Noise-Induced Hearing Loss
Robert A. Dobie
Noise and aging are responsible for most cases of permanent hearing loss in the United States. Although not correctable by medical or surgical treatment, noise-induced hearing loss (NIHL) is preventable.
Otolaryngologists see patients with NIHL in many different contexts. A noise-exposed worker may be referred for preemployment examination, for evaluation of threshold shifts or other abnormalities detected by a hearing conservation program (HCP) at work, or for examination for compensation purposes at the end of his or her career. The otolaryngologist is expected to verify the existence and severity of the hearing loss, to render a differential diagnosis, to recommend treatment and rehabilitation, to counsel the worker, and to report to referring parties. Otolaryngologists also may be called on as consultants by employers designing HCPs or by attorneys involved in medicolegal and compensation disputes.
Patients with NIHL are also seen through the normal pathways of self-referral or physician referral. These patients often have occupational or nonoccupational exposures that are uncontrolled, and then the otolaryngologist’s duty is to provide at least the rudiments of an HCP: periodic audiometry and counseling, including discussion of hearing protection. Unfortunately, most people with NIHL do not see otolaryngologists; thus, we should encourage primary care physicians either to refer noise-exposed patients or to provide these services themselves (1).
The goal of this chapter is to provide the otolaryngologist with most of the information needed to meet these needs.
PATHOGENESIS
Pure-Tone Threshold Shifts
Temporary Threshold Shifts
Exposure to loud noise for seconds to hours may cause a temporary sensorineural hearing loss that recovers almost completely within 24 hours. The magnitude of this temporary threshold shift (TTS) can be predicted from the acoustic parameters of the noise: its intensity, spectrum (frequency content), and temporal pattern. Obviously, more intense sounds lead to larger shifts. Pure tones cause TTSs that are greatest at and slightly above the frequencies of the exposure tones. As might be expected, the frequencies that we hear best are also the frequencies most susceptible to TTS. (See Chapter 141 for a discussion of the effects of middle-ear mechanics and ear canal resonance on thresholds for different frequencies.) Because high-frequency sounds (e.g., a 4-kHz tone) are usually more hazardous than low-frequency sounds (e.g., a 500-Hz tone) of the same intensity, risk cannot be predicted from measurements of decibel sound pressure level (dB SPL) alone. To avoid the cumbersome necessity of assessing risk separately for each of several octave bands of noise, there is an international consensus that estimates of hazard for NIHL should be based on measurements of decibels on the A scale (dBA), which gives greater weight to those frequencies most hazardous to human hearing (1 to 5 kHz) and lesser weight to higher and lower frequencies.
The effect of temporal pattern is more complex. Up to a point, longer exposures lead to increased TTS, but interrupted exposures cause less TTS than continuous exposures with the same overall duration. Presumably, recovery takes place during the rest intervals.
Permanent Threshold Shift
After repeated exposures to noises that initially cause only TTS, a worker may experience threshold changes that do not recover. This is called noise-induced permanent threshold shift (NIPTS). In a typical field study, a researcher determines the NIPTS attributable to 10 years’ exposure at 100 dBA, for example, by measuring the hearing threshold levels (HTLs) of a group of workers so exposed and then subtracting the amount of hearing loss to be expected on the basis of aging. The amount of NIPTS and the frequencies involved depend primarily on the acoustic parameters
of the noise, as described previously for TTS. As with TTS, intermittency has a protective effect, especially for low-frequency NIPTS (2,3).
of the noise, as described previously for TTS. As with TTS, intermittency has a protective effect, especially for low-frequency NIPTS (2,3).
The consensus of most experts (4,5) is that NIPTS does not progress after cessation of the offending exposure. To be precise, as people with NIPTS from a prior exposure grow older, their hearing loss does not progress more rapidly than hearing loss in people who have not been noise exposed. That consensus view has been challenged recently. Noise-exposed mice may show hearing loss that progresses more rapidly than expected after the noise stops, but this phenomenon has only been demonstrated for a single inbred strain exposed as juveniles (6). A review of serial audiograms from the Framingham Heart Study showed that middle-aged men who had 4-kHz notches (presumably due to noise exposure in most cases) experienced more threshold shift at 2 kHz in subsequent years than men whose audiograms were not notched (7). Unfortunately, there was no documentation that the men with audiometric notches had actually been noise exposed (or that those without notches had not). In addition, many if not most of these men were below retirement age at the times of their initial audiograms, and there was no documentation that their occupational and nonoccupational noise exposures (if any) had ceased. A subsequent longitudinal study from South Carolina showed no difference in age-related progression of hearing loss between retirees who had had noisy careers and those who had had quiet careers (8), in agreement with the expert consensus.
Although the acoustic reflex (contraction of the stapedius muscle in response to loud sound) evolved before firearms and industrial noise, it is probably protective against NIPTS, at least for frequencies below 2 kHz, where the acoustic reflex effectively attenuates sound. Borg et al. (9) have shown in experimental animals and in humans with Bell palsy that permanent threshold shift (PTS) and TTS increase dramatically for lower frequencies when the reflex is inactivated. The efferent innervation of the outer hair cells probably exerts a protective role as well; at least in mice and guinea pigs, strong efferent olivocochlear function is correlated with resistance to NIHL (10,11).
We have already mentioned several explanations for the familiar 4-kHz notch (which can also be at 3 or 6 kHz): the greater sensitivity of the human ear to frequencies between 1 and 5 kHz, the protective effect of the acoustic reflex below 2 kHz, and the fact that intermittency is most protective for low frequencies. Recently, another reason has been added: Outer hair cells at the base of the cochlea are especially susceptible to oxidative stress (12). However, a notch is not proof of NIHL and can be seen after head injury, after barotrauma, or even in the absence of any explanatory history (13).
In both TTS and PTS experiments in animals, the amount of threshold shift caused by a particular exposure can often be reduced by adding prior exposure at lower levels; this is called “toughening” or “conditioning” the ear (14). Clearly, inner ear injury and hearing loss are not simply related to the total amount of sound energy entering the ear.
A landmark cross-sectional study of the evolution of NIHL over a working career was reported by Taylor et al. (15), in a jute-weaving factory in which noise levels (over 100 dBA) had probably been constant for generations. Figure 157.1 shows the pattern of hearing loss found in retired female weavers compared with that of age-matched female controls who had had no hazardous occupational or nonoccupational noise exposure. The shaded area indicates the estimated median NIPTS; the greatest change was at 4 kHz. Figure 157.2 shows median NIPTS curves for varying lengths of employment in the mills. As many other studies have shown, when workers are exposed to typical broad-spectrum industrial noise, the earliest changes are in the high frequencies (3 to 6 kHz). After about 10 years, loss in the high frequencies tends to plateau, but the loss continues to broaden gradually into lower frequencies.
![]() Figure 157.2 Median NIPTS as a function of audiometric frequency for different durations of exposure in jute-weaving mills (at level above 100 dBA). |
Pathology
Animal studies show clearly that NIHL involves the organ of Corti, especially the hair cells (Fig. 157.3). Although almost all cell types may be affected, the outer hair cells are most susceptible to damage. It has been difficult to find anatomic correlates to TTS, but it appears that the stereocilia of the outer hair cells become less stiff and therefore respond poorly to stimulation. These floppy stereocilia may recover their normal mechanical properties and function normally again. With increasing intensity and duration of exposure sufficient to cause NIPTS, more severe damage is seen with fusion of adjacent stereocilia and loss of stereocilia. The primary site of injury appears to be the rootlets that connect the stereocilia with the top of the hair cell (16). As stereocilia are lost, the hair cells themselves may die. As the severity of exposure increases, inner hair cells and supporting cells in the organ of Corti may be damaged (Fig. 157.4), and with severe hair cell loss, secondary neural degeneration is reflected in the auditory nerve and brainstem auditory nuclei. Rapid primary degeneration of VIII nerve synapses, followed by gradual loss of ganglion cells, may also occur, probably caused by glutamate excitotoxicity (17).
Acoustic Trauma
A single exposure to a very intense short-duration sound can cause a permanent hearing loss not preceded by TTS. It is generally believed that in these instances sound mechanically damages the organ of Corti, tearing membranes, rupturing cells, and allowing perilymph and endolymph to mix. This is contrasted to the gradual loss of stereocilia and hair cells seen in NIHL, preceded by TTS, and usually considered to involve both injury and metabolic repair processes. Acoustic trauma can produce losses that are more severe than those seen with NIHL, especially in the low frequencies. At extreme levels, such as with explosive or blast injury, even tympanic membrane or ossicular injury can occur, causing conductive or mixed hearing loss.
An important type of exposure that can create either NIHL or acoustic trauma is impulse noise (18). Impulses in the range of 0.2-millisecond duration have peak energy at 2 to 3 kHz, are therefore especially hazardous to human hearing, and are typical of small arms gunfire. Such impulses, when above a critical level of 140 decibels (dB) (peak), are considered potentially hazardous to human hearing (19).
Many industrial environments contain large amounts of impact noise, usually caused by collision of metal objects. These noises have high peaks and are often reverberant as well. Intense impact noises can cause acoustic trauma, but they are less likely than impulse noises to reach critical levels.
The levels at which continuous noise and tones (in contrast to impulses and impacts) cause acoustic trauma are not well defined. The earliest cordless telephones rang through the earpiece; if the person answering the phone failed to switch manually from “ring” to “talk” mode, the phone would ring directly into the ear with a 750- to 800-Hz tone at about 140 dB SPL. Dozens of cases of permanent hearing loss occurred from singlering exposures, each probably less than 1 second in duration. At the other extreme, single 4-hour exposures below 110 dBA probably pose negligible risk of acoustic trauma (20).
Interactions
Aging
The nature of the interaction between NIPTS and age-related hearing changes has been the subject of considerable debate. Epidemiologic studies, such as those illustrated in Figure 157.2, usually assume additivity; that is, the net hearing loss is the decibel sum of the threshold shifts from aging and from noise. When this question has been addressed explicitly, the bulk of evidence favors simple additivity. For example, Macrae (21) showed that
war veterans with NIHL developed about the expected amount of additional hearing loss, based on studies of agerelated hearing loss, over the ensuing years. The American National Standards Institute (ANSI) supports the theory of additivity, with a small correction factor to be used when the total hearing loss exceeds 40 dB hearing loss (22). Recent federal audiometric surveys, like those in earlier decades, show that age-related hearing loss disproportionately affects higher frequencies, especially in men; varies markedly across individuals; and accelerates in middle age (23,24).
war veterans with NIHL developed about the expected amount of additional hearing loss, based on studies of agerelated hearing loss, over the ensuing years. The American National Standards Institute (ANSI) supports the theory of additivity, with a small correction factor to be used when the total hearing loss exceeds 40 dB hearing loss (22). Recent federal audiometric surveys, like those in earlier decades, show that age-related hearing loss disproportionately affects higher frequencies, especially in men; varies markedly across individuals; and accelerates in middle age (23,24).
Vibration
Czanto and Ligia (25) are among those who have shown that workers who use vibrating handheld tools, such as pneumatic hammers, grinders, and chipping tools, and who develop “vibration-induced white finger” also develop excessive NIHL. This could mean that vibration interacts with noise to damage the ear or that people who are susceptible to white finger (a peripheral vascular disorder) are also susceptible to NIHL.
Drugs and Chemicals
Humes (26) reviewed many studies and concluded that no substantial risk of hearing loss occurs from combining a noise exposure and an aminoglycoside drug when neither is present in sufficient amount to cause hearing loss on its own. Naturally, one would be reluctant to combine ototoxic drugs (primarily aminoglycosides and platinumbased antineoplastics) with hazardous noise exposures, but, with the probable exception of the newborn nursery where some borderline noise levels have been recorded, this is not a practical problem. The ototoxic drug most likely to be combined with industrial noise exposure is aspirin, but this causes a reversible hearing loss and has not been shown to potentiate or interact with NIHL.
Carbon monoxide (CO), xylene, styrene, and toluene have been reported to cause sensorineural hearing loss in rats. Workers exposed to toluene or xylene in addition to noise developed more hearing loss than those exposed to noise alone (27,28). Exposures in many of these studies were undocumented or were above Occupational Safety and Health Administration (OSHA) permissible time-weighted
average (TWA) exposures, raising the question of whether exposures below OSHA limits could cause hearing loss, either alone or by interacting with occupational noise. One study (29) showed that styrene exposure below currently recommended values, plus noise, was associated with more hearing loss than noise exposure alone. Another showed that mixed solvent exposures, almost all below OSHA permissible levels, were associated with slightly elevated progression of high-frequency hearing loss (30).
average (TWA) exposures, raising the question of whether exposures below OSHA limits could cause hearing loss, either alone or by interacting with occupational noise. One study (29) showed that styrene exposure below currently recommended values, plus noise, was associated with more hearing loss than noise exposure alone. Another showed that mixed solvent exposures, almost all below OSHA permissible levels, were associated with slightly elevated progression of high-frequency hearing loss (30).
Susceptibility
Persons vary widely in the degree of TTS or PTS caused by noise exposures. Efforts to predict, measure, or explain these differences in susceptibility have generally been fruitless in humans. Men often display more hearing loss in noisy occupations than do women, but this may be due to different nonoccupational exposures (especially shooting) between the genders. Mice inherit susceptibility to both NIHL and age-related hearing loss (31,32); genetic tests (33) or tests of efferent function (10) may someday permit prediction of human susceptibility to NIHL. In most experimental animals, there is a critical period in infancy, about the time of final maturation and innervation of the hair cells, in which susceptibility to NIHL is greater than at other times (34). Whether this happens in human infants is unclear.
Otoacoustic emissions (OAEs) are predictably reduced or absent in NIHL. In military studies of young men with excellent pre-noise hearing thresholds, those with baseline-reduced OAE amplitudes (of unknown etiology) were more likely to experience substantial pure-tone threshold shifts after either continuous (35) or impulsive (36) noise exposure. However, changes in OAE amplitude have not been shown to predict—or even to correlate with— audiometric threshold shifts.
Pathophysiology and Pathogenesis
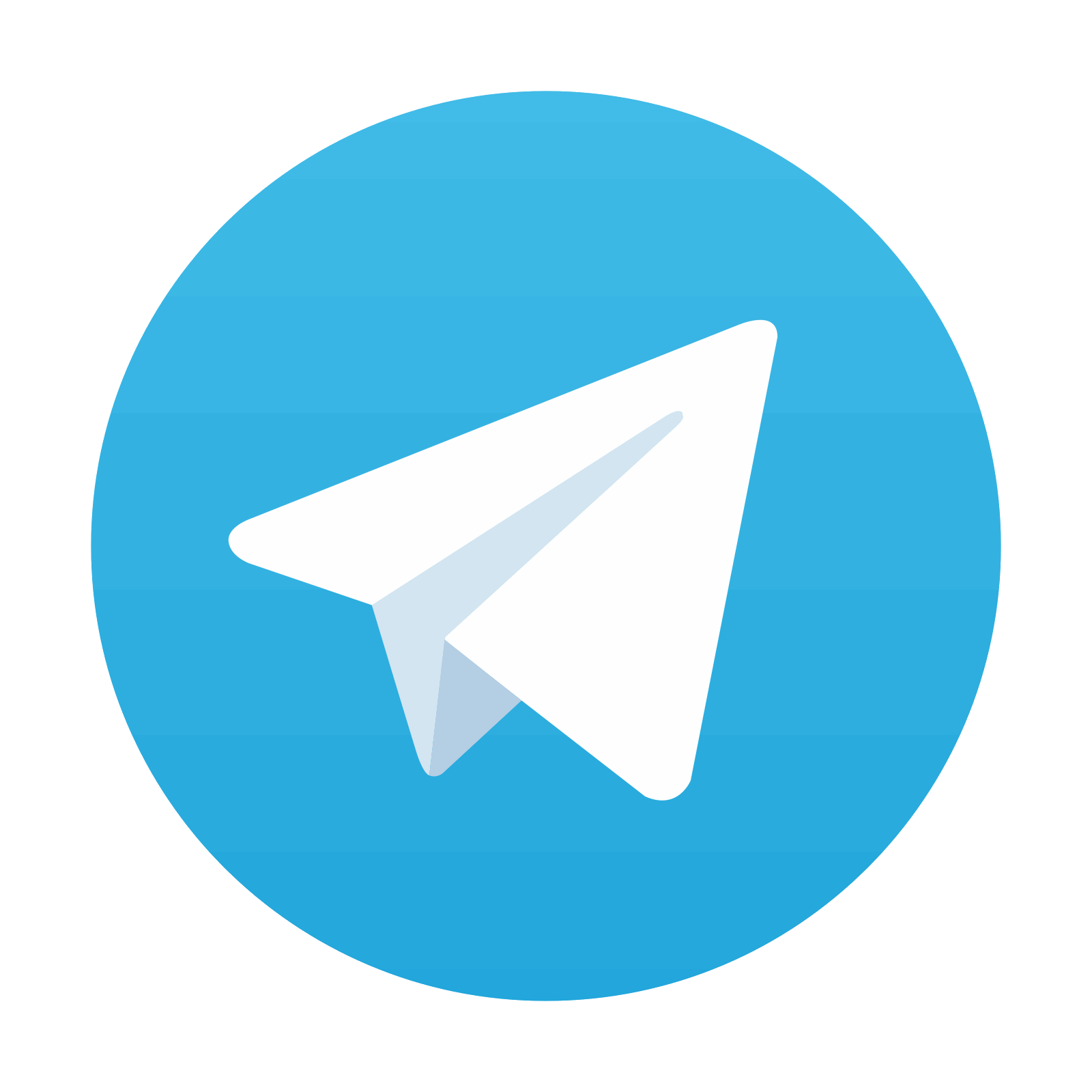
Stay updated, free articles. Join our Telegram channel

Full access? Get Clinical Tree
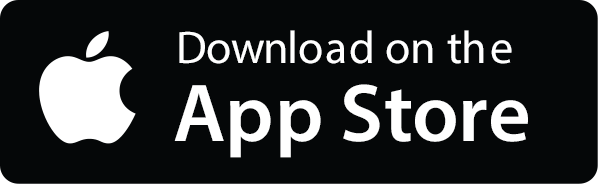
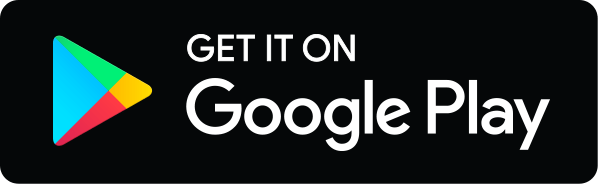