Substance
Common sources
Chemical composition
Activity
Lysozyme
Serum, saliva, tears
Protein
Bacterial cell lysis
Complement
Serum
Protein-carbohydrate lipoprotein complex
Cell death or lysis of bacteria; participates in inflammation
Basic proteins
Serum
Proteins or basic peptides
Disruption of bacterial plasma membrane
Lactoferrin and transferrin
Body secretions, serum epithelial cells
Glycoprotein
Inhibit microbial growth by binding (withholding) iron
Defensin
Epithelial cells, neutrophils
Oligopeptides
Cell death or lysis of bacteria
Peroxidase
Saliva, tissues, neutrophils
Protein
Act with peroxide to cause lethal oxidation of cells
Fibronectin
Serum, mucosal surfaces
Glycoprotein
Clearance of bacteria
Interferons
Virus-infected cells
Protein
Resistance to virus infections
Interleukins
Macrophages, lymphocytes
Protein
Cause fever; promote activation of immune system
29.1.3.1 Defensin
Defensins are a family of evolutionarily related vertebrate antimicrobial peptides with a characteristic beta-sheet-rich fold and a framework of six disulphide-linked cysteines. Two main defensing subfamilies, α- and β-defensins, differ in the length of peptide segments between the six cysteines and the pairing of the cysteines that are connected by disulphide bonds. Defensins are abundant in cells and tissues that are involved in host defense mechanism of mucosal linings such as respiratory and intestinal mucosa. In many species, the highest concentrations of α-defensins are found in granules, the storage organelles of leukocytes (Ganz et al. 1985; Ganz 1987). β-defensins are mainly produced by a variety of epithelial cells such as lung and middle ear (Bals et al. 1998; Moon et al. 2002), and 30 genes from DEFB1 to DEFB136 are currently identified as human β-defensin family. Human β-defensin 1 (DEFB1) is expressed constitutively, whereas β-defensin 2(DEFB4A) is induced by bacterial molecules (Moon et al. 2006; Wehkamp et al. 2004) as well as cytokines (Kao et al. 2004). Paneth cells are another site of high α-defensin concentration and contain defensin-rich secretory granules. Claeys et al. reported that there was no baseline detection of human β-defensin 2 in any sinus mucosal samples, and no upregulation was measured for human β-defensin 2 in paranasal sinus mucosa in patients with chronic sinusitis or nasal polyposis compared with control turbinate mucosa (Claeys et al. 2003). On the other hand, α- and β-defensins were detected in human nasal mucosa by Lee et al. (2002). They examined the expression of defensins in inferior turbinate mucosa of normal subjects and inferior turbinate mucosa and nasal polyps of patients with chronic sinusitis, employing reverse transcription-polymerase chain reaction (RT-PCR) and immunohistochemistry. According to their results, β-defensin 1 mRNA was expressed in all tissue samples. β-defensin 2 mRNA was detected in the turbinate mucosa and nasal polyps of patients with chronic sinusitis, but not in normal mucosa. Its expression level was significantly higher in nasal polyps than in turbinate mucosa. α-Defensin 5 and 6 mRNAs were not expressed in any tissues, but α-defensins 1, 2, and 3 were detected in all tissue samples obtained from patients with chronic sinusitis. These results suggest that β-defensin 1 may play a constitutive role in nasal defenses, whereas α-defensins 1, 2, and 3 and β-defensin 2 may be induced in response to local infection or inflammation.
The average concentration of defensins in these epithelial cells reaches the 10–100 μg/ml range (Harder et al. 1997), but the local concentrations might be higher because of uneven distribution.
Most defensins show antimicrobial activity against bacteria and fungi, especially when tested under low ionic strength conditions (Selsted et al. 1985; Lehrer et al. 1988) and with low concentrations of divalent cations, plasma proteins. It should be taken into consideration that under these optimal conditions, antimicrobial activity is observed at concentrations as low as 1–10 μg/ml (low micromole). Permeabilization of target membrane is the crucial step in defensin-mediated antimicrobial activity and cytotoxicity. It is also reported that defensins act as an immunomodulatory molecules, inducing IL-8 in epithelial cells and modulating complement activation (Van Wetering et al. 1997; Panyutich et al. 1994) and neutrophil apoptosis (Yang et al. 2002; Nagaoka et al. 2008).
29.1.3.2 Defensin Synthesis and Its Regulation
Defensin synthesis and release are regulated by various signals such as microbial signals, developmental signals, and cytokines. Human β-defensin 1 (DEFB1) is expressed constitutively with low level, whereas β-defensin 2(DEFB4A) is highly induced by bacterial molecules (Wehkamp et al. 2004; Kao et al. 2004) as well as cytokines (Claeys et al. 2003). Various signaling pathways are identified to orchestrate β-defensin 2 expression, such as toll/IL-1 receptor (TIR)-dependent NF-κB activation, TIR-dependent MAPK signaling, and NOD-2 dependent NF-κB activation. Wang et al. actually demonstrated that airway epithelia regulate expression of human β-defensin 2 through toll-like receptor 2 (Wang et al. 2003). Vora et al. also proved that human β-defensin 2 expression is regulated by toll-like receptor signaling in intestinal epithelial cells and that LPS and peptidoglycan stimulated β-defensin 2 promoter activation in a TLR4- and TLR2-dependent manner, respectively (Vora et al. 2004).
29.2 Brief Introduction of Toll-Like Receptors in Nasal Epithelial Cells and Signaling Pathway
29.2.1 Distribution of TLRs in Nasopharyngeal Mucosae and Involvement of IL-15 in Inflammation
We employed northern blot assay and RT-PCR to see the TLR distribution (Fig. 29.1) in upper respiratory mucosa and showed that human nasal epithelial cells constitutively expressed mRNA for TLR2, 3, and 6, but not for TLR4 and TLR9 (Fig. 29.2). Lipoprotein used as a pathogen-associated molecular pattern (PAMP) also induced IL-15 production of respiratory epithelial cells, which strictly depend on TLR2 (Fig. 29.3). In Fig. 29.3, in northern blot analysis, IL-15 mRNA was strongly expressed after lipoprotein stimulation. But in contrast, it was not found after lipid A stimulation as a ligand of TLR4. IL-15 concentration in the supernatants of CCL185 was also upregulated after lipoprotein stimulation in a dose-dependent manner. Figure 29.4 showed NF-kB activation of respiratory epithelial cells in response to lipoprotein. In western blot analysis, phosphorylation of IkB-alpha is detected in epithelial cells 15 and 30 min after lipoprotein stimulation. Luciferase assay and DNA-binding assay reveal that NFk-B activity actually correlated with the lipoprotein concentration.
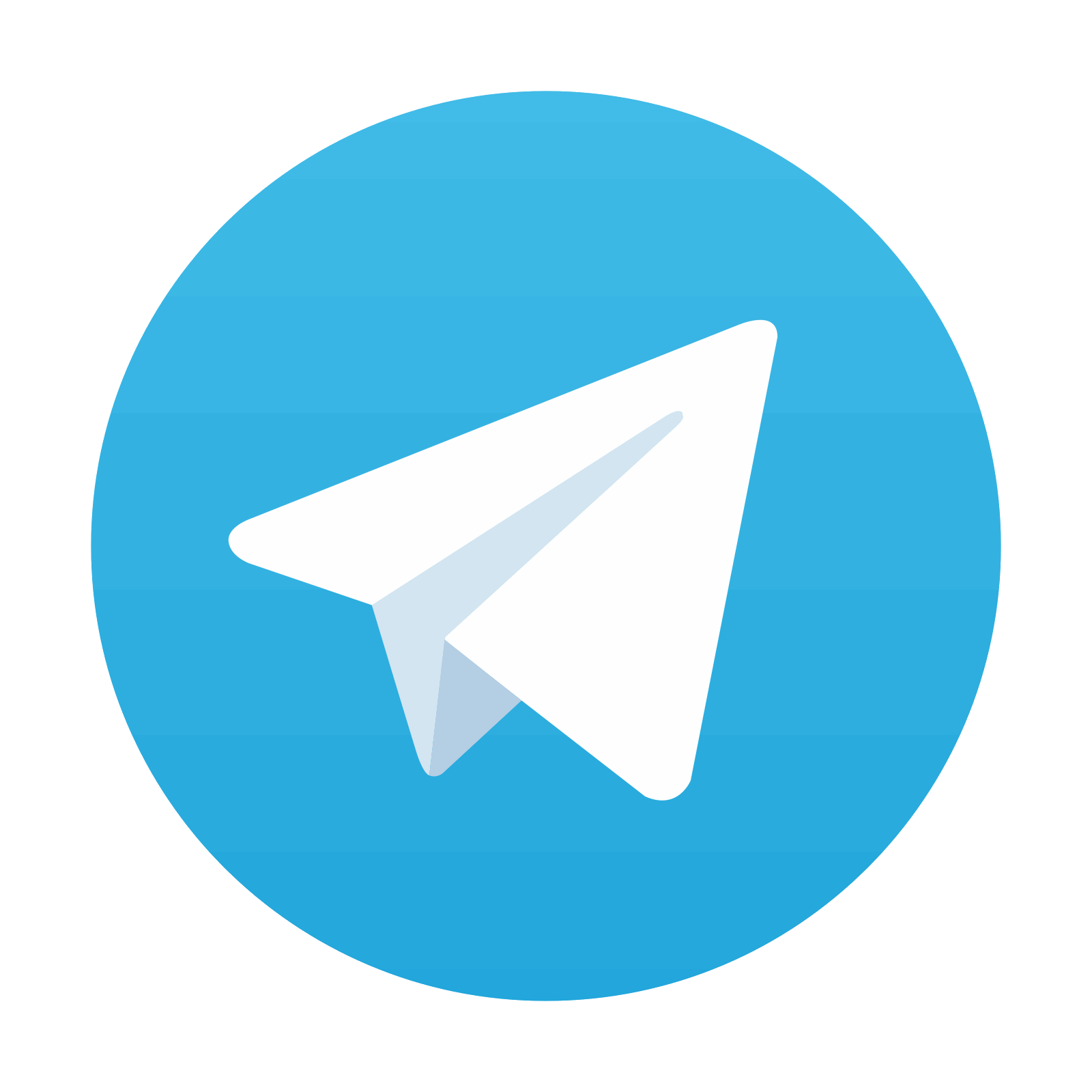
Stay updated, free articles. Join our Telegram channel

Full access? Get Clinical Tree
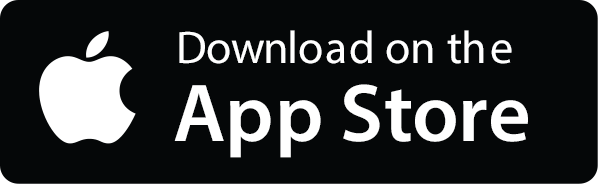
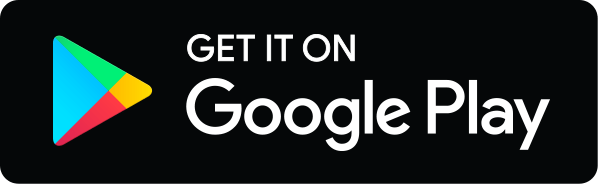