Fig. 37.1
Potential mechanisms for interaction between the upper and lower airways: (1) loss of nasal conditioning function, (2) direct passage of inflammatory mediators and/or microorganisms between the upper and lower airways, (3) nasobronchial reflexes, (4) stimulation at one point of the respiratory mucosal surface results in a pan-airway inflammatory response, (5) olfactory regulation of respiration, and (6) aerocrine messengers (CO2 and NO)
Nasal breathing improves arterial oxygen concentrations and carbon dioxide excretion from the lungs.
37.2 Physiological Interactions
The nose has an important role in filtering, warming, and humidifying inspired air before inhalation into the lungs. The nose also provides a resistance to both inspiration and expiration that is twice that of the open mouth. This increased resistance appears to have a number of physiological benefits. In a study of arterial oxygen levels (PaO2) before and after jaw wiring, which forced patients to breathe continuously through their noses, PaO2 increased by nearly 10 % (Swift et al. 1988). Nasal packing after nasal surgery forcing patients to breathe through their mouths is associated with a reduction in arterial O2 saturation (Ogretmenoglu et al. 2002). At rest, end-tidal CO2 levels in expired air, which are considered to be a reliable indirect measure of CO2 levels in the arterial blood, increase with nasal breathing indicating that nasal breathing improves the efficiency of CO2 excretion from the lungs (Tanaka et al. 1988). During exercise, nasal breathing reduces the fraction of expired oxygen (FEO2), indicating that on expiration the percentage of O2 extracted from the air by the lungs is increased, and increases the fraction of expired carbon dioxide (FECO2), indicating an increase in the percentage of expired air that is CO2 (Morton et al. 1995). This equates to more efficient O2 extraction and CO2 excretion during exercise.
Explanations for these observations still remain largely hypothetical. Nasal breathing increases total lung volume (Swift et al. 1988). The corresponding increase in functional residual capacity (volume of air present in the lungs present after passive expiration) is thought to improve gas exchange leading to improved PaO2. NO derived from the nose and sinuses might also improve ventilation-perfusion relationships in the lungs (Della Rocca and Coccia 2005). The nose also provides an inspiratory resistance forcing the diaphragm to contract against a resistance. On a long-term basis, this might also have an important role in maintaining diaphragmatic muscle strength, although the role of inspiratory muscle training in a range of lung diseases continues to be debated (Padula and Yeaw 2007; Gosselink et al. 2011). Regardless, nasal breathing appears important in aiding O2 absorption and in facilitating CO2 excretion in the lungs.
Nasal breathing significantly improves oxygen extraction and carbon dioxide excretion during exercise.
The breathing cycle is divided into inspiratory and expiratory phases. When the respiratory rate increases, the expiratory phase shortens. Nasal breathing slows the respiratory rate increasing the length of the expiratory phase (Ayoub et al. 1997). Increasing the expiratory phase of the respiratory cycle is known to increase the body’s relaxation response (Cappo and Holmes 1984). A number of ancient disciplines, such as yoga and tai chi, emphasize the importance of nasal breathing in relaxation and meditation.
37.3 Respiratory Inflammation
Upper and lower airway inflammatory processes often coexist and share common pathogenic mechanisms (Selimoglu 2005; Hare et al. 2010; Marple 2010). Based on the predominant cell type, chronic rhinosinusitis (CRS) has been classified as being either eosinophilic or neutrophilic (Meltzer and Hamilos 2011). The eosinophilic group includes CRS with polyps, a subset of CRS without polyps, aspirin hypersensitivity, asthma and nasal polyps (Samter’s triad), and allergic fungal rhinosinusitis. Eosinophilic CRS and allergic rhinitis are associated with asthma (Marple 2010). Lower airway inflammation has also been classified according to the cell profile of induced sputum as being either eosinophilic or non-eosinophilic (Hargreave 2007). In asthma patients, eosinophils are the dominant inflammatory cells in middle meatal lavage (Ragab et al. 2005). In small airway disease patients, neutrophils are the dominant inflammatory cells in middle meatal lavage (Ragab et al. 2005).
Asthma and allergic rhinitis are strongly interrelated (Corren 1997; Krouse et al. 2007; Marple 2010). Corren reported nasal symptoms in 78 % of asthmatic patients and that 38 % of allergic and nonallergic rhinitis patients have asthma (Corren 1997). The severity of asthma symptoms correlates closely with rhinitis symptoms (Krouse et al. 2007). The presence of allergic rhinitis also increases the risk of subsequent asthma development nearly fourfold (Shaaban et al. 2008).
Asthma has also been associated with CRS. The prevalence of asthma is 20 % in CRS patients, which is higher than that seen in the general population (Jani and Hamilos 2005). Asthma severity also correlates with CRS disease severity, as determined by computed tomography (Bresciani et al. 2001). In patients having functional endoscopic sinus surgery, the asthma prevalence is 42 %, rising to 50 % in those with nasal polyps (Senior et al. 1999).
Neutrophilic inflammation is a feature of both chronic obstructive pulmonary disease (COPD) and bronchiectasis (Hargreave 2007). While tissue eosinophilia is an established feature of asthma, a neutrophilic picture can also be seen (Hargreave 2007). In COPD patients, inflammatory cells are found both in the sputum and in lung biopsy specimens (Hurst 2009). COPD patients commonly report nasal symptoms, the most common of which is rhinorrhea (Hurst et al. 2006). Nasal symptoms are also more common in COPD patients with chronic sputum production (Hurst et al. 2006). Nasal symptoms double the risk over 8 years of patients developing COPD (Nihlén et al. 2008). Increased levels of the neutrophil chemoattractant protein IL-8 are found in the upper airways of COPD patients, when compared to control subjects (Hurst et al. 2006). Upper airway IL-8 concentrations correlate with those in the lower airway, and the concentration at both sites is related to indexes of bacterial colonization (Hurst et al. 2006). Many bronchiectatic patients also have nasal and sinus disease. Most bronchiectasis patients (77 %) meet the diagnostic criteria for CRS with 25 % of bronchiectasis patients having nasal polyps. Bronchiectasis severity also correlates with CRS severity (Guilemany et al. 2009).
Patients with asthma, chronic obstructive pulmonary disease, and bronchiectasis frequently have upper respiratory disease.
37.3.1 Inflammatory Interactions
Under the influence of serum IL-5 and eotaxin, eosinophils are released from the bone marrow into the systemic circulation. Depending on the local expression of a variety of adhesion molecules, cytokines, and chemokines, eosinophils then migrate to inflamed areas. Leukocytes migrate along a chemotactic gradient through the endothelium. Local cells upregulate endothelial adhesion molecules through the release of IL-1β, IL-4, and TNF-α. Leukocyte endothelial adherence is increased. Intercellular adhesion molecule 1, vascular cell adhesion molecule 1, and E-selectin are known endothelial adhesion molecules (Kita 2011). Increased local expression of these adhesion molecules occurs after experimental nasal and bronchial allergen challenge. Experimentally this results in increased eosinophilic allergic inflammation in the nasal and bronchial mucosa (Braunstahl et al. 2001a, b).
Even a single nasal allergen challenge administered to non-asthmatic subjects with seasonal allergy increases blood eosinophil levels and IL-5 in both the upper and lower airways (Corren 1997). Prior nasal stimulation using a nasal provocation antigen challenge increases bronchial hyperresponsiveness to methacholine challenge (Bonay et al. 2006). Similarly segmental bronchial provocation in patients with allergic rhinitis induces blood eosinophilia and mucosal inflammation in both the upper and lower airways. This inflammation is characterized by increased numbers of eosinophils, IL-5+ cells, and eotaxin-positive cells. Local allergen exposure in both the upper and lower airways results in generalized airway inflammation – this would appear to occur through vascular mechanisms.
A high level of similarity in bacterial cultures from the nasopharynx and from bronchoalveolar lavage is seen in children with bronchiectasis and protracted bacterial bronchitis as well as in patients with cystic fibrosis.
37.3.2 Microbial Aspiration
The silent aspiration of nasopharyngeal secretions has been hypothesized as important in relationships between upper and lower respiratory airway disease (Bardin et al. 1990; Kogahara et al. 2009). The possibility that Staphylococcus aureus-derived enterotoxins could also be inhaled into the lower airway has been raised (Hamilos 2000). COPD patients with lower airway bacterial colonization have a higher total nasal bacterial load (Hurst et al. 2005). Children with bronchiectasis have a high nasopharyngeal carriage of Streptococcus pneumoniae nontypable Haemophilus influenzae (NTHi), and Moraxella catarrhalis and lower airway infection by NTHi. A high level of similarity in cultures from the nasopharynx and from bronchoalveolar lavage is seen (Hare et al. 2010). Similar agreement is also seen in children with protracted bacterial bronchitis (Hare et al. 2010) and in patients with cystic fibrosis (Godoy et al. 2011). In the critical care situation, the treatment of upper respiratory disease reduces the risk of ventilator-associated pneumonia (de Smet et al. 2009).
People with a high nasal bacterial load (and associated greater nasal inflammation) appear more likely to pass bacteria into the lower respiratory tract. The evidence that gross aspiration of nasopharyngeal secretions into the lower respiratory airway occurs is controversial. Radionucleotide scanning in humans shows that it does not occur (Bonay et al. 2006); however, animal experiments suggest that it may occur during sleep (Kogahara et al. 2009). The similarity in microbiological cultures between the upper and lower respiratory tract suggests that some transmission occurs. Microaspiration of bacteria and inflammatory mediators could possibly occur, but this has yet to be demonstrated scientifically.
37.3.3 Influence of Upper Respiratory Interventions on the Lower Respiratory Tract
In the majority of trials, the treatment of allergic rhinitis with nasal steroids reduces asthma severity (Stelmach et al. 2005; Krouse et al. 2007). At the end of 3 years, the children treated with immunotherapy for grass and/or birch allergic rhinoconjunctivitis were less likely than the control group to develop asthma (Möller et al. 2002). Similarly, a number of studies have shown that the surgical treatment of CRS helps asthma patients through both an improvement in asthma symptoms and the decreased use of asthma medication (Krouse et al. 2007). Unfortunately these studies have been uncontrolled. There are also no randomized controlled studies that have investigated the effect of CRS medical therapy on asthma (Krouse et al. 2007).
Medical and surgical treatment of upper respiratory disease helps asthmatic patients.
37.4 Nasobronchial Reflexes
Nasobronchial reflexes have also been implicated in the interactions between the upper and lower respiratory airways. Mechanical or chemical stimulation of nasal, tracheal, and laryngeal receptors could produce sneezing, coughing, or bronchoconstriction, thus preventing deeper penetration of allergens or irritants into the airway (Sarin et al. 2006). Unilateral models of nasal provocation show that secretory responses can be measured in both nostrils (Sarin et al. 2006). The mechanism appears to be neural (Sarin et al. 2006).
When asthmatic patients exercise with their noses occluded, a 20 % decline in forced expiratory flow occurs, compared with less than a 5 % reduction among patients allowed to exercise while nose breathing (Shturman-Ellstein et al. 1978). Recent research has shown that bursts of cold air on the nasal mucosa increase nasal resistance. This effect was blocked by anesthetizing the nose or by inhaling atropine, an anticholinergic drug, before the provocation (Fontanari et al. 1996, 1997). Other researchers have not been able to confirm these results (Johansson et al. 2000). The existence of nasobronchial reflexes secondary to inflammatory exposure in the upper respiratory airway remains controversial (Sarin et al. 2006).
37.5 Olfaction and the Limbic System
Evolutionary theory teaches that in primitive life forms, the olfactory brain was probably a layer of cells above the brain stem that registered a smell and then simply categorized it. New layers of the olfactory brain then developed into what was initially called the rhinencephalon (nose brain) or limbic system (Le Doux 2003). Our limbic system coordinates the stress “fight or flight” response. As part of this response, not only does the heart rate increase, but the respiratory rate also goes up; the limbic system is able to override normal pCO2 homeostasis (Plum 1992). The physiological evidence suggests that fragrances such as rosemary and lavender may have direct effects on human memory and mood (Herz 2009). Fragrances such as lavender by influencing the limbic system and the stress response could potentially affect breathing patterns and rate. The pheromone androstadienone when applied to women’s nasovomerine organs slowed both their heart and breathing rates (Grosser et al. 2000).
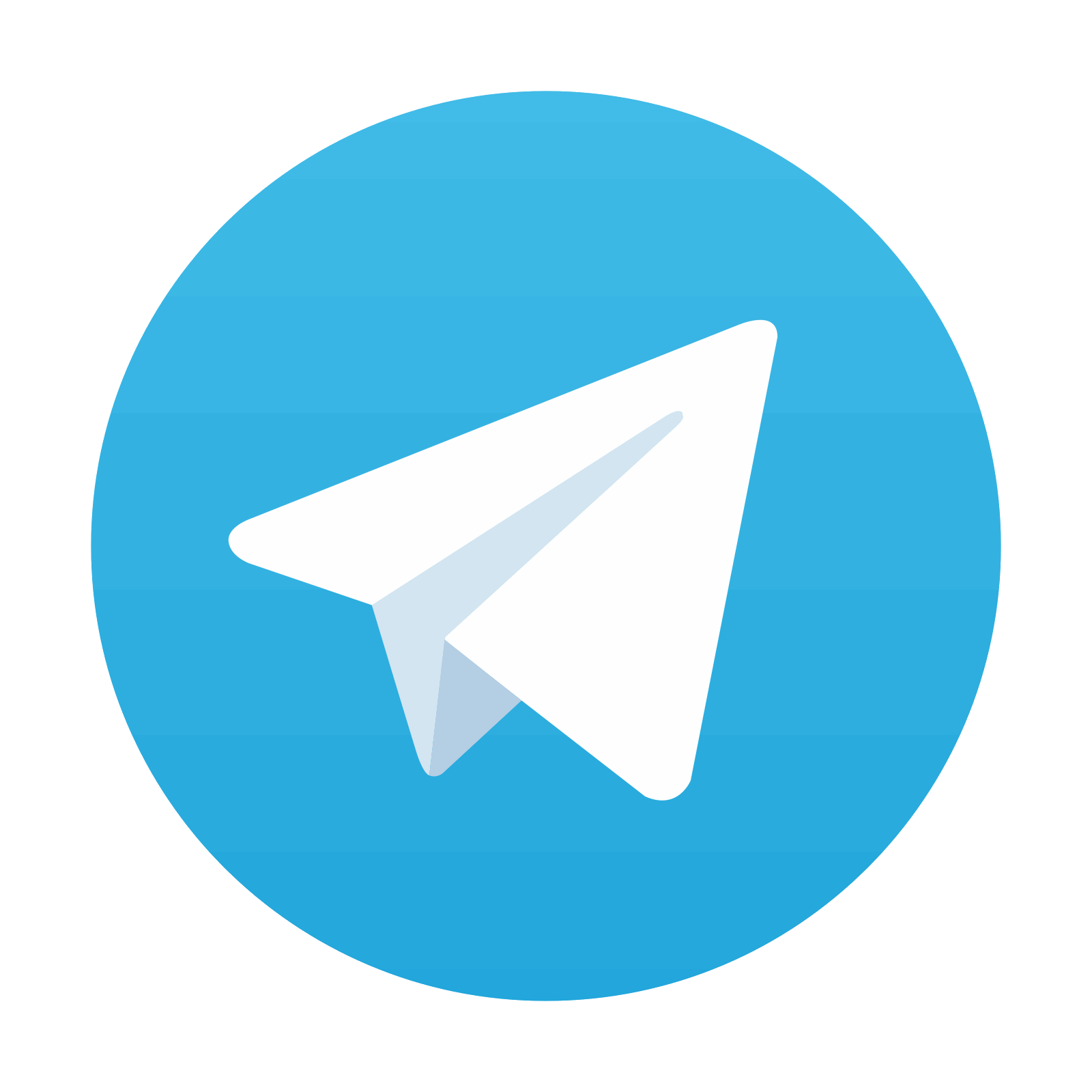
Stay updated, free articles. Join our Telegram channel

Full access? Get Clinical Tree
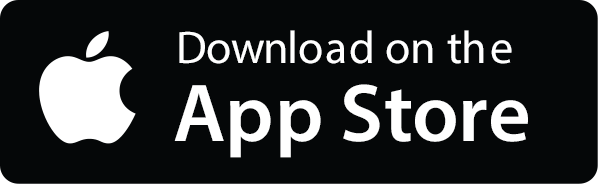
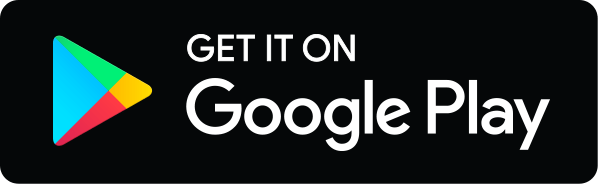