This article provides an introduction to radiation therapy as it applies to intracranial tumors. It also provides a review of the natural growth progression of acoustic neuromas and accuracy of tumor size determination. Literature on the use of linear accelerator stereotactic radiosurgery and fractionated radiotherapy in acoustic neuroma management is reviewed and summarized. Specifically, the rates of reported tumor control, hearing preservation, facial and trigeminal nerve complications, and hydrocephalus are analyzed. Although the complication rates associated with linear accelerator therapy are relatively low, hearing preservation is poor and acoustic neuroma control is variable.
Acoustic neuromas (ANs) are benign, slow-growing tumors that arise from the Schwann cells of the vestibulocochlear nerve. Although these tumors are benign, their expansion in the internal auditory canal and cerebellopontine angle compresses the cranial nerves and the brainstem . Once diagnosed, the tumors are commonly resected microsurgically, managed conservatively with radiologic surveillance, or treated with radiation therapy . This article focuses on the effectiveness of linear accelerator (LINAC) stereotactic radiosurgery and radiotherapy in AN treatment.
Radiation biology
Radiation-induced damage to the cell machinery has been used to control the proliferation of tumors. Interruption of cell division is a desired effect in cells that have lost the ability to respond to appropriate internal and external surveillance because of mutation. The therapeutic role of ionizing X-rays and gamma rays lies in their ability to compromise the integrity of DNA and other important biologic molecules of the target cell. Specifically, excitation of electrons in molecules exposed to radiation produces reactive, free radicals, which in turn degrade structures that are important for cell survival. Double strand breaks in the DNA are most detrimental to the cell. Effective mechanisms for fixing the double strand breaks, which include nonhomologous end joining and a more precise homologous recombination, are often inadequate to deal with the overwhelming damage to the genetic material, especially in highly mutated tumor cells. These lesions prevent the cell from completing the replication cycle and arrest the growth of the tumor. Excessive radiation damage to DNA and the arrest of mitosis lead to induction of the apoptotic—or programmed—cell death pathway. Radiation damage to phospholipids in the membrane of the cell also acts to trigger cascades that lead to cell death .
There are two types of ionizing radiation: electromagnetic, which consists of photons or packets of energy, and particulate radiation. The common feature of these forms of radiation is ionization, a process that ejects an excited electron from the target atom. This process occurs when a photon or particle transfers its energy to the target tissue. X-rays and gamma rays are indirectly ionizing, whereas charged particles are directly ionizing. X-rays and gamma rays are types of electromagnetic radiation and differ in that an X-ray is a result of a collision between an electron and a target, whereas gamma rays are produced when the contents of the nucleus of an atom return to their initial energy state from an excited level, a process known as gamma decay. As these electromagnetic waves pass through tissue, they are absorbed and produce fast recoil electrons. As this occurs, they lose intensity.
Protons are heavy charged particles that cause damage directly. They have an opposite charge from electrons and have considerably more mass. When a proton beam strikes a target, it deposits almost all of its energy at the end of its range. This characteristic, known as the Bragg peak, can be exploited to deliver high doses to tumors with almost no fall-off of radiation. Helium ions act similarly. Neutrons have no charge and are equivalent to a proton in mass. They are not affected by an electric field. They are also indirectly ionizing, and after interacting with matter they produce recoil protons and alpha particles. Although all other radiation modalities work best on parts of tumors in which oxygen is available, neutron radiation provides an opportunity to target the center of large tumors that are oxygen deficient and types of tumors known to be poorly aerated .
Radiosurgery (RS) is a term used for the delivery of ionizing radiation to an intracranial target with the use of stereotactic technique. The exposure of normal, healthy tissues to radiation should be limited, yet significant doses must be delivered to the tumor to have a therapeutic effect. Stereotactic technique facilitates the accomplishment of these goals through accurate localization and targeting of the lesion. The target volume is represented in a three-dimensional space with the use of markers on a fixed frame, the location of which is electronically linked with either CT or MRI scans. Fig. 1 shows the planning of a treatment based on the MRI of a patient with AN. Special care must be taken to isolate and protect the brainstem, which is in close proximity to the target volume. The three-dimensional target created is irradiated from several angles; beams all meet and deliver a high cumulative dose of radiation to a single isocenter. For irregularly shaped targets, more than one isocenter can be used. Three modalities are used to deliver radiation in stereotactic RS .
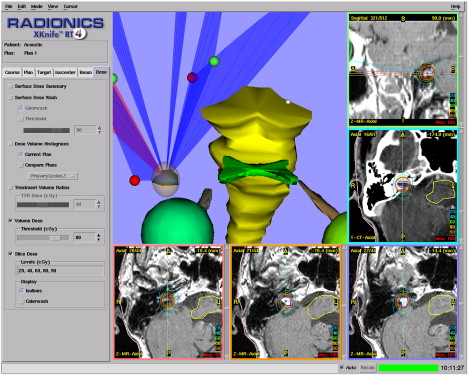
As of 2003, approximately 200,000 patients were treated with gamma knife technology. Gamma knife contains 201 cobalt 60 sources that are collimated using a helmet fitted for patients. Once the treatment is planned using the three-dimensional representation of the target, a patient’s head is fixed and the beams from the sources align on multiple isocenters .
LINAC is a tool that has long been used to generate X-rays. LINAC uses electromagnetic waves of microwave frequency to accelerate electrons. This first became possible with technology borrowed from high-energy microwave generators used in military radars during World War II. LINAC was first used for radiotherapy in 1953 . More recently, in the early 1980s, it was adapted for use in stereotactic RS. Many LINAC units have been modified for RS, whereas others are designed specifically for this use. It is estimated that more than 30,000 people have undergone LINAC-based RS. LINAC delivers a photon beam of high-energy X-rays through a series of arcs or fixed static fields. Conformality is maximized with the use of micro-multileaf collimators. Conformality can be enhanced further with the use of intensity modulated RS, which varies the intensity of dose within a field to treat tumor and spare normal tissue.
Another modality that has been used in RS over the past 40 years is particle beam irradiation. Two to six beams of charged particles are focused on the target. One example is the use of protons in radiation of intracranial tumors. As in the other two modalities used for RS, the radiation dose that reaches structures outside the target volume is minimized by the effect of the Bragg peak .
Stereotactic radiotherapy, or fractionated stereotactic radiotherapy (FSRT), is an alternative to RS. It differs in that the full radiation dose is not administered at one time but is instead divided into several doses. The accuracy of irradiation is reproduced with the use of a head frame, such as the GTC (Gill Thomas Cosman) ( Fig. 2 ), which ensures that the treatment is consistently targeted each time. A bite plate may be used to individualize the frame for each patient and achieve reproducibility with multiple treatments (see Fig. 2 ; Fig. 3 ). Using smaller doses over many fractions reduces late side effects, such as hearing loss, when compared with single fraction RS .
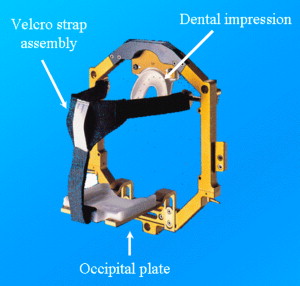
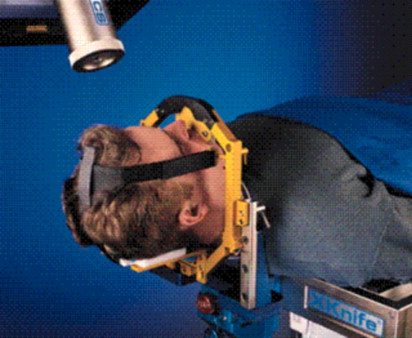
Natural history of acoustic neuromas
When evaluating the effect of radiation therapy on the growth of ANs, the natural history of these tumors must be considered. One of the options used in the management of patients who have ANs is radiologic surveillance of the tumors with repeat MRI scans. Since the introduction of gadolinium-enhanced MRI, tumors of smaller size can be detected and followed over time. A significant amount of data on the natural history of AN tumors has been collected from patients who choose this conservative option.
A review of studies on the surveillance of ANs was published by Selesnick and Johnson in 1998. The authors presented a meta-analysis of 13 studies on the topic published between 1985 and 1997. In all, 571 patients across the studies evaluated were followed for an average of 36 months. The mean diameter of tumors at the beginning of the surveillance was 11.8 mm, and 54% of the tumors (range 14%–74%) grew at an average rate of 1.8 mm per year. Slightly less than half of the patients included in the meta-analysis had no AN growth at a mean follow-up of 3 years. Among the patients whose tumor size increased ( n = 178), the growth rate was 4 mm per year. Initial size of the tumors and the age of the patients were not found to be predictive of growth of AN.
A more recent meta-analysis published by Yoshimoto in 2005 reviewed 26 studies examining the natural course of AN growth published between 1991 and 2002. In the 1340 patients included, the mean initial tumor diameter was 11 mm, and 46% (range 15%–85%) had an increase in tumor size during the mean follow-up period of 38 months (range 6–64 months). A mean growth rate of 1.2 mm per year was calculated from the 16 studies ( n = 964) that contained this information. Eight percent of patients had a spontaneous decrease in tumor size. The author also analyzed a subgroup of 12 studies that used only MRI and not CT for tumor surveillance. In this group, 39% of tumors increased in size in the mean follow-up time of 33 months.
Since the Yoshimoto’s review, Stangerup and colleagues reported on 552 patients from a single institution who had ANs not associated with neurofibromatosis type 2 (NF2) and who underwent radiologic surveillance of tumors with repeated MRI scans. The data were collected for the patient population managed from 1975 to June 2005, and the mean follow-up was 43 months (range 12–180 months). Tumors were characterized as either intrameatal or extrameatal and were analyzed separately. Of the 230 intrameatal tumors, 17% grew to extend extrameatally. Growth occurred 64% of the time during the first year (growth rate, 10.32 mm/y), 23% during the second year (growth rate, 3.83 mm/y), 5% during the third year (growth rate, 2.17 mm/y), and 8% during the fourth year of observation (growth rate, 0.92 mm/y). Extrameatal tumors observed in 322 patients were defined as growing when the largest diameter increased by 2 mm and were defined as shrinking when the diameter decreased by 2 mm. In total, 70.2% of tumors remained stable, 28.9% grew, and 0.9% shrank. Sixty-two percent of growth occurred during the first year (growth rate, 4.90 mm/y), 26% occurred during the second year (growth rate, 2.79 mm/y), 10% occurred during the third year (growth rate, 1.15 mm/y), and 2% occurred during fourth year of follow-up (growth rate, 0.75 mm/y). The difference between growth of intrameatal and extrameatal ANs of 17% and 28.9%, respectively, was reported to be statistically significant. Intrameatal or extrameatal tumor growth was not observed after the fourth year of follow-up.
It is difficult to compare AN growth rates with and without radiosurgical intervention because of differences in follow-up time and methods used to report tumor size and control. Battaglia and colleagues addressed the issue by comparing 111 patients followed radiologically for an average of 38 months at a single institution with data reported in the literature on radiosurgical treatment of AN. Only patients treated with the maximum marginal dose of 12 to 13 Gy administered in a single treatment with any radiosurgical modality were included in the analysis. Mainly, data from studies in which gamma knife surgery was used were represented. Only studies that reported a mean or median follow-up of 24 months were used for comparison. The natural tumor growth rate reported by the authors was 0.7 mm/y, with 18% of tumors growing faster than 1 mm/y and 13% growing faster than 2 mm/y. On average, change in size was noted at 2.2 years after surveillance was initiated. No growth during the follow-up period was noted in 50.5% of the patients. Tumor control in RS studies selected by the authors was defined as no growth more than 2 mm/y or more than 1 mm in two dimensions. Using this same definition, 87% of ANs were controlled by conservative management, a result not significantly different from when the tumors were treated with radiation.
There is some indication that AN tumors do not grow in a linear fashion. In 2000, Tschudi and colleagues reported growth followed by stabilization in some of the patients whose tumors were observed over time. Charabi and colleagues described a group of cases in which the tumor size was stable for an average of 19 months and then experienced a period of growth. This irregularity in the natural progression of the tumors suggests that a period of stability does not necessarily predict long-term control, whereas a period of growth does not predict continuous enlargement.
The fact that the natural history of AN growth undergoes periods of quiescence is important when trying to judge the impact of radiation therapy on these tumors.
Natural history of acoustic neuromas
When evaluating the effect of radiation therapy on the growth of ANs, the natural history of these tumors must be considered. One of the options used in the management of patients who have ANs is radiologic surveillance of the tumors with repeat MRI scans. Since the introduction of gadolinium-enhanced MRI, tumors of smaller size can be detected and followed over time. A significant amount of data on the natural history of AN tumors has been collected from patients who choose this conservative option.
A review of studies on the surveillance of ANs was published by Selesnick and Johnson in 1998. The authors presented a meta-analysis of 13 studies on the topic published between 1985 and 1997. In all, 571 patients across the studies evaluated were followed for an average of 36 months. The mean diameter of tumors at the beginning of the surveillance was 11.8 mm, and 54% of the tumors (range 14%–74%) grew at an average rate of 1.8 mm per year. Slightly less than half of the patients included in the meta-analysis had no AN growth at a mean follow-up of 3 years. Among the patients whose tumor size increased ( n = 178), the growth rate was 4 mm per year. Initial size of the tumors and the age of the patients were not found to be predictive of growth of AN.
A more recent meta-analysis published by Yoshimoto in 2005 reviewed 26 studies examining the natural course of AN growth published between 1991 and 2002. In the 1340 patients included, the mean initial tumor diameter was 11 mm, and 46% (range 15%–85%) had an increase in tumor size during the mean follow-up period of 38 months (range 6–64 months). A mean growth rate of 1.2 mm per year was calculated from the 16 studies ( n = 964) that contained this information. Eight percent of patients had a spontaneous decrease in tumor size. The author also analyzed a subgroup of 12 studies that used only MRI and not CT for tumor surveillance. In this group, 39% of tumors increased in size in the mean follow-up time of 33 months.
Since the Yoshimoto’s review, Stangerup and colleagues reported on 552 patients from a single institution who had ANs not associated with neurofibromatosis type 2 (NF2) and who underwent radiologic surveillance of tumors with repeated MRI scans. The data were collected for the patient population managed from 1975 to June 2005, and the mean follow-up was 43 months (range 12–180 months). Tumors were characterized as either intrameatal or extrameatal and were analyzed separately. Of the 230 intrameatal tumors, 17% grew to extend extrameatally. Growth occurred 64% of the time during the first year (growth rate, 10.32 mm/y), 23% during the second year (growth rate, 3.83 mm/y), 5% during the third year (growth rate, 2.17 mm/y), and 8% during the fourth year of observation (growth rate, 0.92 mm/y). Extrameatal tumors observed in 322 patients were defined as growing when the largest diameter increased by 2 mm and were defined as shrinking when the diameter decreased by 2 mm. In total, 70.2% of tumors remained stable, 28.9% grew, and 0.9% shrank. Sixty-two percent of growth occurred during the first year (growth rate, 4.90 mm/y), 26% occurred during the second year (growth rate, 2.79 mm/y), 10% occurred during the third year (growth rate, 1.15 mm/y), and 2% occurred during fourth year of follow-up (growth rate, 0.75 mm/y). The difference between growth of intrameatal and extrameatal ANs of 17% and 28.9%, respectively, was reported to be statistically significant. Intrameatal or extrameatal tumor growth was not observed after the fourth year of follow-up.
It is difficult to compare AN growth rates with and without radiosurgical intervention because of differences in follow-up time and methods used to report tumor size and control. Battaglia and colleagues addressed the issue by comparing 111 patients followed radiologically for an average of 38 months at a single institution with data reported in the literature on radiosurgical treatment of AN. Only patients treated with the maximum marginal dose of 12 to 13 Gy administered in a single treatment with any radiosurgical modality were included in the analysis. Mainly, data from studies in which gamma knife surgery was used were represented. Only studies that reported a mean or median follow-up of 24 months were used for comparison. The natural tumor growth rate reported by the authors was 0.7 mm/y, with 18% of tumors growing faster than 1 mm/y and 13% growing faster than 2 mm/y. On average, change in size was noted at 2.2 years after surveillance was initiated. No growth during the follow-up period was noted in 50.5% of the patients. Tumor control in RS studies selected by the authors was defined as no growth more than 2 mm/y or more than 1 mm in two dimensions. Using this same definition, 87% of ANs were controlled by conservative management, a result not significantly different from when the tumors were treated with radiation.
There is some indication that AN tumors do not grow in a linear fashion. In 2000, Tschudi and colleagues reported growth followed by stabilization in some of the patients whose tumors were observed over time. Charabi and colleagues described a group of cases in which the tumor size was stable for an average of 19 months and then experienced a period of growth. This irregularity in the natural progression of the tumors suggests that a period of stability does not necessarily predict long-term control, whereas a period of growth does not predict continuous enlargement.
The fact that the natural history of AN growth undergoes periods of quiescence is important when trying to judge the impact of radiation therapy on these tumors.
Tumor size measurement
The current, preferred method for measuring the size of AN tumors involves the use of MRI. Accuracy and precision are important in determining the initial size of the tumor at presentation and evaluating tumor changes. The consistency of these measurements and the precision with which tumor progression can be followed have limitations.
In 2003, Slattery and colleagues addressed the issue of reliability of MRI-based measurement. Factors that contribute to the quality of the scan include the MRI machine used, how the image is acquired, the training of the technician and the radiologist, whether contrast is administered, what dimension is used to measure the size of the tumor, and how much the patient moves during the scan.
A large source of variability could originate in the method of measurement. The radiologist can measure a distance by eye or use computer software. (The latter approach is considered more precise.) Studies evaluated in our review varied with respect to what constitutes the size of the tumor. Greatest diameter of the AN was commonly used. The American Academy of Otolaryngology–Head and Neck Surgery recommends that two measurements be taken: one parallel and one perpendicular to the petrous ridge. Computer algorithms allow tumor volume to be calculated to evaluate size. Each of these methods varies in its accuracy and precision.
To answer a question of how much consistency there is among data obtained from MRI scans and what is the minimum amount of change that can be detected in tumor size, Slattery and colleagues performed six scans on three different MRI machines (two consecutive scans on each) for seven patients with NF2. T1-weighted postgadolium-enhanced gradient three-dimensional sequences were used in this study. In total, 20 meningiomas and ANs were used to evaluate inter- and intrascanner reliability. The volume and the greatest diameter were measured using computer software. The authors reported that calculated tumor size was consistent across the two studies conducted on one machine and across the three machines used. They concluded that the minimal difference in tumor diameter that can be detected on an MRI is 1.1 mm, whereas the minimum reliable volume difference is 0.15 mL. The authors suggested that a more consistent protocol be used to measure tumor size in patients with AN.
This finding is important when judging the effect of radiation on acoustic tumors, because small changes in size may not be appreciated or may be overread.
Methods
For the purpose of this article, MEDLINE was searched for studies using LINAC for AN treatment. The terms “vestibular schwannoma,” “acoustic neurilemmoma,” “acoustic neurinoma,” “acoustic neurilemoma,” “acoustic schwannoma,” and “acoustic neuroma” were crossed with “radiosurger ∗ ,” “stereotactic radiosurger ∗ ,” “linear accelerator,” “LINAC,” “linear accelerator-based surger ∗ ,” and “X knife.” (The use of [ ∗ ] after a word indicates that all possible endings of the word were incorporated in the search.) Only articles published in English since 1996 were considered for analysis. The abstracts were screened to exclude articles pertaining to the use of gamma knife and other stereotactic radiation modalities. The references of selected articles were reviewed for additional relevant sources. Articles from a singles institution reporting on redundant patient populations were identified, and the more recent and complete studies were chosen for analysis.
Radiosurgery
In one of the earliest publications on LINAC RS for ANs, Chakrabarti presented 11 patients in whom surgery failed to control the tumors and who were treated with stereotactic radiation. The diameter of the tumors ranged from 15 to 35 mm (mean 26 mm), and the lesions were targeted with 12.5 to 20 Gy administered to the 90% isodose contour. Ten patients were followed radiologically with repeat CT or MRI scans or both for 3 to 36 months (mean 15 months). Seven of the patients had observable necrosis in the center of the tumor 6 months after RS. During the period of 7 to 20 months after treatment, tumors decreased in size in 4 patients. No change was observed in four cases, and 1 mm increase was seen in 2 patients. Hearing was diminished in 7 patients before treatment; it was stabilized or improved in 5 of these patients, whereas 2 patients became completely deaf. Although no new facial palsy was found after RS, 4 patients experienced it before treatment. In 2 patients, their pre-existing facial palsy was unchanged; it improved in 1 patient at 14 months after RS; it deteriorated to complete facial palsy in 1 patient. In 1 patient, radiation damage to the brainstem caused imbalance .
The experience with LINAC stereotactic RS at Cleveland Clinic Foundation was described by Suh and colleagues . Twenty-nine patients were treated in this study, with 4 suffering from NF2 and 12 having been operated on previously for ANs. The tumor volume ranged from 0.18 to 28.7 mL (median 2.1 mL). Tumors were targeted using a single isocenter in 24 cases and two isocenters in 5 cases. The treatment protocol called for a dose to the periphery that ranged from 8 to 24 Gy (median 16 Gy). Out of concern for possible damage to the brainstem, lower doses were used at first, ranging from 8 to 12 Gy. A 50% to 80% isodose line (median 80%) was used.
Patients were followed for a period of 4 to 110 months (median 49 months), during which they were seen 6 to 8 weeks after the procedure and then at 6-month intervals. The result of the treatment was local control in 28 of 29 patients defined as no increase in tumor size. Decrease in tumor size was achieved in 11 patients, and no size change was seen in 17 patients. This finding translated into an actuarial 5-year control rate of 94%. One tumor grew 41 months after the treatment.
The incidence of hearing complications was 74%, with 7 patients having a reduced level of hearing and another 7 becoming deaf. Because no audiograms were performed, the hearing data are subjective. Acute complications were noted in 2 patients and involved nausea and vomiting soon after the procedure. More serious complications included new or worsening facial numbness in 5 patients, 4 of whom experienced persistent facial numbness. Transient ataxia was noted in 3 patients (10%). New or worsening facial nerve deficit was observed in a total of 8 patients (28%) and was permanent in 7. In 1 patient, surgery to correct difficulty in closing the eye was performed. The 5-year actuarial incidence rates of trigeminal and facial neuropathy were calculated at 15% and 32%, respectively. Hydrocephalus was a problem in 2 patients, both of whom had shunts inserted. Brain stem edema on T2 images was noted on 1 patient’s MRI scan and was associated with balance problems.
Statistical analysis of the results of this study did not show any association between history of previous surgery, age, sex, history of neuropathy, NF2, maximal tumor diameter, or tumor volume and the risks for long-term complications .
Foote and colleagues described 149 patients treated with RS and analyzed the risk factors associated with this procedure. In this large study population of patients with AN, 8 patients (5.4%) had bilateral tumors associated with NF2 and 42 patients (28%) had undergone previous resection of their tumors. The mean tumor volume was 4.8 mL (range 0.3–22 mL). The number of isocenters used ranged from 1 to 11, increasing over the duration of the study, with 2 being the average. The doses of radiation administered ranged from 10 to 22.5 Gy, including only doses at increments of 2.5. A mean dose of 14 Gy was usually given to the 70% or 80% isodose line.
The results reported were based on the radiologic follow-up of 6 to 94 months (median 34 months). Control of tumor growth occurred in 93%, with 78 tumors decreasing in size and 45 tumors remaining stable. Ten lesions enlarged, giving the overall control rate at 5 years of 87% (95% CI, 76–98). Six of the patients participating in the study had to undergo surgery to excise the tumor.
In terms of complications, the risks of facial and trigeminal neuropathies 2 years after treatment were 11.8% (95% CI, 6.2–17.1) and 9.5% (95% CI, 4.5–14.3), respectively. Most of the patients who suffered these complications had transient symptoms.
The incidence of facial and trigeminal neuropathies decreased as the study continued. Of the 108 patients who were treated after January 1994, 5 patients suffered facial and 2 suffered trigeminal neuropathies. The actuarial 2-year incidence of facial neuropathy for the first 41 patients was 29%, whereas for the last 108 patients the incidence was reduced to 5%. Likewise, the risk of trigeminal neuropathy decreased from 29% to 2%. The authors suggested that this rate was representative of imaging and radiation dose planning and dose selection improvement.
Certain risk factors were shown to increase the incidence of complications. Radiation doses to the brainstem of >17.5 Gy increased the risk of facial neuropathy by 45-fold when compared with the doses <17.5 Gy. When comparing the 2-year incidence of any cranial neuropathy at doses of ≤12.5 Gy to doses above this value, the difference was marked at 2% and 24%, respectively. The authors also noted that having surgery before receiving radiation increased a patient’s risk of developing delayed cranial neuropathy by fivefold .
In another study on LINAC single fraction RS for AN, Spiegelmann and colleagues described 48 patients, 44 of whom were followed for 12 to 60 months (mean 32 months). Seven patients underwent prior surgery for ANs. The patients presented with maximum tumor diameters ranging from 10 to 31 mm (mean diameter 20 mm). In 40 cases the tumors were irradiated at one isocenter, 3 cases used two isocenters, and 1 case used three isocenters. Tumor margins were irradiated with an average of 14.55 Gy (range 11–20 Gy). Radiation doses ranging from 15 to 20 Gy were used for the first 2 years of the study. Later, tumors considered small and measuring <16 mm in diameter received a maximum dose of 14 Gy, whereas tumors that exceeded this size were treated with a minimum of 11 Gy at 68% to 90% isodose. This treatment was given over 20 to 45 minutes.
Based on the MRI scans obtained every 6 months earlier in the study and yearly during the last 2 years of the study, 98% of tumors were controlled. During the first year of follow-up, 11 tumors increased in size, and enlargement was combined with facial neuropathy in 8 patients. All tumors returned to smaller size at a later time. In 33 patients, tumor reduction was noted. The magnitude of shrinkage ranged from 15% to 90% and occurred at least 12 months after RS was administered. Most shrinkage occurred between 24 and 36 months. Ten tumors (23%) remained unchanged in terms of size, and 1 tumor enlarged at 48 months.
Serviceable hearing, defined as speech discrimination score of 70% or higher, was preserved in 71%. One improvement of speech discrimination score from 50% to 80% 24 months after therapy was noted.
Complications of hydrocephalus did not occur in this study. Eighteen percent of patients developed a new trigeminal neuropathy that was concomitant with facial neuropathy in all cases. In total, nine cases (24%) of facial neuropathy were reported, all of which occurred within 1 year of follow-up and experienced improvement over time. Facial weakness persisted in 3 patients. The rate of facial neuropathy, defined with the House-Brackmann scale, was 8%. The authors also noted that this rate depended on the dose of radiation administered: 5.5% of patients who received the dose of 14 Gy developed facial neuropathy, whereas patients treated with 15 to 20 Gy had a 42% incidence of neuropathy. Tumor size also had an effect. The risk of facial neuropathy was 9.5% and 54% in patients with small (0.8–3.7 mL) and large (4–11 mL) tumors, respectively. Conversely, 2 patients had improved facial nerve function after RS .
In the 2003 publication by Meijer and colleagues , single fraction RS and fractionated radiotherapy outcomes in treatment of ANs were compared. The two groups were not different with respect to tumor size (mean 2.5 cm); however, patients who received single treatment tended to be older (mean age 63 years) compared with a mean age of 43 years for the fractionated treatment group. This section describes the single fraction RS experience. Forty-nine patients were selected for the treatment with the single fraction of radiation based on their lack of teeth. The first 7 patients received a regimen of 10 Gy at 80% isodose line; the other 42 patients were treated with 12.5 Gy at 80%, with all treatments using a monoisocenter. The difference in outcomes between the patients receiving two different doses of radiation was not statistically significant. The mean follow-up for these patients was 30 months of yearly MRI scans, with tumor control defined as no increase of the diameter by 2 mm or more. The actuarial 5-year tumor control rate for RS patients was 100%.
Hearing was preserved in 75% of patients, with the data based on subjective patient reporting of perceived hearing level. The 5-year rate of facial nerve preservation was 93%, with 45 patients retaining normal function, whereas the preservation probability for the trigeminal nerve was 92%.
One patient who had undergone RS treatment developed hydrocephalus and was required to undergo an operation .
Two groups of patients, one treated with FSRT and the other with RS, were reported by Chung and colleagues in an article entitled “Audiologic and Treatment Outcomes After LINAC-based Stereotactic Irradiation for AN.” The two groups were analyzed independently, and outcomes of RS are described herein. RS was administered to 45 patients. In that group, 10 patients had prior surgery and 1 had ventriculoperitoneal (VP) shunt placed for a pre-existing hydrocephalus. The tumor diameter ranged from 4 to 34 mm (median 20 mm). All tumors were treated with a monoisocenter, with the exception of three tumors, which were treated with two isocenters. Forty-four of 45 patients received a dose of 12 Gy at 50% (3 patients) and 80% (41 patients) isodose line. One patient was treated with 15 Gy to the 80% isodose line, which produced a tumor control rate of 100% during the follow-up period of 8 to 61 months (median 27 months).
Among the complications reported, 2 patients (4.4%) developed hydrocephalus for which VP shunts were placed. One 92-year-old patient died from this complication. Permanent mild facial numbness developed in 3 patients (7.5%), and 2 patients (4.4%) had permanent House-Brackmann grade 2 facial paresis. One patient developed an infection at the pin site of the stereotactic headring. The pons (1 patient) and the cerebellum (1 patient) became edematous because of a vasogenic process, which was mild and responded to the administration of steroids. Tumor swelling, with nonenhancing necrotic core and concurrent swelling of the tumor, caused the compression of the brainstem in 1 patient and resulted in ataxia, ipsilateral numbness at the maxillary distribution, and diplopia .
A 2005 study by Okunaga and colleagues reported on 46 patients with unilateral ANs who were treated with LINAC RS. Of these patients, 12 (26.1%) had previously undergone resection. The tumor volume in this patient population ranged from 0.4 to 7.01 mL (median 2.29 mL) and they were targeted with one to four isocenters (median of two). Tumor margins were targeted with the median radiation dose of 14 Gy (range 10–16 Gy), and median maximal dose was 23.2 Gy (range 17–36.1 Gy). Radiation to the brainstem was limited to 10 Gy. During the 12 to 120 months of follow-up (median 56.5) with MRI studies performed every 3 to 4 months, eight tumors (19%) showed enlargement. This result was persistent and continuous in 3 patients (2 of whom had to repeat RS at 29 and 36 months), whereas in 5 patients, initial enlargement reached a plateau. No change was noted in two lesions (4.8%), whereas transient enlargement followed by shrinkage occurred in 19 patients (45.2%). Another 13 lesions (31%) shrunk directly with the minimum reduction ratio of 0.05-fold. Two tumors regrew approximately 5 years after RS.
Tumor control was achieved in 31 (73.8%) of 42 patients followed for more than 1 year, 31 (81.6%) of 38 patients followed for more than 2 years, and 18 (100%) of 18 patients followed for more than 5 years. In 11 patients, tumor size decreased more than 7 years after RS; in 5 patients tumor size decreased more than 9 years after RS. Central enhancement was lost in 37 (88.1%) of 42 tumors monitored longer than 1 year.
With respect to hearing preservation, useful hearing was maintained at Gardner-Robertson classes I or II in 66.7% of patients.
Among the procedural complications reported, 4 (7.5%) of 53 patients had hydrocephalus noted on initial visit and were treated with VP shunts and RS. Nine (21.4%) of the 42 patients monitored for longer than 1 year had ventricular enlargement. Of these patients, 3 (7.1%) required placement of a VP shunts and 4 (9.5%) improved spontaneously within 1 year of the finding. The study noted that 4.8% of patients developed new facial palsy, and new trigeminal neuropathy developed in 1 patient (2.4%) .
The most recently published data on the use of LINAC for treatment of ANs was found in the 2006 update of the RS experience at the Royal Adelaide Hospital. Roos and colleagues reported on 65 patients with AN who underwent RS, 61 of whom had sporadic and unilateral tumors and 4 of whom had tumors associated with NF2. Six patients had prior surgeries for the tumors, which have since recurred. The median tumor diameter was 22 mm (range 11–40 mm). Radiation was given in a single dose of 12 to 14 Gy to one or two isocenters. The patients were followed with annual MRI scans and audiometry for the first 2 to 3 years and once every 2 years afterwards. The median follow-up for this study group was 48 months (range 12–134 months).
A transient enlargement and central necrosis was noted in tumors of 9 patients (16%). The median increase in size was 4 mm (range 2–5 mm) and occurred from 4 to 25 months after the treatment (median 12 months), typically persisting for 1 to 2 years. Tumor control, which was defined as a reduction or stabilization of tumor size, was achieved in 59 of the 62 patients (95%). Seventeen tumors were stable over 12 to 85 months, and 42 shrank by 2 to 12 mm (median 4 mm) over 13 to 134 months. Of the patients treated with primary RS, 98.5% did not require surgical resection of the disease, and only 1 patient required an operation.
Loss of objectively useful hearing was noted in 18 of the 34 patients with assessable, useful pretreatment levels. The loss occurred by 8 to 77 months (median 24 months). Sixteen patients (47%) maintained a useful level of hearing over 20 to 108 months (median 60 months) of follow-up. Other complications of the procedure included partial trigeminal neuropathies in 7 patients, 2 of whom also developed facial neuropathies. Of these complications, 4 were new findings, whereas the other 3 patients had pre-existing numbness. Three cases of hydrocephalus necessitating a placement of VP shunts were reported .
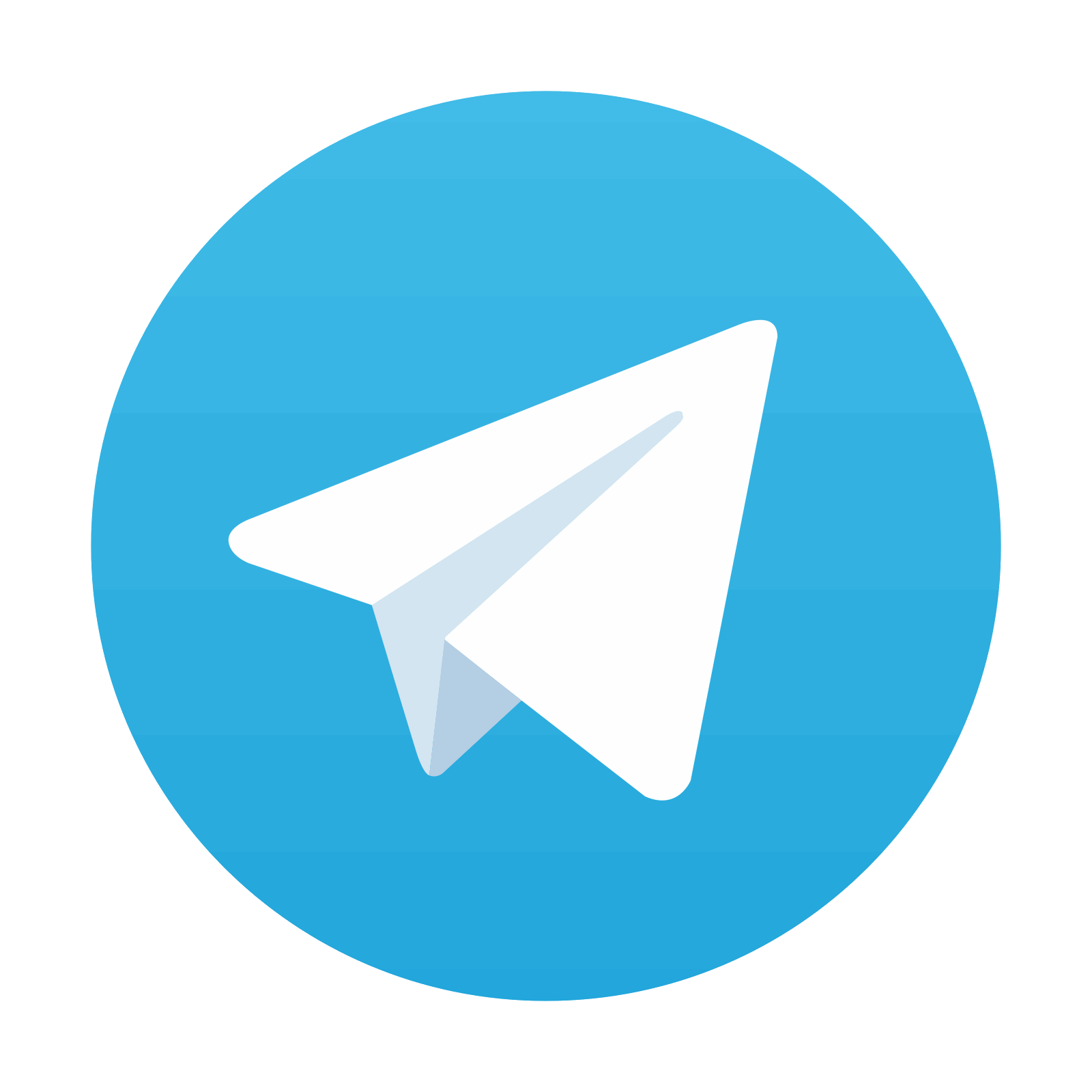
Stay updated, free articles. Join our Telegram channel

Full access? Get Clinical Tree
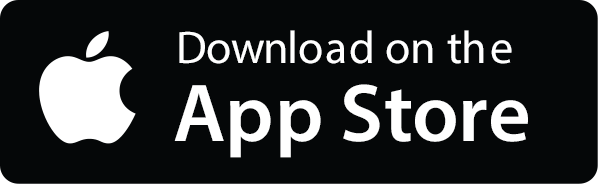
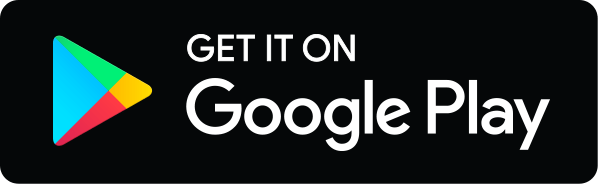