Lipofuscin of the Retinal Pigment Epithelium
Michael E. Boulton
Lipofuscin accumulation is strongly associated with retinal aging and the progression of age-related macular degeneration (AMD) (1). Such lipofuscin accumulation is typical of highly metabolic, postmitotic cells in a variety of aging mammalian tissues (e.g., liver, heart, brain, testes, and retina) that accumulate nondegradable lipid-protein aggregates within lysosomes (2, 3, 4). In the retinal pigment epithelium (RPE), lipofuscin can be derived from autophagy (degradation of spent intracellular organelles such as mitochondria, Golgi, and endoplasmic reticulum) (1,2,5) or the ingestion of photoreceptor outer segments (POS) (2,6). The failure of the RPE lysosomal system to completely degrade this material is probably the result of preoxidative modifications. The high level of lipofuscin in the RPE leads to cellular dysfunction, which in turn contributes to photoreceptor dysfunction and degeneration (1,4).
RETINAL PIGMENT EPITHELIUM LIPOFUSCIN
RPE lipofuscin granules accumulate in the central to basal portion of the cell as spherical membrane-bound granules of 1-2 μm diameter (Fig. 2.1) (7). In addition, in aged donors lipofuscin can be observed complexed with melanin-forming aggregates of heterogeneous composition and size, in which case they are termed melanolipofuscin. Ex vivo and in vivo analyses demonstrate an age-related increase in lipofuscin content, up until the age of about 70 years (6,8, 9, 10). Thereafter, lipofuscin levels plateau or even decline (9). The reason for this decline is not clear, but it may involve one or a combination of lipofuscin degradation routes, e.g., voiding into the extracellular space (as basal laminar deposits or precursor material to drusen) or association with melanosomes. Concomitant with the accumulation of lipofuscin granules are a decrease in melanosomes and an increase in melanolipfuscin granules (8). On the basis of early morphometric data, the accumulation of RPE lipofuscin was erroneously considered to be bimodal (6), but it is now apparent that such accumulation is linear—at least up until the later decades, when the effects of age-related pathology begin to have an impact (Fig. 2.2) (9). Lipofuscin demonstrates a regional distribution across the fundus (9, 10, 11) (see Chapter 3). The distribution of lipofuscin demonstrates a retinal topography similar to that of rod photoreceptors, suggesting that ingested POS are a major substrate for RPE lipofuscin (11). Alternatively, the highest density of photoreceptors will require the highest level of metabolic activity for the RPE, and thus mitochondrial turnover and autophagy will be much higher.
The cytoplasmic volume occupied by lipofuscin increases significantly with age (Fig. 2.1). Feeney-Burns and colleagues demonstrated that 8% of the cytoplasmic volume of the central RPE is occupied by lipofuscin granules by 40 years of age, and this increases to 19% by 80 years of age (8). It is likely that this would affect metabolic function, intracellular compartmentalization of organelles, and lysosomal activity, reducing the functional capacity of the RPE with increasing age. In old age, the cytoplasmic volume occupied by the equatorial and peripheral RPE is considerably less than that in the central retina.
In addition to the regional distribution of lipofuscin across the fundus, there is considerable cell-to-cell heterogeneity in lipofuscin density in any particular region of the retina (Fig. 2.1) (12). It is evident that two adjacent cells (presumably with the same functional and metabolic load) can have high, intermediate, or minimal levels of lipofuscin. This would support the proposal by Burke and Hjelmeland (12) that mosaicism of the RPE is a natural result of normal mechanisms regulating gene expression during development and postnatal aging. This heterogeneity may help explain the highly focal nature of the lesions associated with pathologies such as AMD.
Among the different tissue lipofuscins, RPE lipofuscin has a number of unique properties that determine its photophysical properties and its potential for contributing to RPE dysfunction, as will be discussed in more detail below. First, RPE lipofuscin is derived at least in part from POS and thus contains significant quantities of retinoids (4,13), including the diretinal conjugate A2E (4). Second, RPE lipofuscin is a broadband absorber that is constantly exposed to visible light and is located in a highoxygen environment, which makes it an ideal substrate for retinal photodamage (1).
LIPOFUSCIN GENESIS AND COMPOSITION
Although RPE lipofuscin is believed to result from the incomplete degradation of POS and autophagy of spent or damaged intracellular organelles, the relative contributions of these pathways remain unknown. It is thought that prior oxidative damage to these substrates renders them undegradable by the lysosomal system, the universal intracellular degradation machinery that contains over 40 hydrolytic enzymes. However, prior oxidation does not account for the fluorescent properties of lipofuscin, since Eldred and Katz (14,15) showed that the autofluorescent products of lipid peroxidation differ from those of lipofuscin. Although we have gained an understanding of the initial substrates involved in lipofuscin genesis, we still have a limited understanding of the composition of the mature lipofuscin granules. Probably the first attempt at analysis was made by Eldred and Katz (13), who used Folch’s extraction to separate lipofuscin into three fractions: chloroform-soluble, methanol-soluble, and insoluble (Fig. 2.3A). Thin-layer chromotography analysis of the chloroform fraction revealed 10 discrete fluorophores: two green-emitting, which comigrated with retinol and retinyl palmitate; three emitting yellow/green; and one golden-yellow and four orange-red fluorophores, the most prominent of which was subsequently identified as A2E (Fig. 2.3) (16). Further studies by other researchers identified the fluorophores as retinoid derivatives (4,17). This was followed by many studies on the role of A2E, the only readily synthetic component of lipofuscin, and demonstrations of its phototoxic and lysosomotrophic properties. However, the content of the bulk (>70%) of lipofuscin material, which is located at the insoluble interface on Folch’s extraction, has largely been ignored (18).
It has long been considered that lipofuscin consists of cross-linked oxidatively modified lipids, proteins, and sugars, with the protein content ranging from 10% to 70% of the lipofuscin granule. The first proteome for RPE lipofuscin was reported by Schutt and colleagues (19) in 2002. They identified over 65 abundant cellular proteins, including cytoskeleton proteins, proteins of transduction, enzymes of metabolism, proteins of the mitochondrial respiratory chain, ion channel proteins, and chaperones, which supported the origin of lipofuscin as a combination of photoreceptor phagocytosis and autophagy. Furthermore, analysis of the samples revealed that many of
these proteins were damaged by the aberrant covalent modifications of MDA, 4-HNE and AGEs (20). In a subsequent proteome analysis of RPE lipofuscin, Warburton et al. (21) identified 41 cellular proteins; surprisingly, only 11% these proteins had been identified in the proteomic analysis of Schutt and colleagues (19). Although there are numerous explanations for this discrepancy, the likely problem lies in the purity of the starting material. Lipofuscin is generally isolated by repeated sucrose density gradient centrifugation (19,21,22); however, there is usually some residual contamination by
cellular debris (22). While investigating this further, we demonstrated that highly purified debris-free RPE lipofuscin granules contain little or no protein, but do contain significant amounts of modified material, such as carboxyethylpyrrole adducts (23). In conclusion, although our understanding of the composition of lipofuscin in the RPE remains limited, it does appear that protein makes only a very small contribution, if any, and that oxidized and modified lipids from outer-segment and mitochondrial membranes may be major contributors.
these proteins were damaged by the aberrant covalent modifications of MDA, 4-HNE and AGEs (20). In a subsequent proteome analysis of RPE lipofuscin, Warburton et al. (21) identified 41 cellular proteins; surprisingly, only 11% these proteins had been identified in the proteomic analysis of Schutt and colleagues (19). Although there are numerous explanations for this discrepancy, the likely problem lies in the purity of the starting material. Lipofuscin is generally isolated by repeated sucrose density gradient centrifugation (19,21,22); however, there is usually some residual contamination by
cellular debris (22). While investigating this further, we demonstrated that highly purified debris-free RPE lipofuscin granules contain little or no protein, but do contain significant amounts of modified material, such as carboxyethylpyrrole adducts (23). In conclusion, although our understanding of the composition of lipofuscin in the RPE remains limited, it does appear that protein makes only a very small contribution, if any, and that oxidized and modified lipids from outer-segment and mitochondrial membranes may be major contributors.
![]() FIGURE 2.3. Lipofuscin composition and photoreactivity. (A) Photograph of lipofuscin after Folch’s extraction. The top layer is the methanol/water phase, the bottom layer is the chloroform-soluble phase, and insoluble material is located at the interface. (B) Comparison of the action spectra of initial rates of photo-induced oxygen uptake in suspensions of DMPC liposomes containing both the chloroform-soluble (Δ) and insoluble (∇) interfacial material plus a combination (“reconstituted lipofuscin”, ◊). The arithmetic addition (+) is shown of the rates measured separately for the chloroform-soluble and chloroform-in-soluble fractions. The inset shows the change in content of dry mass of the chloroform-soluble (○) and chloroform-insoluble (▪) material extracted from lipofuscin granules as a function of donor age. Horizontal bars: SD of the donors of the pooled samples; vertical bars: SD of the dry mass measurements (reproduced courtesy of Investigative Ophthalmology and Visual Science from Ref. 18). (C) Comparison of the thin-layer chromatography profile of the chloroform-soluble fraction of pooled lipofuscin granules from donors of different ages: 1) 50-59 years; 2) 60-69 years; 3) 70-79 years. The fractions show a number of different fluorophores when excited by UV irradiation. |
Spectral Characteristics of RPE Lipofuscin
RPE lipofuscin granules exhibit a broad and wavelength- dependent absorption spectrum with a decrease in absorption toward increasing wavelengths (22). The excitation and emission spectra of lipofuscin granules are shown in Figure 2.4, and a summary of excitation and emission peaks is provided in Table 2.1. RPE lipofuscin granules typically exhibit four main regions of interest in the emission spectra when excited at 364 nm: the main peak located at 600-610 nm, a blue-green shoulder located at 470 nm, a green-yellow shoulder at 550 nm, and, in the case of lipofuscin from individuals over 50 years, a far red shoulder at 680 nm. Similar emission peaks are observed at 500, 610, and 680 nm when lipofuscin is excited at 476 nm, which is similar to the excitation employed by in vivo autofluorescence (AF) systems (≥488 nm) (24,25). Excitation spectra
with emissions monitored at 610 nm or 570 nm show a main excitation peak at 470 nm, with two subsidiary peaks at 370 and 405 nm (22). Time-resolved fluorescence microscopy has identified four decay components in lipofuscin with decay times of approximately 0.21, 0.65, 1.75, and 6.6 ns (26). Wavelength-resolved decay analysis demonstrated that the three main fluorescing components of lipofuscin do not strongly interact and thus appear to be excited directly. The spectral characteristics of melanolipofuscin are intermediate between those of lipofuscin and melanin.
with emissions monitored at 610 nm or 570 nm show a main excitation peak at 470 nm, with two subsidiary peaks at 370 and 405 nm (22). Time-resolved fluorescence microscopy has identified four decay components in lipofuscin with decay times of approximately 0.21, 0.65, 1.75, and 6.6 ns (26). Wavelength-resolved decay analysis demonstrated that the three main fluorescing components of lipofuscin do not strongly interact and thus appear to be excited directly. The spectral characteristics of melanolipofuscin are intermediate between those of lipofuscin and melanin.
![]() FIGURE 2.4. Excitation and emission spectra of intact lipofuscin granules isolated from donors of different ages. Excitation spectra were monitored with emission performed at 570 nm and emission spectra were monitored with excitation performed at 364 nm. Lipofuscin granules were prepared from different age groups: (A) 5-29 years; (B) 30-49 years; (C) >50 years. Excitation and emission spectra are expressed in arbitrary units (A.U.). (Modified from Ref. 22.) |
TABLE 2.1 Summary of the Main Fluorescence Peaks for Lipofuscin Granules From Different Age Groups Observed in Figure 2.4. Excitation spectra were monitored with emission performed at 570 nm, and emission spectra were monitored with excitation performed at 364 nm. | ||||||||||||||||||||
---|---|---|---|---|---|---|---|---|---|---|---|---|---|---|---|---|---|---|---|---|
|
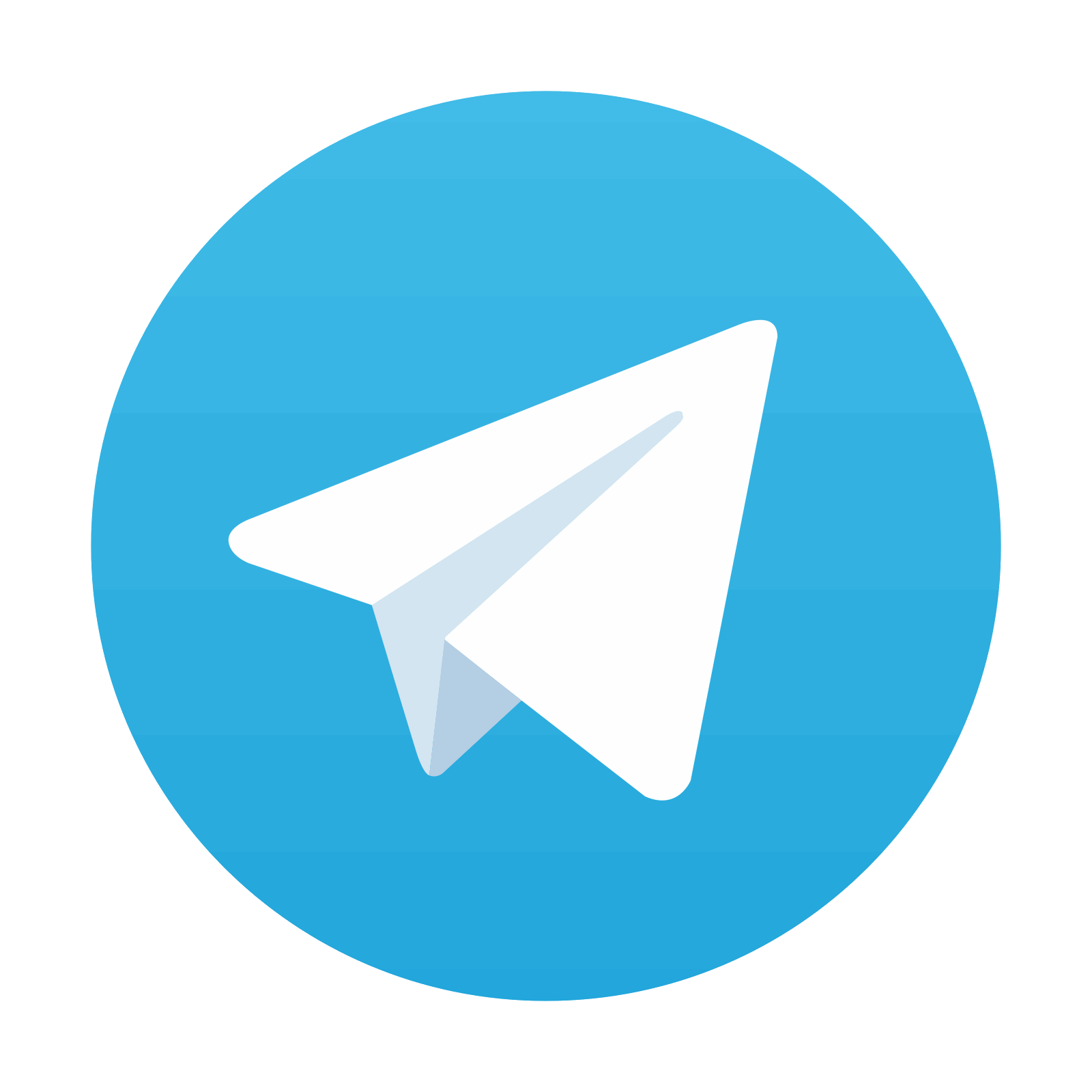
Stay updated, free articles. Join our Telegram channel

Full access? Get Clinical Tree
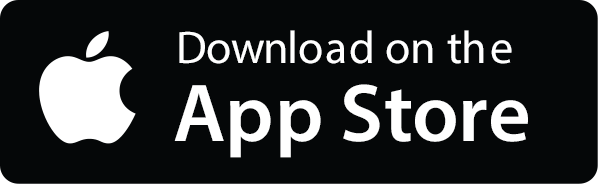
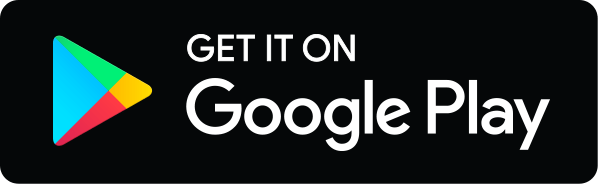