14 Neuro-Ophthalmologic Considerations
A neuro-ophthalmologist is an essential member of the team of physicians caring for patients with pituitary lesions, along with a neurosurgeon, a neurologist, a radiologist, and an endocrinologist. The neuro-ophthalmologist quantifies and evaluates the impact of sellar lesions on the afferent and efferent visual pathways, helps to determine the tempo of tumor growth, and monitors the success and possible complications of treatment. In cases where vision loss or blurred or double vision is the presenting symptom, the neuro-ophthalmologist may be the first to recognize a pituitary lesion. In previously diagnosed cases, the neuro-ophthalmologist’s role is to follow sequential visual fields and determine progression or stability of defects. By supplying information about functioning in the visual pathway, the neuro-ophthalmologist influences decisions about the modality and timing of treatment.
Pituitary adenomas are among the most common lesions in the head, with small ones found at autopsy in a quarter of otherwise normal individuals. Therefore, most pituitary adenomas do not have neuro-ophthalmologic symptoms. Pituitary adenomas greater than 1 cm in height are likely to cause symptoms by compressing the anterior visual pathway or the nerves controlling ocular motility. A similar syndrome of visual loss can be caused by craniopharyngiomas, meningiomas, or gliomas. Information supplied by the neuro-ophthalmologist may move the team toward a therapeutic decision.
This chapter begins with a description of the structures surrounding the pituitary, including the afferent visual pathways and the cavernous sinus, and how their anatomy is affected by pituitary lesions. It goes on to consider various neuro-ophthalmologic presentations of pituitary lesions, especially visual field loss. It outlines indications for treatment, with special attention to pituitary apoplexy, hypophysitis, and pregnancy. After discussing visual recovery, complications of treatment, and follow-up, the chapter concludes with a note on the place of neuro-ophthalmology in the care of patients with pituitary adenomas.
Anatomical Considerations
The Afferent Visual Pathway
The pituitary sets in the sella turcica just above the sphenoid sinus and between the paired cavernous sinuses. The proximity of the pituitary to the anterior visual pathway and to the cavernous sinuses makes knowledge of their anatomy critical. Axons of the retinal ganglion cells destined to synapse in the dorsal lateral geniculate nuclei pass through the optic nerves, optic chiasm, and optic tracts to reach their termination. Each eye provides information about both hemifields, that is, both the right and left halves of visual space, which are distributed at the optic chiasm. Within the optic nerve, axons originating in the temporal retina remain temporal and subserve the contralateral visual hemifield. Axons from the nasal retina subserve the temporal visual field. Axons from retinal ganglion cells in the superior retina are located superiorly in the optic nerve, optic tract, and cerebral cortex, and subserve vision in the inferior hemifield. More than half of the 1.1 to 1.3 million axons in each human optic nerve decussate in the optic chiasm. Axons from the nasal half of the right eye carry information about the right half of visual space and decussate in the optic chiasm to join the left optic tract, and axons from the temporal half of the right eye that carry information about the left half of space remain on the right, to be joined by the nasal axons crossing from the left eye. Hemidecussation allows a neat topographical map of visual space to be projected on the various visual brain centers and allows precise localization of lesions along the pathway from the retina to the occipital cortex. Figure 14.1 shows a retinotopic map of the visual system.
The optic nerves are considered “cranial nerve II,” even though they are embryologically and histologically white matter tracts of the brain and their axons are myelinated by oligodendrocytes like other central fibers. In each eye, axons of retinal ganglion cells run in the nerve fiber layer to reach the optic disk and then pass through the lamina cribrosa, a collection of windows through the posterior sclera of the eye, which is continuous with the dura mater of the brain. Just behind the eye, the axons become myelinated and the optic nerve reaches its greatest diameter of 4 mm, which declines to 3.5 mm posteriorly. After traversing the orbit, the optic nerve passes through the optic canal, where its dural sheath is fused to the wall of the canal, rendering it particularly susceptible to traumatic injury. In 4% of patients, a defect in the wall of the canal exposes the optic nerve to the sphenoid sinus.1 The intracranial portion of the optic nerve passes beneath the falciform ligament, along the planum sphenoidale, and turns superiorly and medially to form the optic chiasm. This intracranial segment is the most variable portion of the nerve, with an average length of 10 mm but commonly ranging from 5 to 15 mm, depending on the position of the optic chiasm. In 25% of patients the optic nerves follow such an upturned course that the incident angle with the chiasm is acute.2
Fig. 14.1 A simplified representation of a clown throughout the visual pathways. First, retinotopic representation (R) is inverted and reversed as light rays cross through the lens. Second, in the optic nerves (ON), reversed and inverted representation is maintained. Third, in the optic chiasm (OC), fibers carrying temporal information cross, whereas fibers carrying nasal information remain ipsilateral. Fibers carrying superior temporal information cross anteriorly in the chiasm (purple path), while those carrying inferior temporal information cross posteriorly (aqua path). Fourth, the optic tracts (OT) carry information from the contralateral visual field. Note that orientation changes as superior fibers (inferior field) move medially and inferior fibers (superior field) move laterally. Fifth, in the lateral geniculate nucleus (LGN), fibers synapse within six layers. Fibers originating in the contralateral eye synapse in layers 1, 4, and 6 (orange), whereas fibers from ipsilateral eye synapse in layers 2, 3, and 5 (lavender). Sixth, optic radiation (OR) carries the inferior half of the contralateral field through the parietal lobe. Seventh, Meyer’s loop (ML) carries the superior contralateral field through the temporal lobe. Eighth, in the occipital (striate) cortex (V1), each side receives the contralateral field. Representation of the clown’s face is shown overlying the two halves of the cortex, which is shown in two axial slices, one superior and one inferior to the calcarine fissure. Macular information (the purple nose) is disproportionately represented at the occipital tip. Ninth, the clown’s ears are contained within the temporal crescent, which is only seen by the ipsilateral eye. Its representation is in the contralateral occipital cortex, anteriorly and medially. Finally, consensual pupillary reactions are shown. Some fibers (light green on the left, red on the right) peel off the optic tracts and synapse in the pretectal nuclei (PTN). From each PTN, fibers reach the ipsilateral Edinger Westphal (EW) nucleus, a subnucleus of the oculomotor nuclear complex where they synapse with parasympathetic fibers of the oculomotor nerve (III). These fibers then synapse in the ciliary ganglion (CG) with postganglionic fibers that travel to the iris sphincter muscle, which constricts the pupil. From each PTN, fibers also travel to the contralateral EW, thereby ensuring consensual constriction of the contralateral pupil.
The optic chiasm is named for the Greek letter Chi (τττ), but its shape more closely resembles the letter H. The dimensions of the chiasm are roughly 12 to 13 mm wide, 3 to 4 mm high, and 8 mm long, varying in size depending on the individual and the method of measurement. In the human brain, slightly more than half (53%) of the axons decussate in the optic chiasm. Nondecussating axons travel in a lateral position through the chiasm. Decussating axons cross left to right or right to left, arranged in parallel bundles across the cross-bar of the “H.”
The location of the chiasm relative to the pituitary and pituitary stalk can vary. In approximately 80% of patients the optic chiasm is located directly above the pituitary. However, in 5 to 10% of the population, the chiasm is pre-fixed, meaning that it lies anterior to the pituitary adjacent to the posterior optic nerves, and in 11 to 15% it is post-fixed, lying above the dorsum sella, not far from the optic tracts. This is diagrammed in Fig. 14.2.
The optic chiasm lies an average of 10 mm above the pituitary, but in 14% of patients this distance may be as little as 2 mm.2 Axons from the macula, the central region of the retina, cross in the posterior portion of the chiasm, whereas axons from the periphery cross more anteriorly.
From the optic chiasm, the optic tracts run posteriorly, superiorly, and laterally, passing between the anterior perforated substance and the tuber cinereum. After curving around the cerebral peduncle, each optic tract gives off the retinal ganglion cell axons that subserve the pupillary light reflex. These axons leave the optic tract and pass medially in the brachium of the superior colliculus to synapse in the pretectum. Other populations of retinal ganglion cells project to the superior colliculus and to the accessory optic system. Nerve fibers responsible for entrainment of circadian rhythms to ambient light come off the dorsal optic chiasm and project into the hypothalamus. The remaining 90% of retinal ganglion cell axons carry visual information to the lateral geniculate nucleus (LGN). The retinotopic organization of axons is maintained in the optic tracts but is rotated in the lateral geniculate such that superior ganglion cell fibers are found more medially and inferior fibers more laterally. The LGN has six cellular layers, with contralateral fibers synapsing in layers 1, 4, and 6, and ipsilateral fibers synapsing in layers 2, 3, and 5 (Fig. 14.1).
Neurons of the LGN give off axons that ultimately synapse in the primary visual cortex (V1) of the occipital lobe. Fibers transferring inferior visual field information from the superior retinal ganglion cells travel through the parietal lobe and synapse superior to the calcarine fissure that divides the occipital cortex in two. In contrast, fibers subserving the superior field travel anteriorly in the temporal lobe and then posteriorly back to the inferior occipital cortex through a bundle of fibers called Meyer’s loop. From the primary visual cortex, information is passed to visual processing areas that are concerned with object recognition (the temporal stream) and visuospatial mapping (the parietal stream).
Fig. 14.2 Variable anatomy of the chiasm determines type of visual loss from pituitary tumors. (A) The chiasm is pre-fixed, above the tuberculum sellae, placing the optic tracts in the line of an expanding pituitary tumor. Field loss may include a homonymous hemianopia. (B) The optic chiasm is normal, lying above the diaphragma sella and the pituitary gland. Typical field loss from an expanding pituitary tumor would be bitemporal hemianopia. (C) The chiasm is post-fixed above the dorsum sellae. The pituitary lies below the optic nerves, so that monocular field defects (cecocentral, arcuate, and altitudinal) result. Note that the angle of the infundibulum varies accordingly. AC, anterior clinoid process; C, optic chiasm; DS, dorsum sellae; In, infundibulum; N, optic nerves; T, optic tracts; TS, tuberculum sellae.
Blood Supply
The blood supply to the anterior visual system is provided by an extensive pial network on its surface, derived from the carotid artery and its branches. The intracanalicular optic nerve receives blood from the ophthalmic artery, with some of its short branches, the posterior ciliary arteries, meeting to form an anastomosis around the anterior optic nerve called the circle of Zinn-Haller. The intracranial portion of the nerve is fed from the internal carotid, anterior cerebral, and anterior communicating arteries, with some recurrent branches from the ophthalmic artery. Some authors have found major input from the anterior superior hypophyseal artery.3,4
The blood supply to the optic chiasm is complex.1,3–5 The inferior aspect is fed by the anterior superior hypophyseal and posterior communicating arteries. The lateral aspect receives blood directly from the ophthalmic (C6) segment of the internal carotid. The superior aspect is fed mainly from the anterior cerebral and anterior communicating arteries. The rich anastomoses among these arteries account for the rarity of ischemic lesions of the optic chiasm. The crossing macular fibers in the central chiasm are susceptible to compression because once the pituitary expands more than about a centimeter, the chiasm begins to be compressed.
The anterior optic tract is fed by a continuation of the chiasmal pial plexus. Posterior to that, its main contributor is the anterior choroidal artery. The lateral geniculate nucleus has a dual blood supply; the anterior aspect is fed by the anterior choroidal artery (arising from the internal carotid), whereas the posterior aspect is fed by the lateral choroidal artery, a tributary of the posterior cerebral artery. Ischemia to the anterior choroidal artery leads to contralateral sectoral defects superiorly and inferiorly, sparing a central zone, whereas the converse is true for lateral choroidal artery infarcts.
Efferent Pathways: Cavernous Sinus
The cavernous sinus is a rete or plexus of blood vessels surrounding the carotid artery on either side of the pituitary fossa. A diagram of its structures is shown in Fig. 14.3.
Cranial nerves responsible for eye movements and pupillary responses to light pass through the cavernous sinuses on their way to the orbit. Expanding pituitary lesions can lead to compression or hemorrhage into the cavernous sinus on either side, resulting in ophthalmoplegia.
The oculomotor nerve (cranial nerve III) is the motor nerve most frequently involved by pituitary adenomas. It exits the midbrain ventrally in the interpeduncular fossa, passing between the posterior cerebral and superior cerebellar arteries, and runs parallel and just lateral to the posterior communicating artery, to reach the cavernous sinus. Fibers controlling pupillary constriction to light are situated together in a bundle on the medial surface of the nerve, in a position where they are vulnerable to compression by a posterior communicating artery aneurysm. In contrast, ischemic third nerve palsies tend to spare this bundle. Just behind the cavernous sinus, the oculomotor nerve travels through the triangle of dura composed of the interclinoid ligament and the anterior and posterior petroclinoidal folds. It pierces the dura anterior and lateral to the posterior clinoid process and then passes within the lateral wall of the cavernous sinus. The oculomotor nerve is organized into two divisions: the superior division controls the levator palpebrae and superior rectus muscles, whereas the inferior division controls the medial rectus, inferior rectus, inferior oblique, and iris sphincter muscles. At the anterior end of the cavernous sinus, the two divisions pass just inferior to the anterior clinoid process before exiting the skull through the superior orbital fissure.
Fig. 14.3 The cavernous sinus (blue) is collection of venous pockets that drain blood from the sphenoparietal veins, superficial middle cerebral veins, and the superior and inferior ophthalmic veins. Blood exits to the petrosal sinus and emissary veins. It is the only location in the body where an artery, in this case the carotid artery (CA), is completely surrounded by a vein. It is also traversed by cranial nerves III, IV, V1, V2, and VI, the latter of which can be seen adjacent to the CA. The sympathetic fibers traveling toward the eye are near the abducens nerve and, in some studies, form a plexus around it. V3 travels through Meckel’s cave (MC), which lies inferior to the sinus. The pituitary gland sits in between the two halves of the sinus, whereas the chiasm sits above. OC, optic chiasm; MC, Meckel’s cave; Pit, pituitary gland; SS, sphenoid sinus; Sym, sympathetic nerves in plexus around VI; III, oculomotor nerve; IV, trochlear nerve; V1, ophthalmic branch of trigeminal nerve; V2, maxillary branch of trigeminal nerve; V3, mandibular branch of trigeminal nerve; VI, abducens nerve.
The trochlear nerve (cranial nerve IV) exits the midbrain dorsally just behind the inferior colliculus. The paired nerves cross in the anterior medullary velum, and each passes around the brainstem within the subarachnoid space. After piercing the dura to enter the cavernous sinus just inferior to the oculomotor nerve, each trochlear nerve runs in the wall of the cavernous sinus parallel to and just below the third nerve.
The abducens nerve (cranial nerve VI) is the only ocular motor nerve to run through the substance of the cavernous sinus rather than in its wall and is located between the trigeminal nerve and the intracavernous carotid. Theoretically, this makes it more susceptible to compression from the lateral wall of the sella turcica. The sympathetic fibers responsible for dilation of the pupil and widening of the palpebral fissure also pass through the cavernous sinus. They enter around the internal carotid artery, “hitchhike” briefly on the abducens nerve, and exit the cavernous sinus with the ophthalmic nerve. This accounts for the co-occurrence of abducens paresis and oculosympathetic defects with lesions in the cavernous sinus. Behind and inferior to the cavernous sinus, in Meckel’s cave, the trigeminal ganglion gives off its third (mandibular) branch into the foramen ovale, leaving only the first and second divisions of the trigeminal (ophthalmic and maxillary branches) to pass through the cavernous sinus. Both divisions lie in the wall of the cavernous sinus, inferior to the trochlear nerve. The maxillary branch leaves the skull through the foramen rotundum, and the ophthalmic branch continues on in the wall of the cavernous sinus and exits through the superior orbital fissure.
Neuro-Ophthalmologic Presentations of Pituitary Lesions
Presenting Signs and Symptoms
Pituitary lesions can present with specific symptoms or can be discovered incidentally, for example, when neuroimaging is performed after unrelated trauma or head pain as in the case presented in Fig. 14.4A. Although magnetic resonance imaging (MRI) or computed tomography (CT) can indicate that a pituitary mass is of sufficient size to be in contact with the optic chiasm or the cavernous sinuses, only careful clinical examination can determine the compressive effect of the mass on the visual system. A full neuro-ophthalmologic exam should be performed, including visual acuity, color vision, stereoacuity, visual fields, pupil response, evaluation of eye movements (especially for subtle motility disturbances or nystagmus), and assessment of the optic disk for pallor and patterns of nerve fiber layer dropout.
Fig. 14.4 Bitemporal defects due to chiasmal compression. (A) Inearly compression from below, superior bitemporal defects may be seen. Note that the defects hug the vertical meridian. (B) In more advanced compression, the superior fibers have been affected as well, and a bitemporal hemianopia has evolved.
Bitemporal Hemianopia
The classic ophthalmologic presentation of a pituitary tumor is a bitemporal hemianopia caused by compression of the crossing axons in the optic chiasm. The superior quadrants of the temporal visual field typically are affected first, corresponding to compression of the optic chiasm from below. However, depending on the patient’s underlying anatomy and the growth pattern of the lesion, other loci of visual field loss are possible. A post-fixed optic chiasm is likely to produce monocular retinal nerve fiber layer defects from optic nerve compression, and an ipsilateral relative afferent pupillary defect (RAPD). A pre-fixed chiasm is likely to produce a homonymous hemianopia resulting from compression of the optic tract along with a contralateral RAPD.
The visual field has been described as an “island of vision surrounded by a sea of darkness”6 because sensitivity to stimuli is greatest in the center at fixation and then declines toward the periphery until complete darkness is reached. Therefore, an object must be larger or brighter to be seen in the periphery than at fixation. A defect in the visual field can be thought of as part of this island sinking into darkness. Two approaches can be taken to map out a visual field defect: kinetic, in which a test object of specified size and luminance is moved from the periphery to the center until it is perceived; and static, in which a stationary stimulus is presented with increasing brightness until it reaches the threshold of perception. Formal kinetic perimetry is performed using either a tangent screen, which is a wall-mounted flat screen against which the examiner moves standardized test objects from the periphery to the center, or a Goldmann perimeter, which is a bowl-shaped background of uniform luminance against which a pantograph projects spots of different size, luminance, and color. In Goldmann perimetry, a peephole in the center of the bowl allows the examiner to monitor the patient’s fixation while controlling the presentation of stimuli. For both Goldmann and tangent screen methods, the result is a series of isopters or concentric topographical curves, representing the distance from fixation at which the patient became aware of test objects.
Static perimetry has largely replaced kinetic perimetry as the standard method for obtaining visual fields, and usually is performed on a Humphrey visual field analyzer. The Humphrey analyzer consists of a similar bowl-shaped background of set luminance, and tests vision by having the patient fixate on a central target and press a button when a stimulus is perceived. The Humphrey machine offers a variety of standardized strategies that differ in the extent of grid tested, and the color, size, and luminance of the test object or background. The most common test, the 24–2 threshold test, obtains sensitivity at points within 24 degrees of fixation; the “dash 2” signifies that the grid is arranged with test points not on the axes but rather straddling the vertical and horizontal meridians, providing more value in localizing pathology. A “full-threshold” test, where sensitivity is determined at numerous points within the test grid, can be a grueling ordeal, requiring up to 30 minutes per eye. The Swedish Interactive Threshold Algorithm (SITA) was developed to shorten the testing time by eliminating empirically redundant tests. SITA Standard testing takes up to 15 minutes per eye for a 24–2 field. The more recently developed SITA Fast strategy is even more efficient, taking 3 to 5 minutes per eye. All Humphrey threshold strategies include internal controls for fixation and for false-positive and false-negative errors. The machine is automated, so that while a human operator is required to set up the test, monitoring of the patient, presentation of stimuli, and data processing are performed by the machine.
The typical visual field heralding a pituitary tumor—the bitemporal hemianopia—may be demonstrated by either static perimetry (Fig. 14.4) or by kinetic perimetry (Fig. 14.5).
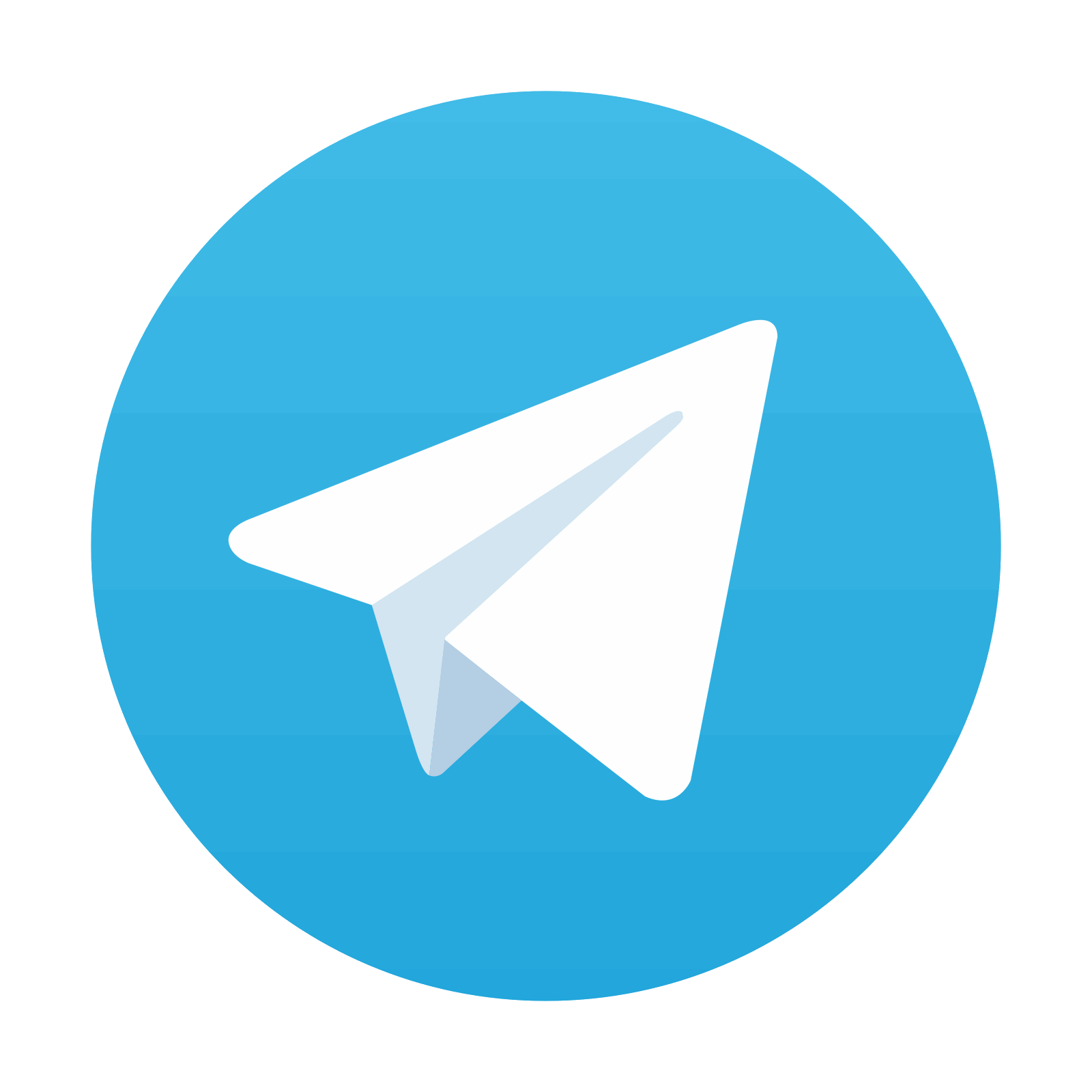
Stay updated, free articles. Join our Telegram channel

Full access? Get Clinical Tree
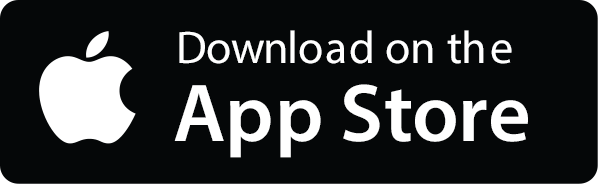
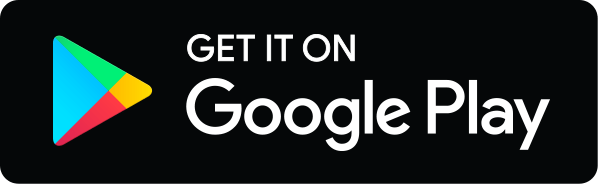