Middle Ear and Temporal Bone Trauma
Rodney C. Diaz
Sally M. Kamal
Hilary A. Brodie
Temporal bone trauma can result in significant morbidity and, rarely, mortality. The temporal bone houses or encapsulates many important structures, all of which are at risk of injury with trauma to the temporal bone; these include the facial nerve, vestibulocochlear nerve, cochlea and labyrinth, ossicular chain, tympanic membrane, external auditory canal, temporomandibular joint, lower cranial nerves, jugular vein, and carotid artery. Damage to each anatomic structure can lead to distinctive long- and short-term sequelae.
Adjacent intracranial structures such as the temporal lobe and meninges, abducens nerve, and brainstem may also be injured with temporal bone fracture. Fracture of the temporal bone can expose intracranial contents to the external world, resulting in cerebrospinal fluid (CSF) fistula, meningitis, and brain herniation.
In addition to inducing neurotologic manifestations by direct injury to these structures, temporal bone fractures can have associated intracranial complications such as epidural or subdural hematomas, intraparenchymal contusion or hemorrhage, cerebral edema, posttraumatic encephalopathy, and elevated intracranial pressure. Neurotologic symptoms can also result from shearing strain within the brain tissue with disruption of vessels, axons, dendrites, and synapses (1).
EPIDEMIOLOGY
Injury to the lateral skull has increased with the advent of modern technology; the leading cause of temporal bone fracture is involvement in motor vehicle accidents (2). In the past, 75% of motor vehicle accidents resulted in a head injury. The increased utilization of seat belts and the advent of frontal and side curtain airbags may, however, alter these statistics in the future. When the head trauma is of sufficient magnitude to fracture the skull, 14% to 22% of those injured sustain a temporal bone fracture (3, 4). In the largest series of temporal bone fractures reported to date, 31% of the temporal bone fractures in the general population resulted from motor vehicle accidents (2). Assault was the second most common cause, followed by falls and motorcycle accidents. Pedestrian injuries, bicycle accidents, gunshot wounds, all-terrain vehicle accidents, sports injuries, and miscellaneous injuries accounted for a quarter of all cases (2) (Fig. 150.1). The most common injury etiology in temporal bone fractures specific to the pediatric population is equally divided between motor vehicle accidents and falls (between 30% and 60% each) (5, 6, 7, 8, 9).
Temporal bone fractures are reported to occur across all age groups with over 70% of fractures occurring in second, third, and fourth decades of life (2). These fractures occur predominantly in males, with a 3:1 to 4:1 ratio of males to females affected (2, 10). The predisposition to temporal bone fractures in males is attributed not to an inherent structural weakness of the male skull versus the female skull but rather to biased involvement of males in many of the above at-risk activities. This is evidenced by the fact that head injuries in general also follow a 4:1 male-to-female ratio (10).
In a prospective study of 350 consecutive patients treated for head trauma, 10% were found to have temporal bone fractures on radiographic evaluation using helical CT, whereas only 6% manifested clinical signs of temporal bone fracture on primary evaluation (10). Large retrospective reviews at level I trauma centers have found an incidence of temporal bone fracture in 2% to 4% of consecutive head injury patients (11, 12).
PATHOPHYSIOLOGY
The temporal bones are pyramidal structures in the thick bone of the skull base and consequently require a great force to fracture. Early studies of static loading of the lateral
skull estimated fracture thresholds of 300 to 800 kg (15). More recent dynamic loading studies have estimated the force of lateral impact required to fracture the temporal bones of fresh cadavers at 6,000 to 8,000 N, or approximately 1,300 to 1,800 lb (16, 17). Comparison of data from static versus dynamic loading experiments indicates an increase in force tolerance by a factor of two under dynamic loading (18). Such fractures typically take the path of least resistance, which is along structurally weakened points such as the various foramina perforating the skull base.
skull estimated fracture thresholds of 300 to 800 kg (15). More recent dynamic loading studies have estimated the force of lateral impact required to fracture the temporal bones of fresh cadavers at 6,000 to 8,000 N, or approximately 1,300 to 1,800 lb (16, 17). Comparison of data from static versus dynamic loading experiments indicates an increase in force tolerance by a factor of two under dynamic loading (18). Such fractures typically take the path of least resistance, which is along structurally weakened points such as the various foramina perforating the skull base.
Sixty percent of temporal bone fractures are categorized as “open” fractures presenting with bloody otorrhea, brain herniation, or CSF draining from the ear canal, eustachian tube, or penetrating wound site (2). These patients are at greater risk for meningitis than those without evidence of an intracranial connection. In addition, those patients with fractures traversing the otic capsule are at even still greater risk of meningitis, sometimes delayed for years or decades, due to an inability of the otic capsule enchondral bone to remodel and heal (19, 20, 21). Pollak reported a 51-year-old man who died of meningitis who had suffered an otic capsule-disrupting fracture in childhood (20). The histopathology of his temporal bone revealed pus in the middle ear extending through an unhealed fracture line across the otic capsule. The fracture line contained a loose fibrous tissue. Membranous bone, such as that along the tegmen, has the capability to form callus and heal, whereas the enchondral bone of the otic capsule does not. Fractures through the otic capsule will generally only partially fill with fibrous tissue, although the surface may potentially seal with periosteal bone reaction (20) (Fig. 150.2).
Trauma to the temporal bone often results in one or more neurotologic complications, depending on the severity of injury and type of fracture, and can vary between adult and pediatric populations. Table 150.1 summarizes incidence of common complications of temporal bone fractures in the general and pediatric populations (2, 6).
CLASSIFICATION
Temporal bone fractures have traditionally been divided into transverse and longitudinal fractures, based on the relationship of the fracture line to the axis of the petrous ridge (11, 22, 23). Some authors argue that the majority of fractures are actually oblique as opposed to longitudinal and/or are quite frequently mixed (24, 25). This anatomical classification scheme is being replaced with a new structural scheme classifying fractures by whether they disrupt or spare the otic capsule, the bone housing the cochlea and semicircular canals (2, 26, 27) (Figs. 150.3, 150.4, 150.5).
Fractures that spare the otic capsule typically involve the squamosal portion of the temporal bone and the posterosuperior wall of the external auditory canal. The fracture passes through the mastoid air cells and middle ear and fractures the tegmen mastoideum and tegmen tympani. The fracture proceeds anterolateral to the otic capsule, typically fracturing the tegmen in the region of the facial hiatus. Otic capsule-sparing fractures typically result from a blow to the temporoparietal region.
TABLE 150.1 INCIDENCE OF COMMON COMPLICATIONS OF TEMPORAL BONE FRACTURES IN THE GENERAL AND PEDIATRIC POPULATIONS | ||||||||||||||||||||||||||||||||
---|---|---|---|---|---|---|---|---|---|---|---|---|---|---|---|---|---|---|---|---|---|---|---|---|---|---|---|---|---|---|---|---|
|
Otic capsule-disrupting fractures pass through the otic capsule generally proceeding from the foramen magnum across the petrous pyramid and otic capsule. The fracture will often pass through the jugular foramen, internal auditory canal, and foramen lacerum. These fractures do not typically affect the ossicular chain or the external auditory canal (28). Otic capsule-disrupting fractures generally result from blows to the occipital region.
Longitudinal fractures are reported to constitute 70% to 90% of temporal bone fractures with the remaining 10% to 30% categorized as transverse (11, 24, 26, 28, 29, 30, 31). In two large series using the newer classification scheme, only 2.5% to 5.8% of fractures disrupted the otic capsule (2, 25). This would suggest that many fractures that are oriented perpendicular to the petrous ridge do not actually cross the otic capsule. Many of the otic capsule-disrupting fractures are actually oriented in the longitudinal plane (25).
![]() Figure 150.3 Axial bone window CT demonstrates a longitudinally oriented fracture (arrows) sparing the otic capsule. |
![]() Figure 150.4 Axial bone window CT demonstrates a transverseoriented fracture, secondary to a motor vehicle accident, disrupting the otic capsule (arrows). |
The traditional schema of anatomical designation of fracture type was first extensively used in biomechanical studies of cadaveric skull deformation, without correlation to functional outcome (23). In contrast, the newer structural schema underscores the importance of otic capsule involvement in heralding neurotologic sequelae. Otic capsule-disrupting fractures have a much higher incidence of facial nerve paralysis than otic capsule-sparing fractures (30% to 66% vs. 6% to 13%) (2, 25, 32). In addition, Fisch reported a much higher incidence of nerve disruption in fractures involving the otic capsule (33). There is a two- to tenfold increase in CSF fistula in otic capsule-disrupting fractures as well as a much greater risk of intracranial injuries, compared to otic capsule-sparing fractures (2, 25, 27). Fractures
that disrupt the otic capsule will almost always result in a sensorineural hearing loss, although there are reported exceptions to the rule (34). Hearing loss in otic capsule-sparing fractures tends to be conductive or mixed (2, 27). Historically, sensorineural hearing loss has been associated with transverse rather than longitudinal fracture type, but in a recent study, this correlation was not borne out but rather inverted: longitudinal fractures were three times as likely to be associated with sensorineural hearing loss as were transverse fractures (27). This study demonstrated a statistically significant difference in complication rates involving facial nerve injury, CSF fistula, and conductive hearing loss when 155 temporal bone fractures were categorized with the structural schema of otic capsule-sparing versus otic capsule -disrupting fracture types, while concurrently demonstrating no statistical significance when using the traditional classification schema of longitudinal versus transverse fracture types. A recent review of 30 temporal bones supported these findings and noted that otic capsule-disrupting fractures were five times more likely to have facial nerve injury, 25 times more likely to have sensorineural hearing loss, and 8 times more likely to have CSF leak (32).
that disrupt the otic capsule will almost always result in a sensorineural hearing loss, although there are reported exceptions to the rule (34). Hearing loss in otic capsule-sparing fractures tends to be conductive or mixed (2, 27). Historically, sensorineural hearing loss has been associated with transverse rather than longitudinal fracture type, but in a recent study, this correlation was not borne out but rather inverted: longitudinal fractures were three times as likely to be associated with sensorineural hearing loss as were transverse fractures (27). This study demonstrated a statistically significant difference in complication rates involving facial nerve injury, CSF fistula, and conductive hearing loss when 155 temporal bone fractures were categorized with the structural schema of otic capsule-sparing versus otic capsule -disrupting fracture types, while concurrently demonstrating no statistical significance when using the traditional classification schema of longitudinal versus transverse fracture types. A recent review of 30 temporal bones supported these findings and noted that otic capsule-disrupting fractures were five times more likely to have facial nerve injury, 25 times more likely to have sensorineural hearing loss, and 8 times more likely to have CSF leak (32).
![]() Figure 150.5 Axial bone window CT demonstrates a mixed fracture (arrows) that spares the otic capsule. |
The newer classification scheme emphasizes functional outcome and better prognosticates those temporal bone fractures that will exhibit neurotologic manifestations. In addition to the predictive value for various complications and comorbidities, categorization of fractures into otic capsule-sparing and otic capsule-disrupting injuries guides the indications for surgical intervention for CSF fistula and facial paralysis as well as the surgical approach to be utilized in their repair.
EVALUATION
It is uncommon for temporal bone fractures to occur in isolation of other bodily injury, and consequently the initial evaluation and management is focused on the urgent life-threatening issues of securing an airway, controlling hemorrhage, evaluating the neurologic status, and stabilizing and evaluating the cervical spine. Following or concurrent with this evaluation, the neurotologic exam is performed. It is extremely important to assess facial nerve function in the emergency room as early as possible, prior to the administration of muscle relaxants, as will be discussed below. The ear exam focuses on the condition of the auricle, ear canal, tympanic membrane, and middle ear.
Clinical Evaluation
The auricles are inspected for lacerations and hematomas. Lacerations are closed after thorough cleaning and debridement of exposed cartilage. Hematomas are drained and pressure bolsters are sutured in place to close the dead space and prevent a recollection of blood. Untreated, the auricular hematomas will result in an auricular chondropathy or “cauliflower ear.”
Battle sign is seen in the presence of basilar skull fractures, including temporal bone fractures. Extravasation of blood from the mastoid emissary veins leads to ecchymosis over the mastoid bone and mastoid tip. This sign may be present in the initial clinical assessment but more often appears in a delayed fashion days after the injury.
The ear canal is inspected for fractures along the roof, CSF otorrhea, degree of hemorrhage, and the presence of brain herniation. The ear is examined as aseptically as possible. Blood and cerumen in the ear canal should never be debrided with irrigation. Following stabilization in the emergency room and transfer to the ward or intensive care unit, the ear can be more carefully examined with the aid of an operating microscope. Typical findings include fractures along the scutum and roof of the external auditory canal and/or tympanic membrane perforations. Hemotympanum or bloody otorrhea are almost invariably present and are two of the most common signs of temporal bone fracture (Figs. 150.6, 150.7, 150.8).
The integrity of the tympanic membrane is assessed, as is the presence of hemotympanum. Hemotympanum and any associated serous effusion generally resolve spontaneously, with resolution of concomitant conductive hearing loss, within 4 to 6 weeks and simply require observation. Traumatic tympanic membrane perforations generally heal spontaneously as well, and consequently no acute intervention is necessary.
Hearing is initially assessed clinically at the bedside with a progressively louder whispered voice. Tuning fork examination is useful in delineating conductive from sensorineural hearing loss. Pure tone and speech audiometry are not typically necessary in the acute setting and are obtained after the patient is stabilized. However, in the presence of complications of facial paralysis or CSF fistula in which surgical intervention may be necessary,
preoperative audiometry is necessary as results will dictate the options available for management within the treatment algorithm, as is discussed below.
preoperative audiometry is necessary as results will dictate the options available for management within the treatment algorithm, as is discussed below.
![]() Figure 150.6 Otoscopic image demonstrating a nondisplaced fracture along the scutum (black arrow). Blood is layering out inferiorly (white arrow). |
The neurotologic exam should note the presence or absence of nystagmus as well as the type of nystagmus. Peripheral vertigo generally manifests with horizontal or rotatory nystagmus and is suppressible with visual fixation. Central vertigo may present with vertical or direction- changing nystagmus that fails to suppress and may even enhance with fixation. The most common type of vertigo following head trauma is benign paroxysmal positional vertigo (BPPV) (35). BPPV classically manifests with latent-onset, transient rotatory nystagmus and concomitant vertigo with the Dix-Hallpike maneuver (35, 36). The nystagmus occurs with the affected ear down. There is a 2- to 10-second latency followed by 10 to 30 seconds of rotatory nystagmus in the geotropic direction—that is, with the upper half of the globe rotating in fast phase direction towards the ground. Upon returning to an upright sitting position, a second bout of vertigo with nystagmus in the counterrotatory direction is often seen. The nystagmus is fixed in direction and is fatigable with repeated maneuvers. In contrast, central positional vertigo induces directionchanging nystagmus, which has no latency and is nonfatigable. Interestingly, the incidence of vertigo does not closely correlate with the severity of the trauma (37).
Electronystagmography (ENG) is helpful in categorizing and localizing the vestibular injury; however, as with audiometry, it is typically not obtained in the acute setting. The vast majority of posttraumatic vertigo resolves spontaneously. If the symptoms persist following discharge from the hospital, an ENG can be obtained to help clarify diagnosis on an outpatient basis.
Pneumatic otoscopy, which consists of applying positive and negative pressure in the ear canal with a pneumatic otoscope, should not be performed in the acute setting. Application of positive and negative pressure in the potential setting of CSF fistula, and/or communication with the labyrinth if the fracture is otic capsule disrupting, increases the risk of iatrogenic injury from introduction of infection or air into the intracranial space or inner ear. This risk outweighs any potential diagnostic benefit in the acute setting. If the patient continues to experience vertigo or is experiencing fluctuating or progressive hearing loss longer than 1 week after the injury, a perilymph fistula is suspected and pneumatic otoscopy can then be performed to check for nystagmus and vertigo. The continued presence of spontaneous nystagmus following traumatic injury to the temporal bone is also suggestive of a perilymph fistula. Alternobaric testing with pneumatic otoscopy should not be performed if there is evidence of a persistent CSF fistula or infection in the middle ear.
Radiographic Evaluation
Patients with severe head trauma of the magnitude required for a temporal bone fracture will generally already have had a computed tomography (CT) scan of the head to assess for intracranial hemorrhage and other intracranial injuries. Temporal bone fractures can typically be discovered on standard head CT alone and can be traditionally classified as longitudinal, transverse, or mixed, or categorized with the more clinically significant otic capsule-sparing versus otic capsule-disrupting classification, as discussed above.
Intravenous contrast-enhanced CT is not necessary for the diagnosis of temporal bone fractures. Additional imaging of the temporal bone with axial and coronal high-resolution CT scanning (HRCT) is indicated in the presence of facial paralysis, signs of CSF otorrhea or
rhinorrhea, disruption of the superior wall of the external auditory canal or scutum with potential trapping of epithelium (Fig. 150.9), or suspected vascular injury. HRCT of the temporal bones is also indicated if surgical intervention is required in the management of a neurotologic complication.
rhinorrhea, disruption of the superior wall of the external auditory canal or scutum with potential trapping of epithelium (Fig. 150.9), or suspected vascular injury. HRCT of the temporal bones is also indicated if surgical intervention is required in the management of a neurotologic complication.
![]() Figure 150.9 Otoscopic photograph demonstrating a distracted fracture along the roof of the external auditory canal and scutum allowing for potential in growth of canal skin. |
HRCT proves an invaluable tool for management decision making in the setting of immediate-onset, complete facial paralysis: A finding of bony impingement of the facial nerve and fallopian canal, either by bony spicules or by translocation of fracture components, is an indication for facial nerve exploration and decompression. In contrast, delayed-onset or incomplete facial paralysis alone does not require further radiologic evaluation with HRCT.
Hearing loss alone, whether conductive or sensorineural, in the absence of other complications also does not warrant additional temporal bone imaging in the acute setting. Demonstration of a transverse fracture or otic capsule-disrupting fracture in the setting of anacusis or profound sensorineural hearing loss will not alter the treatment plan. However, preoperative assessment with CT scanning in patients with a conductive hearing loss of sufficient magnitude to warrant exploration and ossicular reconstruction may provide useful information that may influence the surgical approach.
Ossicular discontinuity following temporal bone trauma can often be imaged on HRCT; types of discontinuities most easily identifiable on HRCT are incus dislocation and malleoincudal subluxation (38). In complete incus dislocation, in which both the malleoincudal and incudostapedial joints have been disrupted, the resulting position of the incus can be quite variable: residing in the epitympanum lateral to the malleus head (causing a “Y” configuration when viewed on coronal cuts), within the external auditory canal, or even not visible at all (presumably extruded from the body or “lost” within the mastoid air cells). Partial dislocation of the incus and subluxation of the malleoincudal joint creates a diastasis of the malleus head “ice cream scoop” from the incus body “cone,” with or without rotation of the incus body, on axial imaging. Incudostapedial subluxation and stapes fracture are difficult to ascertain on CT.
Posttraumatic sensorineural hearing loss is highly correlated with otic capsule-disrupting fractures, but many cases present with no radiologic findings. In these cases, an impulsive disruption of the membranous labyrinth, termed cochlear concussion, is theorized (39). Intralabyrinthine hemorrhage is an etiology that can be imaged and is seen as a region of signal hyperintensity within the vestibule and cochlea on noncontrast T1-weighted MR imaging if performed acutely (40, 41).
Similarly, identification of perilymph fistula is usually not directly possible on radiographic imaging, but this diagnosis can be suggested when recognition is made of otic capsule-disrupting fracture, stapes fracture, loss of stapes bone, or pneumolabyrinth in the setting of persistent fluctuating hearing loss and vertigo (38, 42).
The role of HRCT in the evaluation of potential carotid artery injuries is unclear. Resnick et al. reported a 5% incidence of carotid injury in basilar skull fractures if the fracture spares the carotid canal and an 18% incidence of carotid injury in patients with fractures through the carotid canal seen on HRCT (43). However, Kahn argues that, in an asymptomatic patient, HRCT demonstration of a fracture through the carotid canal yields no valuable additional information (44). Subsequent angiography performed in cases of asymptomatic carotid canal fractures yielded no evidence of carotid artery injury and provided no clinical utility (44). Consequently, in completely asymptomatic patients sustaining a temporal bone fracture, who are neurologically intact, HRCT and angiography are not required. On the other hand, if there are any transient or persistent neurologic deficits in patients with basilar skull fractures, HRCT of the temporal bone together with CT angiography is indicated (Fig. 150.10). MR angiography can also be considered for screening evaluation of the petrous carotid artery.
Sun et al. (45) sought to identify predictive findings of carotid artery injury on CT scan in patients who underwent angiography. Sphenoid bone fracture, petrous carotid canal fracture, and pneumocephalus were evaluated. With all three findings on CT scan, specificity of 85% and negative predictive value of 80% were obtained, while sensitivity was low. Further evaluation of correlation between CT findings and carotid artery injury is warranted.
Conventional skull radiography, including Schüller, Towne, Chamberlain, Stenvers, and basal views as well as tomograms, has been supplanted by HRCT scanning and no longer plays a role in the evaluation of patients suspected of having a temporal bone fracture.
In evaluating temporal bones for fracture on CT, care must be taken to avoid mistaking normal anatomic structures for fracture lines. Extrinsic suture lines (petro-occipital, temporo-occipital, occipitomastoid sutures), intrinsic suture lines (tympanomastoid, tympanosquamous, petrotympanic fissures), and intrinsic channels (cochlear aqueduct, vestibular aqueduct, glossopharyngeal nerve/glossopharyngeal sulcus, subarcuate artery/petromastoid canal, singular nerve/singular canal, Arnold nerve/mastoid canaliculus, Jacobson nerve/inferior tympanic canaliculus, and greater superficial petrosal nerve/facial hiatus) may all mimic fracture lines within the temporal bone (38). Knowledge of their anatomic relationships and distinction with true fracture lines is necessary to prevent incorrect interpretation of CT imaging.
MANAGEMENT
The morbidity and mortality associated with temporal bone fractures result from injuries to the structures passing through the temporal bone or abutting the temporal bone as described above. The most common complications include facial nerve injury, CSF fistula, sensorineural hearing loss, conductive hearing loss, cholesteatoma formation, and stenosis of the ear canal. In addition, rare complications may occur, including abducens nerve injury, trigeminal nerve injury, Horner syndrome, carotid injury, sigmoid sinus thrombosis, traumatic porencephalic cyst formation, and intracranial dislocation of the mandibular condyle (12, 46, 47, 48, 49, 50, 51).
Facial Nerve Injury
Facial paralysis is a severely disfiguring complication of temporal bone fractures. Six to seven percent of temporal bone fractures result in facial paralysis. This figure represents data based on large prospective and retrospective series of all consecutive patients treated for head injury or temporal bone fracture, thereby avoiding previous systematic bias towards overreporting of clinical complications (2, 10). Of these facial nerve injuries, one-fourth are complete. The incidence of facial nerve injury in temporal bone fractures of the pediatric population is 3% to 9%, comparable to that of the adult population (5, 8, 9).
The incidence of facial paralysis in the literature has previously been reported as high as 30%. However, this estimation is exaggerated due to sampling error: Simple, uncomplicated temporal bone fractures without facial nerve injury are often not referred for otolaryngologic consultation. The incidence of facial paralysis in the literature has also been overestimated by including patients in retrospective reviews who have been referred to a tertiary care center for management of complications of temporal bone fractures such as facial paresis. Since the entire pool of temporal bone fracture patients had not been included in prior statistics, the reported incidence of complications has previously been quite biased.
If head trauma patients are carefully evaluated in the emergency room upon admission, prior to the administration of muscle relaxants, 27% of facial nerve injuries will present with immediate-onset facial paralysis; 73% will have facial motion in the initial examination and subsequently deteriorate (2). The latency in onset of facial palsy ranges from 1 to 16 days. It is crucial to differentiate “delayed onset” from “delayed diagnosis.” Delayed onset of facial paralysis is defined as documented facial function in the emergency room that subsequently deteriorates. A delayed diagnosis of facial paralysis occurs when the patient is given a paralytic agent and intubated prior to examination of facial function. In this situation, an assessment of facial function is delayed until extubation. These patients should be categorized as unestablished onset and treated similar to the immediate-onset patients. In one large series, 10% of patients fell into this unestablishedonset category (52).
Many aspects in the management of facial nerve injury remain controversial. One of the main issues to be resolved is the indication for surgical exploration. Since the vast majority of traumatic facial palsies resolve spontaneously, the decision of which injuries to explore is based on prognostic factors for poor outcome. The factors that are assessed in predicting recovery of facial function include timing of onset (delayed vs. immediate onset), severity of the injury (penetrating vs. nonpenetrating), and the presence of associated infection.
The delay of onset of paralysis following temporal bone fracture is the most important of the predictive factors. In one series of 37 delayed-onset facial palsies, five were lost to follow-up and the remainder recovered to a House- Brackmann grade I or II (2). McKennan and Chole (53) described their experience with 17 patients with immediate- onset facial paralysis and 19 patients with delayedonset paralysis. Complete spontaneous recovery of facial function occurred in 94% of the patients with delayedonset paralysis. The one remaining patient had a House- Brackmann grade II recovery. In contrast, 8 out of the 17 immediate-onset paralysis patients had facial nerve transections.
Turner reviewed a large series of traumatic facial paralysis treated conservatively (54). His paper included 36 immediate-onset and 34 delayed-onset facial palsies. Complete recovery occurred in 94% of the delayed-onset cases and 75% of immediate-onset palsies. The one patient with delayed-onset paralysis who had no recovery of function developed the facial palsy coincident with acute otitis media. Similarly, in a recent systematic review, the outcomes of 25 patients with immediate paralysis and 20 patients with delayed paralysis treated with conservative management were assessed. Eighty percent of the delayed paralysis patients had complete recovery of facial function while less than 40% of those with immediate paralysis recovered complete facial function (55). Maiman et al. (56), in contrast, found no correlation between immediate- or delayed-onset facial paralysis, treated nonsurgically, and outcome. However, 44 out of 45 of their patients (including both immediate and delayed onset) had satisfactory recovery. Review of the above literature argues strongly against surgical exploration and decompression of delayed posttraumatic and facial paralysis. The natural course of delayed facial paralysis is almost always recovery of facial function to a House-Brackmann grade I or II. There are no convincing data in the literature that demonstrate that surgical intervention in delayed-onset paralysis will increase the probability of complete recovery of function.
May describes the difficulty in differentiating immediate-from delayed-onset facial paralysis. He explored reportedly “delayed-onset” palsies and found on occasion a severed nerve (57). This observation highlights the necessity of careful examination in the emergency room prior to chemical paralysis. Even in comatose patients, painful stimuli will generally induce a symmetric grimace when both facial nerves are intact; unilateral weakness of all facial branches implies a peripheral—that is, facial nerve— lesion, whereas selective weakness of the lower branches with bilateral temporal branch sparing implies a central lesion, proximal to the facial nucleus. Admittedly, information regarding facial nerve function immediately following an injury is not always available. Sometimes the exam is omitted due to attention to other life-threatening complications or because the patient has already received muscle relaxants with intubation. Some patients are not responsive to pain and a grimace cannot be induced. The critical issue is whether or not any facial function has been identified. If facial function is present in the emergency room and subsequently deteriorates, our experience is that the patient will recover without surgical treatment. When reliable information from the emergency room is unavailable, the patient is never documented to have facial function, and the diagnosis of facial palsy is delayed by a few days, these cases must be categorized as “unknown onset” and considered with the immediate-onset group when considering management options.
The degree of facial nerve injury is also a critical factor in guiding the management algorithm. Incomplete paresis rarely fails to completely resolve spontaneously unless an additional insult to the nerve, such as infection, occurs (2). A review of 71 patients with partial facial weakness demonstrated that the overwhelming majority of patients achieved recovery to House-Brackmann grade I and no patients had House-Brackmann grade VI outcome (55). Consequently, only patients with complete paralysis of immediate or unknown onset are considered for surgical exploration.
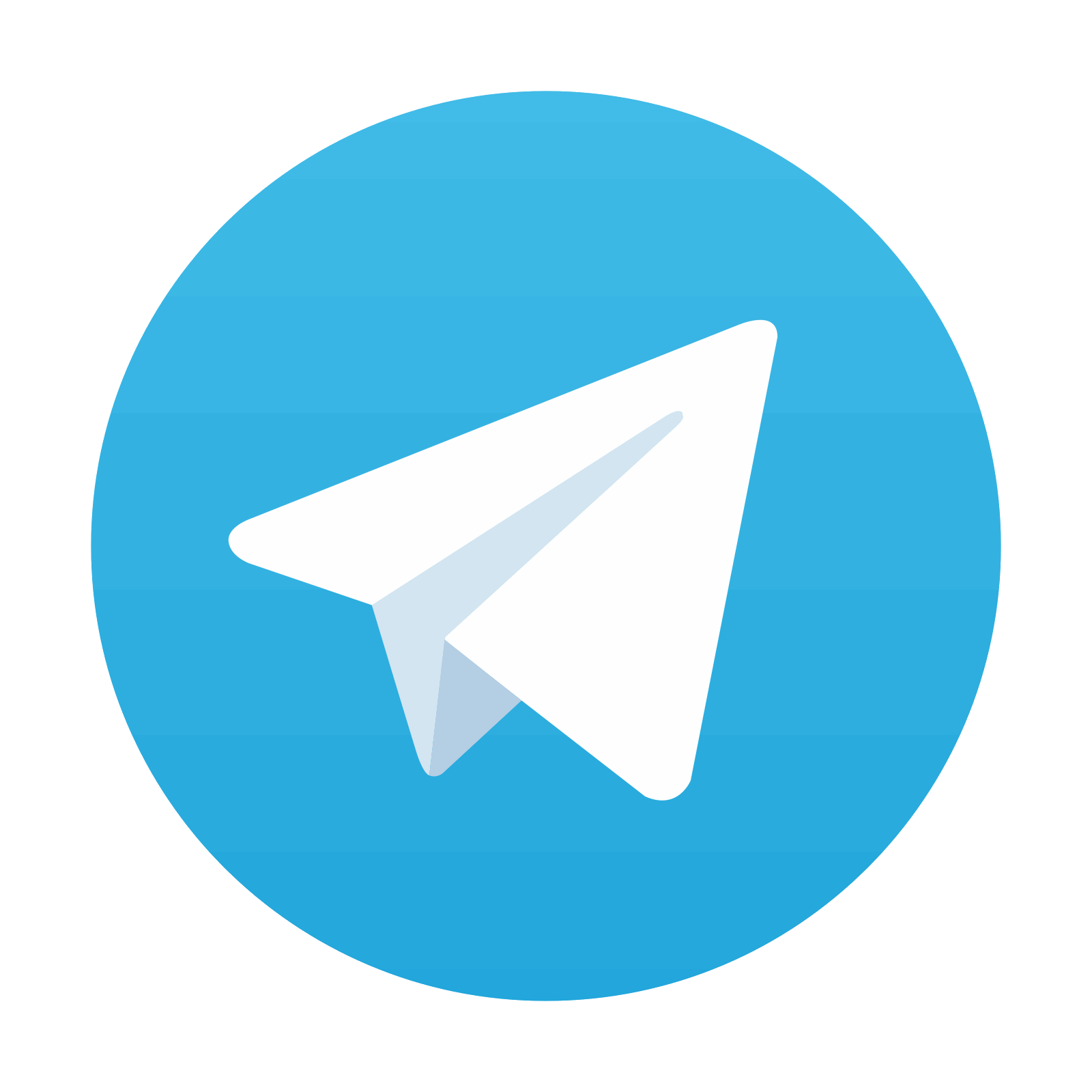
Stay updated, free articles. Join our Telegram channel

Full access? Get Clinical Tree
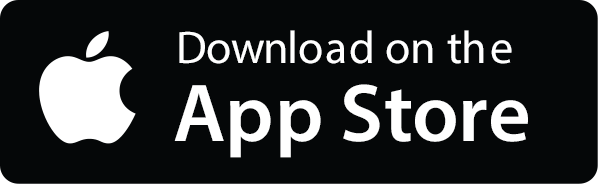
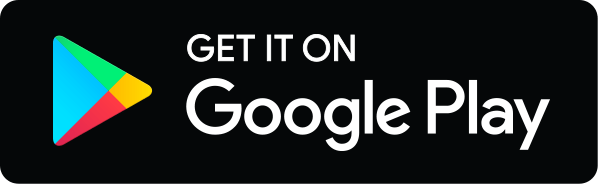