Mujtaba A. Qazi, MD; Abu-Bakar Zafar, MD; and Jay S. Pepose, MD, PhD
WHY THE PREMIUM INTRAOCULAR LENS SURGEON SHOULD TRANSITION TO MICROINCISIONAL CATARACT SURGERY
Patient expectations have changed dramatically in recent years. In the past, cataract patients were delighted with the increased contrast sensitivity and reduction of forward light scatter associated with cataract extraction and intraocular lens (IOL) implantation and were satisfied with a change in spectacle correction a month later. Today, many cataract surgery patients anticipate better vision postoperatively than they may have even enjoyed prior to cataract formation, regardless of IOL selection. In effect, irrespective of the choice of a conventional or a premium IOL, many patients expect to experience outcomes consistent with refractive cataract surgery. As a rule, this benchmark is even higher in those patients who have paid the additional expense of premium lifestyle-enhancing, presbyopia-correcting IOLs.
With the convergence of refractive corneal and lens surgeries, satisfying these progressively higher demands requires meticulous and uncomplicated cataract surgery, control and adjustment of corneal astigmatism, and an uneventful postoperative course characterized by rapid visual rehabilitation without potentially devastating complications such as endophthalmitis. In consideration of these stated goals, Table 11-1 lists a number of potential advantages of cataract surgery using an incision of 2 mm or less, which has been termed microincisional cataract surgery (MICS).1 This chapter details the following: the evolution of microincisional surgery; highlights the various steps, instruments, and phaco settings involved; and presents a step-by-step approach to smoothly transitioning to MICS.
Table 11-1
(Potential) Advantages of Microincisional Cataract Surgery
▲ Watertight wound closure
▲ Reduced need for suture placement
▲ Reduced risk of endophthalmitis
▲ Reduced surgically induced astigmatism
▲ Reduced iris prolapse
▲ Improved anterior chamber stability
▲ Less fluid egress and volume of balanced salt solution utilized (decreased vitreous hydration)
▲ Increased phaco efficiency requiring lower ultrasound power and shorter time
BACKGROUND
Modern cataract surgery has transitioned from en bloc extraction techniques, requiring incision sizes greater than 10 mm, to techniques incorporating phacoemulsification (phaco) combined with foldable and injectable IOL insertion through incision sizes less than one-fifth those initially required by Sir Harold Ridley and his contemporaries. With further advancements in phacoemulsification and aspiration equipment, ocular viscoelastic device (OVD), fluidics, and IOL designs, strategies to further reduce incision size have become feasible. An important early step in development was in 1985, when Shearing2 described a method of bimanual phacoemulsification as a means to further diminish the incision size. By the turn of the 21st century, the concept of MICS, through a sub–2-mm incision, had been introduced,1 with continued refinement ever since. This transition may become mandatory if we are to eventually progress toward the ultimate goal of accommodating, capsule-filling polymers.
Among the key motivators for these advances has been the elevation of cataract surgery to a full-fledged refractive procedure, along with the advent of foldable IOLs capable of insertion through a sub–2-mm incision. MICS facilitates the goals of the modern refractive lens surgeon, which include minimal and predictable changes in corneal astigmatism, rapid healing and visual recovery, and smaller, stable watertight wound architecture to minimize wound leaks and lower risks of postoperative endophthalmitis. Nowadays, cataract surgery is routinely coupled with limbal relaxing incisions and toric and presbyopic IOL implantation. Reduction of thermal wound injury and corneal edema, control of surgical induction of corneal astigmatism (SIA), and consistency of capsulorrhexis size are key elements of modern-day cataract surgery. Improvement in immediate and long-term postoperative visual acuity and reproducibility of results has gained paramount importance.
A 2008 market survey3 of 915 surgeons from 5 European countries found that 34% were performing MICS, with the penetrance anticipated to grow to 50%. More of these surgeons utilized coaxial MICS (C-MICS, 27%) than bimanual MICS (B-MICS, 7%), as the development of coaxial MICS systems permitted more natural transition from small incision coaxial surgery to C-MICS with a few simple modifications in surgical techniques. While advances in surgical instrumentation and techniques suggest an easy transition into sub–2-mm incision cataract surgery, there are some basic steps to streamline a successful and complication-free conversion, including understanding of the required fluidics, revision of surgical instruments, adjustment of intraoperative maneuvers, management of corneal astigmatism, and further modification of IOL materials and designs. A stepwise learning process is recommended and outlined in the following section in order to facilitate a smooth transition.
FLUIDICS OF MICROINCISIONAL CATARACT SURGERY
Fluid management is a key component of MICS. Higher infusion and vacuum pressure are required for fluid injection and nuclear fragment aspiration through a smaller bore cannula, just as higher suction power is required to sip liquid through a thin vs a thicker straw. Similarly, the amount of volume aspirated at a fixed flow or suction rate through a thin straw is lower than through a large-diameter straw at the same rate. These principles are outlined in Poiseuille’s law, which relates the flow through a tube to a function of the radius cubed (r3) of the tube. A result of this relationship, along with the minimal wound leakage in MICS, is that phacoemulsification is completed using a far lower volume of balanced salt solution (BSS), resulting in less vitreous hydration and corneal edema.
In this regard, MICS phacoemulsification platforms have incorporated gas compressors to increase the infusion pressure and flow. Gas is delivered to the infusion bottle in order to maintain a continuous, high inflow of fluid via higher resistance tubing. This allows for formed and consistent anterior chamber dimensions throughout the procedure. Gas-forced infusion also serves to cool the phaco tip.
Higher vacuum may increase the risk of post-occlusion surge, where flow rates can reach 300 cc/min (6 times normal) when occlusion is broken at high (400 mm Hg) vacuum levels. Therefore, MICS fluidics often involves use of flow restrictors (Figure 11-1) to limit the impact of complete occlusion. Cruise Control, in the Sonic WAVE phacoemulsification platform (STAAR Surgical), places a 2-cm long flow restrictor (see Figure 11-1A) behind a mesh filter that traps the nuclear emulsate. This prevents the narrow 0.3-mm lumen from becoming clogged. Hence, regular aspiration flow occurs below 50 cc/min, but unwanted flow surge above 60 cc/min is limited (see Figure 11-1B), thereby minimizing the inherent risk of capsular capture and tear. Alcon’s newest peristaltic system (Centurion) utilizes active fluidics to allow a more consistent physiological intraocular pressure. The system automatically adjusts compression of the fluid bag and pump flow rate to stabilize the anterior chamber.
Some MICS fluidics designs utilize a Venturi pump in place of or in addition to peristaltic pumps. The Venturi pump (Stellaris and Millennium) customizes chamber fluidics using either a flow-based or vacuum-based system. It requires smaller diameter, flow-resistant tubing to generate vacuum as high as 600 mm Hg and steady, low flow (Figure 11-2, top). When vacuum pressure builds during occlusion, resistance to outflow increases such that the anterior chamber remains stable (Figure 11-2, bottom), thereby limiting the possibility of capsular incarceration in the phaco tip. The Stellaris also incorporates a mesh filter.
Figure 11-1. (A) Site flow restrictor reduces risk of (B) post-occlusion surge. (Reprinted with permission from STAAR Surgical.)
Thus, higher fluid inflow and vacuum pressures, along with the use of pulsed rather than continuous ultrasound power, promote influx of fragments to the phaco tip, improving followability. Often, the high flow required for MICS fluidics can help break up a fragment engaged at the phaco tip without the use of ultrasound power. The lower power utilization lessens the impact on the corneal endothelium and can result in clearer corneas on the first postoperative day.4,5
INCISION
Decreasing the incision size has been one of the fundamental advances in modern surgery, including outside the domain of intraocular surgery. Laparoscopic and mini-incisional techniques have been developed for abdominal, cardiothoracic, and gynecological procedures with the aim of limiting tissue trauma and promoting faster healing. For ocular surgeries (see Table 11-1), these principles are extended to make small incisions self-sealing as well. Watertight wound construction is paramount for proper multifocal, Crystalens (Bausch & Lomb), and toric IOL positioning; avoidance of anterior Crystalens IOL vaulting with a resultant myopic shift; and prevention of toric IOL rotation. Watertight incisions may play a significant role in reducing endophthalmitis due to late influx of surface flora.4 Additionally, smaller incision size prevents egress of viscoelastic (OVD) during capsulorrhexis formation, reducing the risk of errant peripheral migration during this critical step. Smaller incisions limit iris prolapse, especially in cases of intraoperative floppy iris syndrome (IFIS), so that altered pupil contour and suboptimal postsurgical cosmesis can be avoided. Smaller incisions allow for better fluidics control and fragment followability, leading to reduced applied phaco power and improved postoperative visual acuity and corneal optical quality.5,6
Figure 11-2. Stellaris tubing design and reduction of surge with advanced modules. (Reprinted with permission from Bausch & Lomb.)
However, if wound construction is not performed properly, then thermal injury, fluid leakage, induced astigmatism, iris prolapse, and oar-locking of instruments are all possible. In this regard, close attention to incision location, size, shape, and self-sealing ability is required. The authors utilize a posterior clear cornea or “near clear” limbal incision site, so as to avoid conjunctival chemosis and anterior entry of the incision, which can interfere with intraoperative visibility from fluid pooling on the ocular surface or induction of corneal warpage during intraocular maneuvers, respectively. The placement of incisions should generally coincide with comfortable positioning of the hands, avoiding regions with prominent orbital rim structure or proximal to lid speculum wires or blades. The sizing of the incision should match phaco instrument size, to avoid leakage of infusion fluid around the phaco instruments. However, if the incision is too narrow, it will hamper instrument insertion and maneuverability while stretching and distorting the incision. A narrow incision may also impinge the irrigation sleeve in C-MICS, leading to chamber instability and increasing the risk of thermal wound injury. An incision that has been stretched or heated may not seal properly. In order to prevent such complications, surgeons have utilized different incision instruments to assist with reproducible, square wound construction, including trapezoidal steel (Figure 11-3A), sapphire, and diamond knives (Figure 11-3B). The application of femtosecond lasers for use in cataract surgery may further facilitate and standardize MICS incisions.
Figure 11-3. (A) Trapezoidal steel, (B) sapphire (top), and diamond (bottom) knives.
Typical incision sizes for B-MICS are 1.2 mm for 20-gauge instruments and 1.4 mm for 19-gauge instruments. One incision in B-MICS may need to be enlarged to allow for IOL insertion. C-MICS typically involves a 1.8- to 2.0-mm incision. The incision tunnel can be created by direct entry or in steps. At the end of the procedure, all corneal incisions should be hydrated with BSS and tested to be watertight. Application of fluorescein to the wounds to show that they are Seidel negative is also a sound consideration, particularly for specialty IOLs.
CAPSULORRHEXIS AND HYDRODISSECTION
Microincision size requires revision of capsulorrhexis forceps size as well. Several designs have been developed by companies such as Storz, Katena, and MST, using both Utrata-style as well as coapting microincision instruments. Care must be taken during anterior capsulotomy formation to ensure that the micro-forceps do not become oar-locked during lateral or circular movement. This can be avoided by extending the wound slightly as the blade is exiting the incision site, regrasping the rhexis more frequently, and using a rotational wrist motion. Otherwise, there may be contact with the incision borders, translating into corneal distortion and OVD leakage. Such issues may be obviated by use of a femtosecond laser–assisted capsulorrhexis.
As the smaller incision limits OVD leakage, MICS procedures usually have a more controlled chamber. There is, therefore, usually less of a chance of a peripheral tear during MICS capsulorrhexis formation, affording an increased safety margin. However, it is important to wash out some OVD prior to full hydrodissection, as the small incision size and denser OVD may block the egress of fluid, increasing anterior chamber pressure that can then be directed to the posterior capsule, causing capsular blowout.
ULTRASOUND POWER MODULATION
Varying the power modulation during phacoemulsification can reduce ultrasound energy exposure to ocular tissues and improve the efficiency of cataract surgery. Pulsing of ultrasound (Table 11-2) and/or adjusting the duty cycle of phaco power on to phaco power off can allow for safer removal of denser nuclei by reducing the overall phaco time, thereby decreasing the risk of endothelial damage and wound burn. Miyoshi and Yoshida7 demonstrated though ultra-high-speed digital video imaging that micropulse (8 ms on, 4 ms off) allowed for nuclear fragments to remain engaged and rotate at the tip, rather than being pushed away by the ultrasound pulse. The use of torsional phacoemulsification (Infiniti) can additionally diminish the delivered energy and improve followability.8
BIMANUAL MICROINCISIONAL CATARACT SURGERY
Bimanual MICS involves 2 microincisions of the same diameter. In addition to the advantages of fluidics with smaller incisions, as outlined previously, this technique allows for greater intraoperative maneuverability, as the irrigation and aspiration hand pieces can be used via either port. This is particularly helpful in subincisional removal of nuclear and cortical material. It also allows for more thorough anterior and posterior capsular polishing, which is important for the stability of hinged-IOLs, such as the Crystalens, and in centration and stability of multifocal and toric IOLs. Bimanual techniques allow the use of irrigating choppers available through many companies such as Katena and MST (Figure 11-4).9 B-MICS has been espoused for challenging cases,10 such as for IFIS, miotic pupils, traumatic cataracts, pseudoexfoliation, posterior polar cataracts,11 vitrectomy,12 and combined glaucoma cases.
TRANSITIONING TO MICROINCISIONAL CATARACT SURGERY
The advantages of MICS do not require significant modification of the surgeon’s phacoemulsification techniques, whether they range from divide and conquer to horizontal or vertical chopping. In fact, MICS fluidics, greater chamber stability, and pulsed phaco allow for a relatively seamless transition to MICS.
Table 11-2
Coaxial Microincisional Cataract Surgery Settings With Stellaris, 1.8-mm Incision, Gas-Forced Infusion (50 mm Hg), and Venturi Pump
Abbreviations: pps, pulses per second; dc, duty cycle.
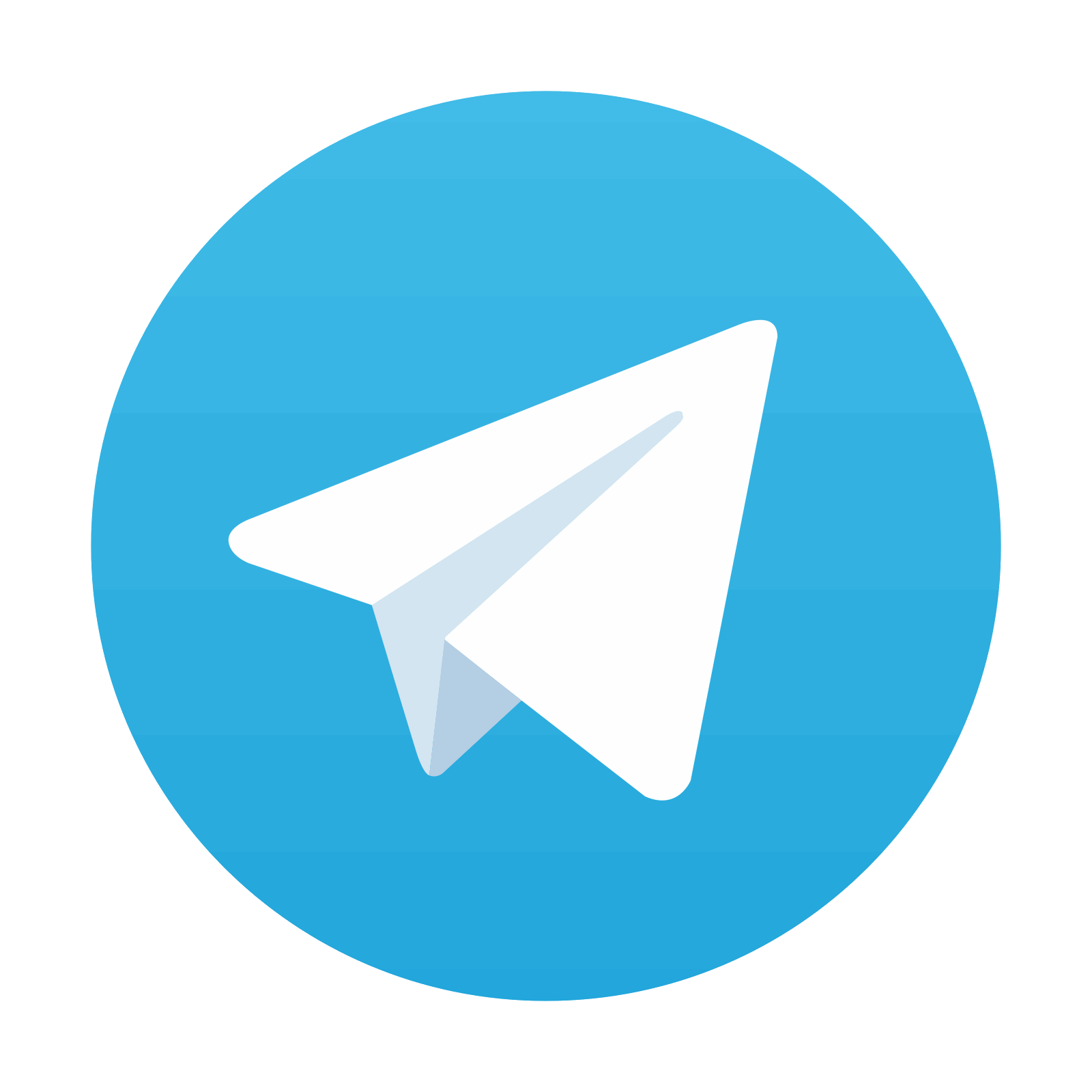
Stay updated, free articles. Join our Telegram channel

Full access? Get Clinical Tree
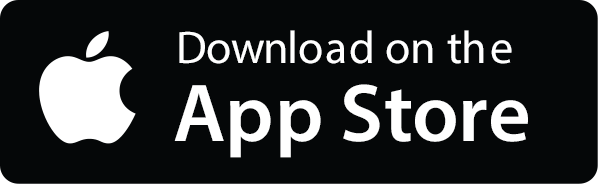
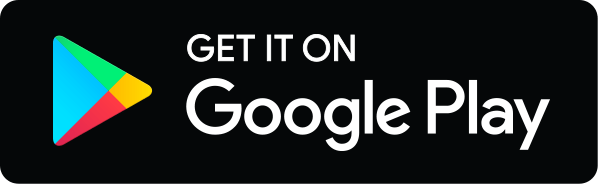