4
Middle Ear Molecular Biology
Allen F. Ryan, Michelle Hernandez, and Stephen I. Wasserman
The process of hearing depends on specialized groups of cells working in concert to deliver acoustic information from the ear to the brain. The function of these specialized cells, in turn, requires the activation of specific sets of genes. In otologic disease states, the expression of genes in the cells of the ear may be altered. In addition, during infection, disease organisms bring their own genes into play. The gene sequences expressed in the ear therefore provide a potential wealth of information regarding normal otologic biology and disease. Methods to recover this information have been developed at a rapid rate, and are now being applied to clinical and basic science problems in otology. Such molecular information can be used for diagnostic purposes, and to increase our understanding of the etiologies of otologic diseases. Moreover, methods with which to manipulate gene expression directly are now under active development, and may in the future translate into gene therapy for use in otology. Molecular biology is also helping to elucidate the basic cellular processes that govern hearing. These processes include the expression of genes involved in middle and inner ear homeostasis, production of proteins unique to the ear, cochlear and vestibular transduction, neurotransmission, responses to inner ear damage, and hair cell regeneration.
This chapter briefly describes the basic methods of molecular biology. The molecular biology of the middle ear (ME) is also explored. We concentrate on immediate and future clinical applications of the basic molecular research being performed today. More specifically, the focus is on molecular diagnostics, pathogen genomes, and vaccine development as well as aspects of host biology that underlie inflammatory responses, tissue hyperplasia, and bacterial resistance in otitis media (OM). Factors that predispose patients to ME infections are also considered. We also describe current work on growth factors that contribute to the healing of tympanic membrane perforations.
Basic Methods in Molecular Biology
A short review of the basic molecular processes that take place within a cell will facilitate an understanding of the information contained in this chapter. One of the most fundamental events that occur within a cell is transcription of a gene, encoded in the genomic DNA of the cell nucleus, into messenger RNA (mRNA). During transcription, a faithful RNA copy of the gene sequence is produced within the nucleus. This copy is then edited to remove segments, called introns, which are not required for the production of protein. The remaining RNA segments, called exons, are spliced together to form the mature mRNA, which is then exported to the cytoplasm for translation. The mRNA is translated into protein by ribosomes, which assemble amino acids sequentially according to the mRNA blueprint. Once the protein is produced, there can be substantial modifications to its structure via posttranslational processing. This can involve cleavage, phosphorylation, the addition of carbohydrate moieties, and other changes that alter the behavior of the protein.
A basic technique of molecular biology is DNA cloning. Segments of DNA can be isolated by digestion with enzymes that cleave the DNA at specific sites (restriction enzymes) and cloned into a variety of self-replicating DNA structures, called vectors, which have been derived by genetic engineering primarily from naturally occurring bacteriophages. When the vectors replicate within bacteria in the laboratory, millions of copies of the inserted DNA sequence are produced, and can then be analyzed.
A second basic method is reverse transcription. Viral enzymes known as reverse transcriptases are capable of producing complementary DNA (cDNA) copies of RNA. When this is applied to mRNA, it allows the rapid and efficient cloning of the mRNA sequences. Because RNA is a relatively ephemeral molecule, cDNAs provide a stable record of the mRNA. Together, reverse transcription and DNA cloning have revolutionized the study of genetics and protein chemistry.
A third method with wide application is the polymerase chain reaction (PCR), which relies on the repeated replication of double-stranded DNA. Through heating, double-stranded DNA separates to form single strands, whose specific sequences can then be replicated. With appropriate primers to recognize the sequence, two identical double-stranded DNA segments are then formed. This process is repeated many times, resulting in exponential amplification of the original DNA sequence. The use of DNA components that fluoresce when integrated into such copies allows the original amount of target DNA to be quantified in a process known as real-time or quantitative PCR (Q-PCR).
Tremendous strides have been made in DNA sequencing technology, which has led to the recent sequencing of not just the human, mouse, and chimp genomes, but also the genomes of many disease organisms. This includes pathogens that contribute to otologic diseases. It has been estimated that the cost of sequencing a human genome will decrease to around $1000 within 10 years, which could make patients’ genome sequence part of their medical record. Rapid sequencing of cDNAs has also allowed us to profile thousands of cDNA sequences expressed by individual tissues and organs, including the inner ear.1 The availability of genome sequences has allowed the development of gene arrays, or chips, which include essentially all genes, permitting rapid and comprehensive profiling of gene expression in tissue samples. Gene expression can be localized at the cellular level using in situ hybridization of mRNA, in which complementary probes are used to label mRNA in tissue sections.
The increase in sequence information has had a tremendous impact on genetics, allowing the identification of an increasing array of unique molecular markers for linkage analysis, a process by which inherited disorders are linked to a particular chromosomal location. The precision of linkage and the complete map of genes in the region have made the identification of disease-causing mutations significantly easier and faster.
In the experimental realm, techniques for engineering the DNA of animals have become commonplace, resulting in the generation of numerous gene deletion (knockout) mice for many genes. Methods to limit gene deletion to specific tissues (conditional knockouts) have allowed the analysis of deletions that may be embryonically lethal. Deletions that can be activated at a given time (inducible knockouts) allow developmental functions to be separated from the role of a gene in the adult. Gene substitution (knock-in) techniques allow animal models of specific human mutations to be created.
Although there have as yet been few applications to patients, gene therapy methods have advanced greatly with the development of improved viral vectors for induced gene expression. They have been used successfully in the ear, most spectacularly in hair cell regeneration.2 In addition, gene silencing through such techniques as short, interference RNA (siRNA) promises to improve the treatment of dominant genetic disorders.
This review of molecular biology is necessarily limited, and is intended only as a brief introduction. For more detailed information on the methods outlined above, and on many other aspects of gene structure and function, many excellent reviews are available.3–5
Molecular Biology of the Middle Ear
Host Responses During Otitis Media
From a molecular perspective, the normal tympanic cavity is a relatively quiescent tissue environment. With the exception of the ME muscles, the activities of the acoustic transmission apparatus are largely passive, and that of the remaining ME tissues are related primarily to homeostasis. Thus, the ME mucosa and eustachian tube maintain the gaseous environment of the ME and maintain a first line of defense against potential infection. However, in disease the ME becomes highly active and exhibits substantial levels of molecular activity. This activity determines the response of the ME to infection, and recent research has generated a substantial amount of new information regarding molecular events during OM. Also, variation in the molecular response to infection is thought to be a major component of differential sensitivity to this disease.
Mucosal Hyperplasia and Recovery
The mucosa that lines the ME cavity can undergo extensive modification during OM, involving changes in the expression of a large number of genes. In response to inflammation, the mucosa changes from a simple, squamous epithelial monolayer to a pseudostratified, respiratory epithelium with many cell layers. This respiratory epithelium has goblet and ciliated cells, and a greatly expanded stroma and vasculature. The hyperplasia of the mucosa is rapid, occurring in a few days, and involves extensive cell proliferation and differentiation. Recovery from OM is characterized by rapid loss of these additional cells, and return to a relatively simple epithelial morphology.
ME mucosal hyperplasia is almost certainly controlled in part by growth factors (GFs) through their interaction with specific transmembrane receptors. The role of GFs in the regulation of cellular growth and differentiation in other tissues is undisputed, and we have obtained substantial evidence that GFs play a similar role in the ME during OM.6,7 GFs produce their effects on cells by interacting with specific transmembrane receptors. Although there are several classes of GF receptors, many are tyrosine kinase (TK) receptors, including those for the fibroblast GF (FGF), vascular endothelial GF (VEGF), and epidermal GF (EGF) families. GF binding leads to receptor dimerization and autophosphorylation of the intracellular TK domains. Once phosphorylated, the TK domains interact with cytoplasmic adaptor proteins to activate intracellular signaling cascades.8,9 Many of the adaptor proteins that interact directly with GF receptors bind via src homology 2 (SH2) domains, and link to additional proteins via SH3 domains.10 A wealth of signaling cascades can be activated. However, the mitogen-activated protein kinase (MAPK) pathways are perhaps the most closely associated with tissue proliferation. MAPK activation stimulates gene expression via transcription factors, including AP-1, Elk1, ATF-2, and CREB, that are often involved in the proliferative responses.11
Other transmembrane receptors are also likely to be involved in mucosal responses during OM. Several mammalian cell surface receptors directly recognize pathogens. These pathogen-associated receptor proteins (PARPs) include the Toll-like receptors (TLRs) and transforming GF-β receptors,12 which recognize a variety of pathogen-associated molecular patterns (PAMPs). The best-studied PARPs are the TLRs. Recognized by their homology to the Drosophila Toll receptor, the 10 human (and 13 mouse) TLRs have been shown to be important mediators of responses to pathogenic organisms.14 Because each receptor senses a distinct repertoire of conserved microbial molecules, collectively they can detect most if not all microbes.15 Several elegant studies using epithelial cell lines derived from the human ME mucosa have linked TLR signaling to the production of mucin and cytokines in these cells.16–19 Of particular importance for OM are the TLRs that respond to bacterial products. These include TLR4, which responds to the lipopolysaccharide (LPS) of gramnegative bacteria.20 TLR1 and TLR2 respond to bacterial lipoproteins, lipopeptides, and other molecules, whereas TLR9 responds to CpG-containing DNA, from both gram-negative and gram-positive bacteria.5 Given their distributions in other tissues21,22 and ME epithelial cell lines,16–19 TLRs should be present on a variety of cell types in the ME. Downstream signaling from these TLRs occurs via a family of adaptor molecules, especially MyD88 and MAL, which via a series of intermediates can activate the MAPKs as well as NF-κB. These events influence the expression of many genes involved in inflammation, tissue proliferation, apoptosis, and other processes.5,15,23 MyD88-independent pathway for TLR4, involving TRAM and TRIF, is activated later and can activate NF-κB and produce interferon-β (IFN-β), leading to gene activation.5,15,23 Thus there are multiple means by which TLRs might influence mucosal proliferation. MyD88 is also used as an adaptor for interleukin-1 (IL-1) signaling, activating many of the same downstream signaling cascades.23 Other receptors that can mediate host cell responses to the bacterium nontypeable Haemophilus influenzae (NTHi) include the PAF receptor,24 ganglioside receptors,25 and β-glucan receptor.26 Bacteria may also signal via direct mechanical action by the motile type IV pilus,27 a pilus form recently demonstrated on NTHi.28
Although many groups have evaluated the proliferative response of ME tissue in cholesteatoma, fewer groups have studied the ME mucosa itself. However, several laboratories have evaluated GFs in the ME mucosa during OM. For example, Palacios et al29 surveyed mRNAs encoding epithelial GFs in the ME mucosa of rats during experimental bacterial otitis. They observed rapidly increased expression of several EGF family members, including EGF itself, human GF (HGF), and neuregulin-α, but reported a downregulation of β-cellulin. Both EGF and HGF receptors were also upregulated. Yetiser et al30 evaluated EGF levels in granulation tissue recovered from patients with OM, and found EGF levels comparable to those in skin but lower than those in cholesteatoma. These results suggest that several GFs have the potential to be involved in epithelial proliferation during OM.
Similarly, Jung et al31 assessed mRNAs encoding isoforms of VEGF during OM. Expression of several VEGF isoforms increased within 1 hour of endotoxin instillation in the rat ME, and remained elevated for several days. VEGF expression was also elevated in chronic human ME effusions. Their data suggest that VEGF may be involved in neovascularization of the ME subepithelial tissue during otitis.
In vitro model systems have been developed for the evaluation of GF effects on the ME mucosa. For example, Palacios et al32 exposed cultured explants of normal rat ME epithelium to several epithelial GFs. Significant enhancement of epithelial growth from the explants was observed with keratinocyte growth factor (KGF), β-cellulin, and EGF, whereas suppression of growth was observed with HGF and heregulin. Evidence of increased epithelial differentiation was observed with KGF, HGF, amphiregulin, and heregulin (Fig. 4–1).
Binding of GFs to their cognate receptors is linked to the cell nucleus, and then to cellular responses including proliferation and differentiation, by intracellular signaling pathways. To explore these pathways during OM, Palacios et al33 developed an in vitro model of bacterially induced mucosal proliferation. They found that ME mucosa harvested 48 hours after instillation of NTHi into the ME exhibited greatly enhanced proliferation in culture. They evaluated the signaling events involved in this response using assays for activation of signal transduction molecules, as well as specific inhibitors of different pathways. They observed phosphorylation of the Erk MAPK immediately after instillation of bacteria in the ME, and then later during the resolution phase. Inhibitors of both the small G protein Ras and the Erk MAPK blocked bacterially induced proliferation in ME cultures. The results suggest that Ras-Erk signaling plays a critical role in ME proliferation during OM, and may also mediate aspects of recovery from OM. Palacios et al34 used a similar strategy to evaluate signaling via p38 MAPK. This MAPK is activated early in OM, and as with Ras-Erk, inhibition reduced mucosal proliferation.
OM involves not only mucosal proliferation but also in many cases osteoneogenesis. Melhus and Ryan35 assayed the expression of genes involved in bone formation during bacterial otitis in the rat, using quantitative PCR. They found that after a brief period of inhibition of genes associated with osteoclast activity, a prolonged period of expression of bone formation genes occurs. The regulation of mucosal proliferation and differentiation during OM offers opportunities for novel treatments designed to return the mucosa to its resting state.
Mucus Production
Mucins are an important structural component of the mucociliary transport system of the ME mucosa, especially of the eustachian tube, and as such contribute to ME defense again infection. With the exception of the region near the eustachian tube orifice, the normal ME mucosa has few goblet cells and produces mucus at low levels. However, during OM the population of goblet cells and the production of mucus can increase dramatically. Mucus can become an important component of ME effusions, contributing to their viscosity and persistence, and thence to the negative sequelae of OM.
Mucins are large, glycosylated proteins produced by mucosal epithelial cells, and are the major component of mucus. They protect epithelial surfaces by trapping infectious particles, including bacteria and viruses, for mucociliary clearance. Thus the expression of mucin genes in infectious diseases, including OM, is an important component of the host response to microbes. Of the 18 mucins identified, there is currently evidence that 11 are expressed in the ME of patients during OM.36–40 Recently, substantial evidence has been obtained regarding how the genes encoding these mucins are regulated in the ME mucosa.
Lin et al41 performed a gene array analysis of altered gene expression in the rat ME during pneumococcal infection. They observed upregulation of the Muc2 and Muc5 genes 6 weeks after inoculation. In addition, their morphology studies demonstrated a thickened mucosa and submucosa with increased expression of glycoproteins. Jono et al38 reported that NTHi utilizes the TGF-β-Smad and TLR2-MyD88-TAK1-NIK-IKKβ/γ-IκBα pathways to upregulate Muc2 mucin gene transcription. In contrast, Wang et al39 and Jono et al42 found that Muc5Ac transcription was upregulated by a TLR2-MyD88-dependent p38 MAPK pathway and downregulated by a phosphatidylinositol (PI3)-kinase-Akt pathway. Also in contrast to Muc2, TGF-β-Smad signaling downregulates p38 by inducing MAPK phosphatase-1, thereby negatively regulating Muc5Ac. In addition to the studies performed using bacteria, Kim et al43 found that bacterial LPS upregulates MUC5AC mRNA in the rat ME mucosa, with maximal expression 1 to 3 days after inoculation.
Figure 4–1 (A) Surface area (in millimeters squared) of epithelial growth of middle ear mucosa explants treated with different concentrations of specific growth factors (in nanograms per milliliter). Compared with the negative control (untreated) mucosa, treatment with epidermal growth factor (EGF), keratinocyte growth factor (KGF), and β-cellulin resulted in significant (p < .05) growth. Vertical lines represent 1 standard error (SE). (B) Optical density of middle ear mucosa epithelium treated with different concentrations of specific growth factors (in nanograms per milliliter) and stained with anticytokeratin antibody to evaluate the degree of differentiation of the cells. Compared with the negative control mucosa, treatment with heregulin, amphiregulin, human growth factor (HGF), and KGF resulted in significant differentiation. Vertical lines represent 1 SE.
Because proinflammatory cytokines play a role in the pathogenesis of OM, Lin et al44 assessed the effect of tumor necrosis factor-α (TNF-α) on rat ME mucin gene expression and found that TNF-α increased Muc2 expression. Similarly, Smirnova et al45,46 observed that TNF-α, IL-6, and IL-8 stimulated MUC5AC and MUC5B mucin secretion.
Chung et al47 showed that MUC5AC was expressed in only a small portion of the goblet cells of ME mucosa from patients with mucoid OM. They concluded that both serous secretions and mucin might make the ME effusion more viscous and that mucins other than MUC5AC might have a major role in the viscosity of ME effusion.
As with mucosal proliferation, as our understanding of the regulation of mucin production increases, it may be possible to design pharmacologic interventions that could reduce their production and enhance recovery from OM.
Innate Immunity
The concept of innate immunity has expanded significantly in the past decade, driven by the discovery of new families of natural antibiotic substances, and transmembrane receptors that respond to a wide variety of molecules produced by pathogens, such as the TLRs. These discoveries have revolutionized our concepts regarding the first line of defense in the ME.
Lim et al48 demonstrated the importance of innate immune molecules in protecting the tubotympanum against OM pathogens. Their results show that β-defensin-1 (BD-1) and BD-2, surfactant proteins A and D, lysozyme, and lactoferrin are expressed in the ME and eustachian tube. They also found that the defensins and lysozyme have distinct and potent antimicrobial activity against NTHi, Streptococcus pneumoniae, and Moraxella catarrhalis. Moon et al49 found BD-2 is expressed in the ME mucosa of humans and rats, and that its expression is upregulated by proinflammatory stimuli such as IL-1α, TNF-α, and LPS. Moreover, they demonstrated that transcriptional activation of the BD-2 gene is mediated through an Src-dependent Ras-Raf-MEK1/2-ERK pathway.
Stenfors et al50 evaluated the coating of the bacteria with antibacterial substances, including lysozyme, lactoferrin, and immunoglobulins IgG, secretory IgA, and IgM. They also tested the microbes’ ability to penetrate epithelial cells during infectious mononucleosis (IM) caused by Epstein-Barr virus. The samples studied were collected from the oropharynx of patients with current IM. Their results demonstrated a significant reduction in bacterial coating with IgG and S-IgA, whereas a significant increase in coating was observed with lactoferrin and IgM. No significant change in coating of the bacteria by lysozyme was seen. The authors concluded that reduced bacterial coating with IgG and S-IgA immunoglobulins, combined with bacterial penetration into epithelial cells, may exacerbate the bacterial colonization of oropharyngeal mucosal membranes observed during IM. This enhanced colonization may result in a greater frequency of infections such as OM and sinusitis.
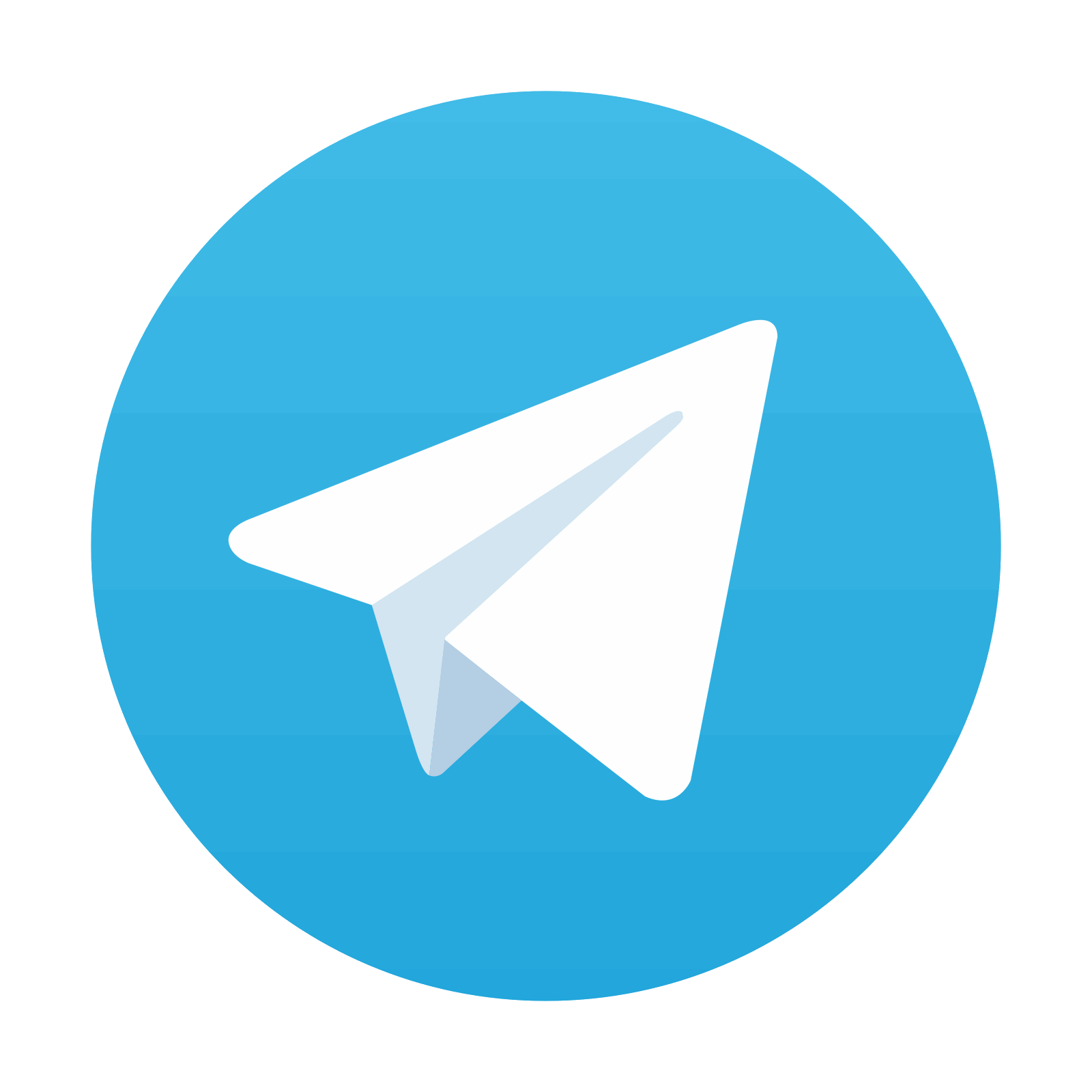
Stay updated, free articles. Join our Telegram channel

Full access? Get Clinical Tree
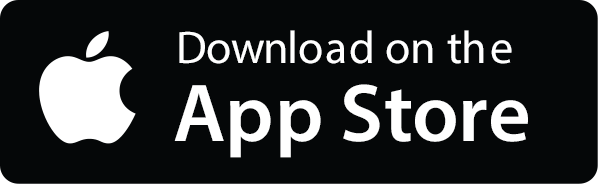
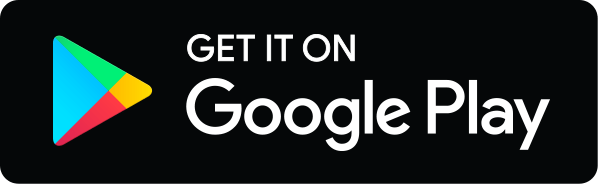