear antibody, are nearly always noncontributory. Antigen-specific Western blot immunoassay is commercially available now at IMMCO Diagnostics, Buffalo, New York. CT or magnetic resonance imaging (MRI) studies of the head are important if trauma or tumor is suspected.
Natural History
The natural history of SHL is highly variable, probably because its pathogenesis is multifactorial. Many patients with SHL improve without treatment of any kind. Because most spontaneous improvement occurs in the first 2 weeks after onset, the prognosis is worse the longer the symptoms last. Studies are prone to selection bias, in that patients might not all be seen in the same stage of disease. In 15% of patients, the hearing loss progresses.136
Treatment
Although many treatment regimens have been proposed for idiopathic SHL,163 at present independent studies have not consistently supported the use of any one treatment modality. The treatment that has been the most widely accepted is corticosteroids, such as prednisone, 1 mg/kg of lean body mass per day for 7 to 14 days.164,165 If hearing levels improve, the course of treatment can be extended. Inhalations of carbogen (95% oxygen with 5% carbon dioxide) have been advocated by some because they may increase cochlear blood flow, at least temporarily.166 A low-salt diet and diuretic therapy, as commonly used in classic Meniere’s disease, has been advocated.137
There are few clinical trials in SHL. Wilson and coworkers116 studied 33 patients treated with steroids. Some received dexamethasone, 4.5 mg twice daily for 4 days, followed by a short tapering dose; others received methylprednisolone, 16 mg for 3 days, which was tapered for the next 8 days. Although the study design contained several flaws, preliminary results suggested that steroids had statistically significant benefits on the recovery of hearing in patients with moderate hearing losses. Moskowitz et al167 confirmed Wilson et al’s results in 36 patients treated with dexamethasone, 0.75 mg four times daily for 3 days, followed by a tapering dose during a total of 12 days. Conversely, Byl,168 found no treatment benefit in an 8-year prospective study of 225 patients with SHL.
The great majority of patients with SHL can be managed in the ambulatory setting with oral corticosteroids (e.g., 1 mg/kg of prednisone for 7 to 14 days), possibly with salt restriction and diuretics. Follow-up audiometry is scheduled along with hearing aid evaluation in selected patients. Appropriate tests are conducted to exclude acoustic tumors. Vestibular suppresives are used if needed. Histamine, peripheral vasodilators, and other less commonly proposed medical treatments have little evidential basis to be recommended at this time.169 The intratympanic application of steroids in SHL has been suggested (see below).170,171 A randomized clinical trial by Tucci et al172 showed no benefit to adding the antiviral medication valacyclovir to standard systemic steroid treatment in SHL.
Because intratympanic (IT) steroids for idiopathic SHL can be given in high concentration without systemic side-effects and risks, this route of administration is becoming increasingly popular. At the time this chapter was written, two important studies were underway, one of them recently completed. Drs. Battaglia and Cueva at Kaiser San Diego (California) performed a multi-center, double-blinded, placebo-controlled randomized study to compare hearing results in idiopathic sudden sensorineural hearing loss (ISSNHL) patients who received either a high-dose prednisone taper (HDPT), IT-dexamethasone alone, or IT-dexamethasone and HDPT. Fifty-one patients with less than a 6-week history of ISSNHL were randomized to one of the three arms and followed prospectively. The ISSNHL patients treated with both IT-dexamethasone and HDPT experienced statistically significantly improved hearing recovery compared with treatment with HDPT or IT-dexamethasone alone (see final publication for details).173
While these results might suggest that every patient with ISSNHL should receive combined systemic and IT steroids, many patients cannot tolerate systemic steroids or cannot take them because of other medical problems. These patients would like to know if IT therapy is equally effective as systemic therapy. This question will be answered by another multi-center, randomized study headed by Steven D. Rauch, M.D. of the Massachusetts Eye and Ear Infirmary.174 Hopefully preliminary results will be available in 2008-2009.
In SHL the treating physician’s primary responsibilities include the following: (1) to diagnose and treat underlying causes, (2) to discuss the risks and potential benefits of treatment with the patient, (3) to treat aggressively or not at all, (4) to arrange rehabilitation for those patients whose hearing does not improve, (5) to exclude the diagnosis of retrocochlear tumor, and (6) to offer follow-up for possible delayed symptoms and contralateral ear disease.
Ototoxicity
Ototoxicity is “the tendency of certain therapeutic agents and other chemical substances to cause functional impairment and cellular degeneration of the tissues of the inner ear, and especially of the end-organs and neurons of the cochlear and vestibular divisions of the eighth cranial nerve.”175 Ototoxicity must be distinguished from neurotoxicity, the process by which drugs and other substances may alter hearing or equilibrium by acting at the level of the brainstem or central connections of the cochlear and vestibular nuclei. Ototoxicity has been recognized since the late 1800s, when it was observed that quinine and acetylsalicylic acid (ASA) produced dizziness, tinnitus, and hearing loss. Since World War II, other drugs, often more toxic, have joined the list of ototoxic substances: aminoglycoside antibiotics, loop diuretics, macrolide antibiotics, and some antineoplastic agents.
Aminoglycosides
The aminoglycosides are highly polar, polycationic compounds, predominantly 4,6-diglycosylated 2-deostreptamines. The first aminoglycoside, streptomycin, was isolated from soil bacteria in 1943, followed by dihydrostreptomycin and neomycin (1949), kanamycin (1957), gentamicin, amikacin, tobramycin, netilmicin, dibekacin, and sisomicin. The bacterial activity of the aminoglycosides, largely against gram-negative and enterococcal infections, results from inhibition of protein synthesis at the level of the ribosome. Aminoglycoside ototoxicity has been reviewed by Forge and Schacht176 and recently by Roland and Rutka.177
Dihydrostreptomycin was developed to treat tuberculosis; its use was quickly abandoned, however, because of the severe and unpredictable cochlear ototoxicity it produced. It differs from streptomycin only by the reduction of an aldehyde to an alcohol. Neomycin, which is also predominantly cochleotoxic, is used only topically or orally for bowel preparation before bowel surgery.178
It has been estimated that ototoxicity due to aminoglycoside use ranges in prevalence from 2 to 15% overall, with values of 2.4% for netilmicin, 6.1% for tobramycin, 8.6% for gentamicin, and 13.9% for amikacin in a survey of prospective studies by Kahlmeter and Dahlager.179 A randomized, prospective study by Matz and Lerner180 found a prevalence of ototoxicity of 5% for gentamicin and 7.5% for amikacin. Proposed risk factors for aminoglycoside-induced ototoxicity have included the type of aminoglycoside, concomitant exposure to other ototoxic drugs (loop diuretics, chemotherapeutic agents), noise exposure, duration of therapy, total dose, plasma level, perilymph level, age, sex, liver dysfunction, renal dysfunction, bacteremia, dehydration, and hyperthermia. A prospective study found that of the latter 11 variables, only age increased risk significantly.181
The highly polar nature of the aminoglycosides accounts for their water solubility, renal excretion, and difficulty with traversing plasma membranes passively. They must be transported actively or engulfed by pinocytosis to reach the interior of cells. Only 1 to 3% of the oral dose is absorbed. Aminoglycosides have very strong tissue-binding properties and may be found in the urine of patients with normal renal function as long as 20 days after the cessation of therapy.182 Animal experiments indicate that the serum half-life of aminoglycosides is about 80 minutes.182 The concentration of aminoglycoside in the perilymph rises slowly, reaching its peak 2 to 5 hours after injection, at a level 3 to 5% of peak serum level.183 The half-life of aminoglycoside in the perilymph has been reported to be from 3 to 15 hours182,183; however, ototoxicity does not correlate with drug concentration in inner ear tissue or fluids, and inner ear fluid concentrations at no time exceed serum levels.184–187 Gentamicin can be found localized in hair cells for weeks to months, even without electrophysiologic signs of ototoxicity.188 Ototoxicity does not correlate with nephrotoxicity; furthermore, damage to the auditory and vestibular systems occurs somewhat independently.
A mitochondrial mutation (A1555G) in the 12S rRNA gene was shown initially in Chinese families to predispose to ototoxicity from aminoglycosides. Only the cochlea is affected.189
Pathophysiology
In animals exposed to aminoglycosides the loss of hair cells of the organ of Corti is most severe in the basal turn of the cochlea and is progressively less toward the apex. The inner row of the outer hair cells is affected first, followed by the outer two rows. The inner hair cells and the rest of the organ of Corti are damaged only in cases of severe toxicity. Evidence of damage to other cochlear structures has also been noted, including the stria vascularis, spiral ligament, spiral prominence, outer sulcus, and Reissner’s membrane.190 Nerve fibers can be damaged after hair cell derangement, but this is thought to be secondary to the changes in the hair cells; ganglion cells do not seem to be affected directly.191,192 Histologic and clinical cases of asymmetric and unilateral cochleotoxicity have been reported.193
Electrochemical studies of animal models of cochlear toxicity demonstrated that aminoglycosides produced a reduction in the endolymphatic potential and cationic content of endolymph.194 Reduction of the levels of adenosine triphosphatase and succinic acid dehydrogenase has been found in the stria vascularis and may explain changes in endolymph composition.195,196
Animal studies of aminoglycoside toxicity suggest that the type I hair cells of the crista ampullaris are damaged earlier than the type II cells. The summit of the crista appears to be the area damaged first, followed by the sloping regions. The hair cells of the saccule appear to be less sensitive to aminoglycoside toxicity than those of the utricle.182
Human vestibulotoxicity has also shown good agreement with animal models. Loss of hair cells, type I more than type II, and vacuolization of the remaining hair cells have been described in the cristae.191,195 Hair cell loss and vacuolization have also been observed in the maculae of the utricle and saccule.193,197
Aminoglycosides cause both acute and chronic ototoxic effects. Acute effects manifest with neuromuscular and auditory blockage, is reversible, and is attributed to calcium antagonism and channel blockade.198–201 Postsynaptic excitatory amino acid blockade has also been postulated.202
Aminoglycosides bond with extremely high affinity to polyphosphoinositides.176 Polyphosphoinositides are membrane lipids involved in intracellular second messenger systems and are also sources of substrates for precursors in the synthesis of prostaglandins and leukotrienes.203
Several lines of evidence reviewed by Schacht176,204 strongly suggest that oxygen and nitrogen free radical species are the primary mechanisms of permanent cellular injury in aminoglycoside ototoxicity. Priuska showed with nuclear magnetic resonance spectroscopy that gentamicin can act as an iron chelator. Priuska and Schacht proposed that a redox active gentamicin-Fe(II) complex activates molecular oxygen in a process that generates reactive oxygen species and damages the cell. Cell death occurs primarily by apoptosis.
A role for ornithine decarboxylase has also been proposed; neomycin and gentamicin are known to inhibit both the cochlear and renal enzymes. Induction of ornithine decarboxylase is important in cellular response to injury, and inhibition could lead to the accumulation of toxic moieties.205
It has been postulated that there exists an as yet unidentified metabolite of the aminoglycosides that is the true toxic agent. This hypothesis has been proposed to explain several observations, including the poor correlation between drug levels and ototoxicity and the delay seen before chronic toxicity occurs. This hypothesis also may account for the observation that gentamicin does not destroy isolated hair cells in vitro, but does so after incubation with liver cytosolic fraction or when injected systemically, applied locally, or in organ culture.206–208 Distribution of such a transforming agent in different amounts in susceptible tissues may also account in part for the differential toxicity in the kidney, cochlea, and vestibular organs.
Some compounds have been proposed to confer partial protection from aminoglycoside ototoxicity, including sulfhydryl compounds and free radical scavengers, though other free radical scavengers have not been effective.209–211 Clinical trials are needed to determine the clinical effectiveness and safety of chemoprotective strategies.
Streptomycin
Streptomycin was the first effective antibacterial agent employed against tuberculosis. As early as 1945, however, it was observed that 2 to 3 g/day of streptomycin would damage or destroy vestibular function in 2 to 4 weeks.190 This effect was dose-dependent and cumulative. Adverse effects on hearing, although reported, were far less common.
McGee and Olszewski212 gave cats 200 mg/kg/day of streptomycin and found that nystagmus developed after 12 days and a sloping sensorineural hearing loss developed after 28 days. Temporal bone sections revealed the previously noted changes in the cristae, with little, if any, damage to the maculae. Changes in the cochlea were confined to the basal turn and varied from loss of outer hair cells to total destruction of the organ of Corti.
Dihydrostreptomycin
Unlike streptomycin, the ototoxic effects of dihydrostreptomycin can occur long after the drug has been withdrawn. Hearing loss has been reported as long as 5 months after discontinuation of the drug and after a patient has received as little as 4 g.190 Animal experiments have demonstrated histopathologic changes similar to those caused by streptomycin. There seems, however, to be greater damage to the outer hair cells, involving both the basal and middle turns of the cochlea.212 Dihydrostreptomycin has been discontinued for clinical use.
Gentamicin, Tobramycin, and Amikacin
Many clinical studies have investigated the ototoxicity of gentamicin, tobramycin, and amikacin. Results have been difficult to compare due to lack of control of confounding variables and variability in methods. Tests of cochlear function have been limited primarily to pure-tone audiometry, and assessment of vestibular ototoxicity usually has not included clinical tests of vestibular function.
In 1980 Fee182 conducted a prospective study of 113 patients who received 138 courses of gentamicin or tobramycin. Dosages were modified to maintain blood levels within the recommended safe ranges, and patients were followed with pure-tone audiometry and electronystagmography. Toxicity was defined as sensorineural hearing loss of 20 dB or greater or reduction by 33% or more of slow-phase nystagmus velocity 90 seconds after irrigation. Cochlear toxicity developed in 16.4% of patients receiving gentamicin and in 15.3% of patients receiving tobramycin. Vestibular toxicity developed in 15.1% of patients receiving gentamicin and in 4.6% of patients receiving tobramycin. The only statistically significant difference between the two drugs was vestibular toxicity (which was less for tobramycin). Cochlear and vestibular toxicity progressed after discontinuation of treatment with either drug. The same type of hearing loss demonstrated in Fee’s study has been observed with amikacin administration by the authors (Fig. 23–1).
Aminoglycoside cochlear toxicity has generally been thought to involve frequencies above 6 kHz. In Fee’s182 study, however, 27% of patients developed significant change only in the 1- to 4-kHz range. Decreased pure-tone sensitivity was unilateral in 91% of patients. Toxicity was first noted 3 to 35 days after the onset of therapy. Fifty-five percent of the patients recovered their loss of pure-tone sensitivity within 1 week to 6 months. Nonrecovery was associated with hearing losses greater than 25 dB, delayed onset, immediate onset that progressed despite discontinuation of the drug, and continuation of the drug after the onset of toxicity. In cases of vestibular toxicity, the mean depression of slow-phase velocity was 45%. The mean time of appearance of vestibular toxicity was 11.5 days after onset of treatment. Fifty-three percent of patients recovered within 10 days to 9 months.182
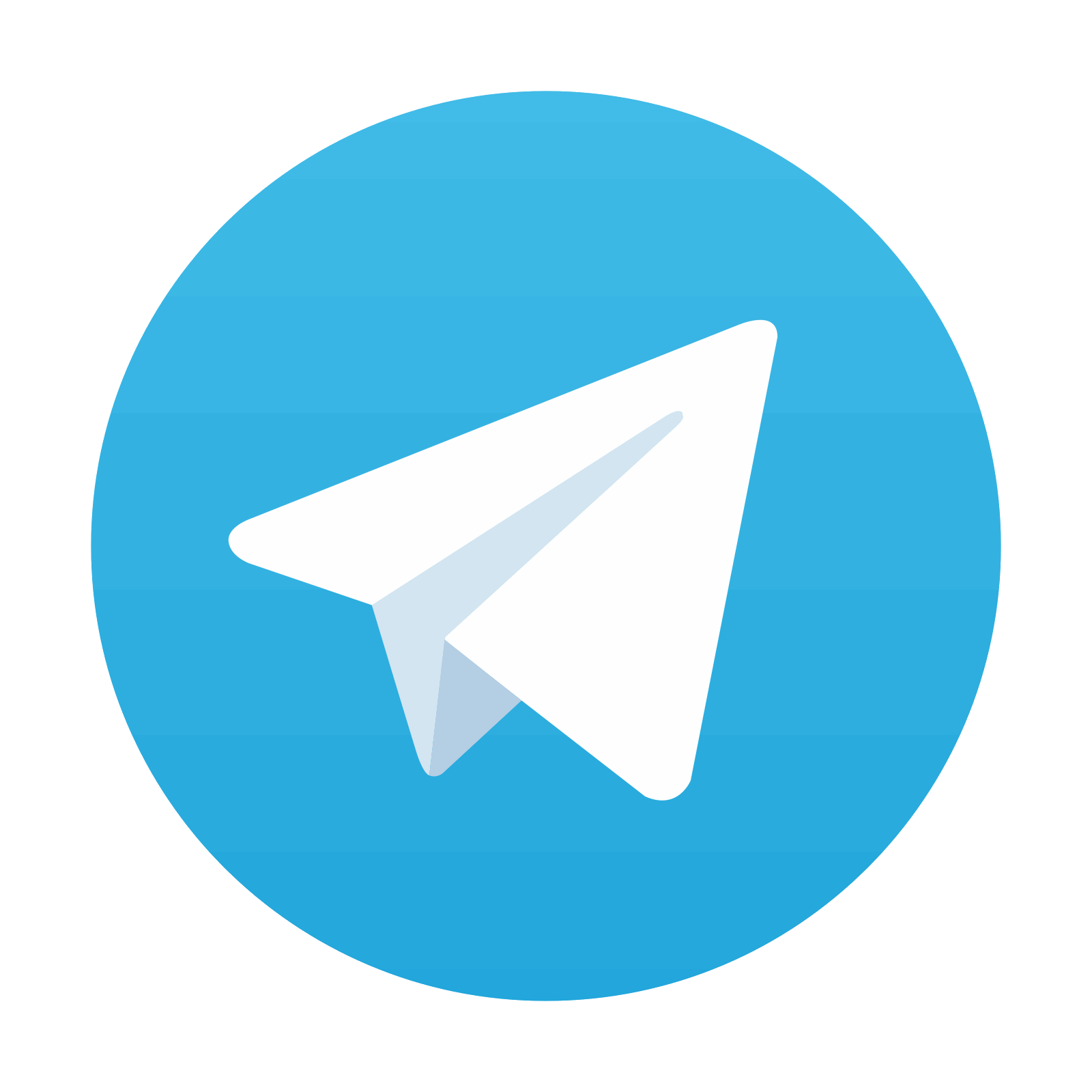
Stay updated, free articles. Join our Telegram channel

Full access? Get Clinical Tree
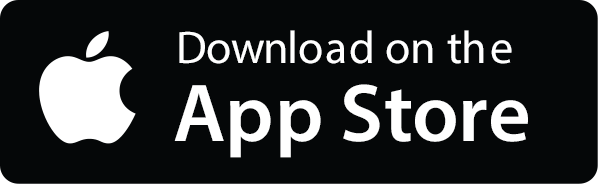
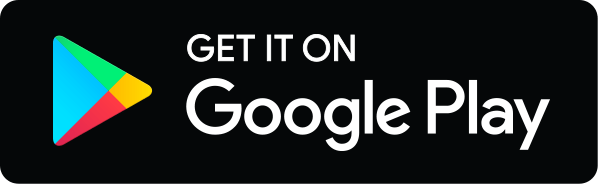