Lasers in Facial Plastic Surgery
William Russell Ries
Joseph E. Hall
“The Quantum Theory of Radiation,” published by Einstein in the early 1900s, established laser theory. Maiman built the first laser in 1960, and patient treatment began shortly thereafter (1). The first cutaneous laser treatment with ruby and neodymium pulsed laser systems was performed by Goldman, and Patel subsequently developed the first CO2 laser in 1964 (2). Lasers have become an integral treatment option for multiple disease processes, and clinical applications for lasers are ever expanding with the concurrent development of imaging systems, computer technology, and robotics (3). Lasers have become increasingly easy to use, and the fundamentals of laser physics are taught to a myriad of health care providers.
In this chapter, laser biophysics, laser-tissue interactions, technical aspects of laser treatment, and complications associated with lasers in facial plastic and reconstructive surgery are discussed. It is the hope of the authors that this chapter will provide a detailed understanding of laser technology, common laser applications, indications and reasons for employment of specific lasers, and practical knowledge related to the technical aspects of laser treatment.
LASER BIOPHYSICS
LASERs (light amplification by the stimulated emission of radiation) produce light energy that travels in a waveform. The distance between two successive peaks of the wave corresponds to the wavelength, while the height of the peak of the wave corresponds to the amplitude. The amplitude is related to the intensity of the light. The period, or the time for the completion of a full wave cycle, is referred to as the frequency (4).
To produce this waveform, lasers follow the fundamentals of quantum mechanics. Specifically, a power source provides energy to a system. Subsequently, photons, units of light energy, present in the system strike atoms in the laser medium and can raise one of its electrons to a higher energy level. Either spontaneous or stimulated emission can occur at this point. Spontaneous emission occurs when the atom in the excited and unstable state reemits a photon and the electron returns to the lower energy level. If an atom in the higher energy state is struck by an additional photon, two photons are emitted. This process is termed stimulated emission (Fig. 194.1). The photons produced will continue to propagate the energy transfer via mirrors within the system. The photons emitted characteristically have the same wavelength, progress in the same direction, and travel in phase (3).
Lasers consist of four main components including a power source, a laser medium, an optical cavity or resonator, and a delivery system (Fig. 194.2). Electrical, light, and high-energy radio frequency waves are examples of possible excitation mechanisms. The optical cavity or resonator contains a medium that may be solid, liquid, gas, or a semiconductive material. Lasers also have a mirror that is 100% reflective and a mirror that is partially transmissive to allow energy to escape the chamber. Delivery systems are variable with most utilizing a fiberoptic delivery system but some using an articulated arm system (CO2 laser).
The components of the laser together produce light that is of one wavelength (monochromatic), with little divergence (collimated), and in identical temporal and spatial phases (coherent). These properties differentiate laser light from ordinary light.
LASER-TISSUE INTERACTION
Laser energy can have a variety of effects on tissue ranging from activation of biochemical substances or stimulation of biologic tissues at low energy to coagulation or vaporization of biologic tissues at high energy (Fig. 194.3) (3). Most clinically relevant lasers utilize the thermal effects of lasers to coagulate or vaporize. The effect of a laser on a given
tissue is a function of the laser wavelength; laser energy density; and the amount of reflection, scatter, absorption, or transmission of energy that occurs (Fig. 194.4) (3).
tissue is a function of the laser wavelength; laser energy density; and the amount of reflection, scatter, absorption, or transmission of energy that occurs (Fig. 194.4) (3).
Chromophores are substances that absorb energy at specific wavelengths. The specific absorption patterns of tissues partly determine the most efficacious laser for a given lesion. The chromophore absorbs light energy, which is then converted to heat. Coagulation of tissues occurs at 60°C to 70°C, and tissue vaporization occurs at 100°C (5). Appropriate laser selection allows for targeted thermal damage in tissues that absorb a specific wavelength of light. This process is termed selective photothermolysis (5). For example, the soft tissues of the body, which consist of mainly of water molecules, absorb CO2 laser energy efficiently, whereas bone, with little water content, does not. Another important laser property is wavelength, with deeper tissue penetration occurring with longer wavelengths of light (5). The peak absorption wavelength for oxyhemoglobin, which is found in high concentrations in vascular malformations and hemangiomas, is 577 to 585 nm.
Two important laser parameters are energy density and power density. Energy density (fluence) is a function of the power density (intensity) of the laser and the time of exposure. Power density is a function of the power divided by the cross-sectional area of the laser beam (spot size). Thus, by altering power density or time of exposure, the amount of energy delivered by a particular laser can be altered. Additionally, by decreasing the laser spot size, power density is increased.
Energy density (fluence) = Power density × Time
Power density = Power (watts)/Cross-sectional area of laser beam (spot size)
Based on these equations, one can readily deduce that by decreasing the spot size of the laser beam, the power density to the tissue of interest increases. Pulsed energy can also be utilized to alter tissue effects. The pulsed energy mechanism incorporates regular periods of irradiation (on period) with alternating periods of no irradiation (off period). This alternating delivery of laser energy permits heat dissipation during the off period. Thus, thermal heat damage to surrounding tissue is minimized, particularly if the off period is longer than the thermal relaxation time of the tissue of interest. The thermal relaxation time is defined as the amount of time required for an object to dissipate half of its heat. The duty cycle is defined as the ratio of the on interval to the on plus off intervals.
Several pulsed modes have been created for use with lasers. Shuttering can block the delivery of a beam of laser light for specified intervals of time. Superpulse mode refers to a mechanism in which energy is stored during the off period and released during the on period. Q-switched lasers function by storing energy during the off period and releasing high-energy bursts of light for short time intervals using an electro-optical shutter (3). Ultimately, one
must match the laser wavelength, spot size, energy density, power, and duration of action to the tissue of interest and the desired outcome (5).
must match the laser wavelength, spot size, energy density, power, and duration of action to the tissue of interest and the desired outcome (5).
LASER CHARACTERISTICS
Flashlamp-Excited Dye Laser
The flashlamp-excited dye laser (FEDL) was designed for the treatment of vascular cutaneous lesions. The laser medium is a rhodamine dye, which is excited optically by a flashlamp. It is delivered by a fiberoptic carrier. This laser produces visible, yellow light (6). The FEDL system is frequently used to treat hemangiomas, port-wine stains, rosacea, and telangiectasias as it emits wavelengths of 585 nm, near the absorption peak of oxyhemoglobin (577 nm) (6,7). The FEDL has a dynamic cooling device that reduces the risk of epidermal injury.
The FEDL has an interchangeable lens system that allows for spot sizes of 3, 5, 7, or 10 mm. The pulse is 450 µs. More recently developed FEDLs can produce longer wavelengths (595 to 600 nm) with spot sizes of 10 to 12 mm and higher peak fluence potentials. These alterations have allowed for more effective treatment of the deeper vessels found in hemangiomas and port-wine stains (6,8). Additionally, longer pulse durations of 1.5 to 40 ms have allowed for telangiectasia treatment without the development of significant purpura (6,8,9,10,11). The alterations to the FEDL have provided a treatment that has been shown to reduce erythema, decrease height, and improve texture in scars (12). A decreased rate of recurrence of hypertrophic scars has also been found with the FEDL (13).
Pulsed dye lasers (PDLs) have recently incorporated a unique pulse structure where each macropulse is subdivided into eight micropulses. This technology allows for single-pass treatment with delivery of higher total fluencies with less risk of purpura (14,15). Most patients develop variable erythema and/or purpura following PDL treatments, which may last for 7 to 10 days. Furthermore, patients may develop hyperpigmentation, hypopigmentation, or scarring (6).
Intense Pulsed Light
Intense pulsed light (IPL) uses crystal filters to emit light with wavelengths of 590 to 1,200 nm. The light is noncoherent. The range of wavelengths and pulse durations capable of being produced by this laser are its primary advantages. Cutoff filters produce wavelengths that can be utilized for telangiectasias, skin discoloration, hair removal, or photoaging. Likewise, IPL system filters can eliminate shorter wavelengths to allow for greater dermal penetration with light of longer wavelength (6). IPL systems have also been used to treat port-wine stains and hemangiomas (6). In comparison with the PDL, IPL treatments require more time and have greater associated risks as additive heating occurs with successive passes and longer pulse durations (6).
The primary use for IPL systems is treatment of telangiectasias. Selected wavelengths between 470 and 1,400 nm corresponding to absorption peaks of oxyhemoglobin are used to specifically target vessels. The range of wavelengths allows for treatment of vessels at various depths and greater energy penetration into the tissue (6). Newer systems incorporate cooling with chilled sapphire windows.
Potassium Titanyl Phosphate Laser
The potassium titanyl phosphate (KTP) laser is a frequencydoubled (wavelength halved) Nd:YAG laser. This is accomplished by passing the laser energy though a KTP crystal. The resultant light is green with a wavelength of 532 nm, corresponding to a hemoglobin absorption peak. The penetration and scattering of this laser light is intermediate between the CO2 laser and the Nd:YAG laser. The laser energy is delivered to tissue via a fiber. If the fiber is not in contact with the tissue, vaporization and coagulation occur. Lower settings are typically used for coagulation. If the fiber is in semicontact with the tissue, cutting occurs. The KTP laser has a wide range of pulse durations, from 1 to 100 ms. The KTP laser has a shallow penetration depth. Accordingly, there is low risk of purpura, edema, or crusting (6,16). Atrophic scarring is a concern with the KTP laser.
Red and Infrared Lasers
The alexandrite (755 nm), diode (800 to 940 nm), and Nd:YAG (1,064 nm) lasers are in the red and near-infrared (IR) range. These lasers have been used to treat reticular veins, port-wine stains, and deep vessels in vascular malformations. Red and IR lasers are effective for these lesions as they have wavelengths that target peaks of deoxyhemoglobin in the near-IR range (700 to 1,200 nm), achieve deeper penetration, and allow for higher fluence (6).
The alexandrite laser is a flashlamp-pumped solid-state laser in the red spectrum of light (755 nm) but is not visible. This laser is absorbed by melanin and achieves deep penetration. The alexandrite laser has been used with significant improvement in hypertrophic port-wine stains with settings of 3-mm spot size, fluence range from 30 to 85 J/cm2, and dynamic cooling (17). Others use settings of 8-mm spot size for the initial treatment of deep malformations (6). Li et al. (18) also demonstrated the effectiveness of the long-pulse pulsed alexandrite laser for hypertrophic, purple port-wine stains. The authors did note that this laser can cause dyspigmentation but no scarring was noted (18). This wavelength is also absorbed by the blue and black pigments in tattoos and can be used for hair removal.
The diode laser uses superconducting materials that are directly coupled to fiberoptic delivery devices. These lasers result in varying wavelengths depending on the material used. Diode lasers are efficient, converting electrical power to light at efficiencies of 50%, which allows for less heat production and power input (16). The light is fiberoptically delivered.
The Nd:YAG laser emits a wavelength of 1,064 nm, in the near-IR spectrum. The laser medium is neodymium:yttrium aluminum garnet and uses a helium-neon aiming beam. Pigmented tissue is selectively targeted with this laser as it penetrates up to 4 to 6 mm for treatment of deeper vessels (19). The depth of penetration of the Nd:YAG is related to its scatter, which is much greater than with the CO2 laser. Thus, the Nd:YAG laser can be used for deep vessel coagulation (˜3 mm). Yang et al. found the long-pulsed Nd:YAG laser to be equally effective as the PDL for the treatment of port-wine stains in a series of 17 patients (20). The authors found that purpura lasted longer following PDL treatments, but the Nd:YAG caused greater perivascular and epidermal injury with scarring noted in one patient (20).
Depressed scarring and hyperpigmentation are potential complications associated with the Nd:YAG laser (6). Cooling devices assist in minimizing these risks. In comparison with the near-IR diode laser, the Nd:YAG laser has deeper penetration, incurs less surrounding epidermal injury, and may be used more safely in darker pigmented individuals (6).
Carbon Dioxide (CO2) Laser
The CO2 laser uses CO2 gas as a medium and has a wavelength of 10,600 nm. This wavelength is found in the mid-IR range and is absorbed by water. This laser is utilized for tissue with high water content given that it is absorbed well by water molecules. Effects are typically superficial due to minimal scatter and high absorption rates. This laser seals vessels up to 0.5 mm in diameter and nerve fibers.
A helium-neon aiming beam is utilized with this laser system as the laser beam is invisible. Typically, the beam is delivered to tissue through mirrors and lenses in an articulated arm either by a handpiece or attached to an operating microscope (4).
Recently, the fractionated CO2 laser has gained popularity as it provides several distinct advantages over traditional CO2 laser treatments. The fractionated CO2 laser employs the concept of fractionated photothermolysis, which spares the epidermis from significant injury while causing coagulative injury in the dermis (21). Injury to the surrounding tissue is minimized as the fractionated laser delivers microthermal zones that allow for rapid reepithelialization at the periphery. The fractionated CO2 laser decreases scarring, hypopigmentation, and the recovery time of traditional CO2 laser treatments (21,22). The fractionated CO2
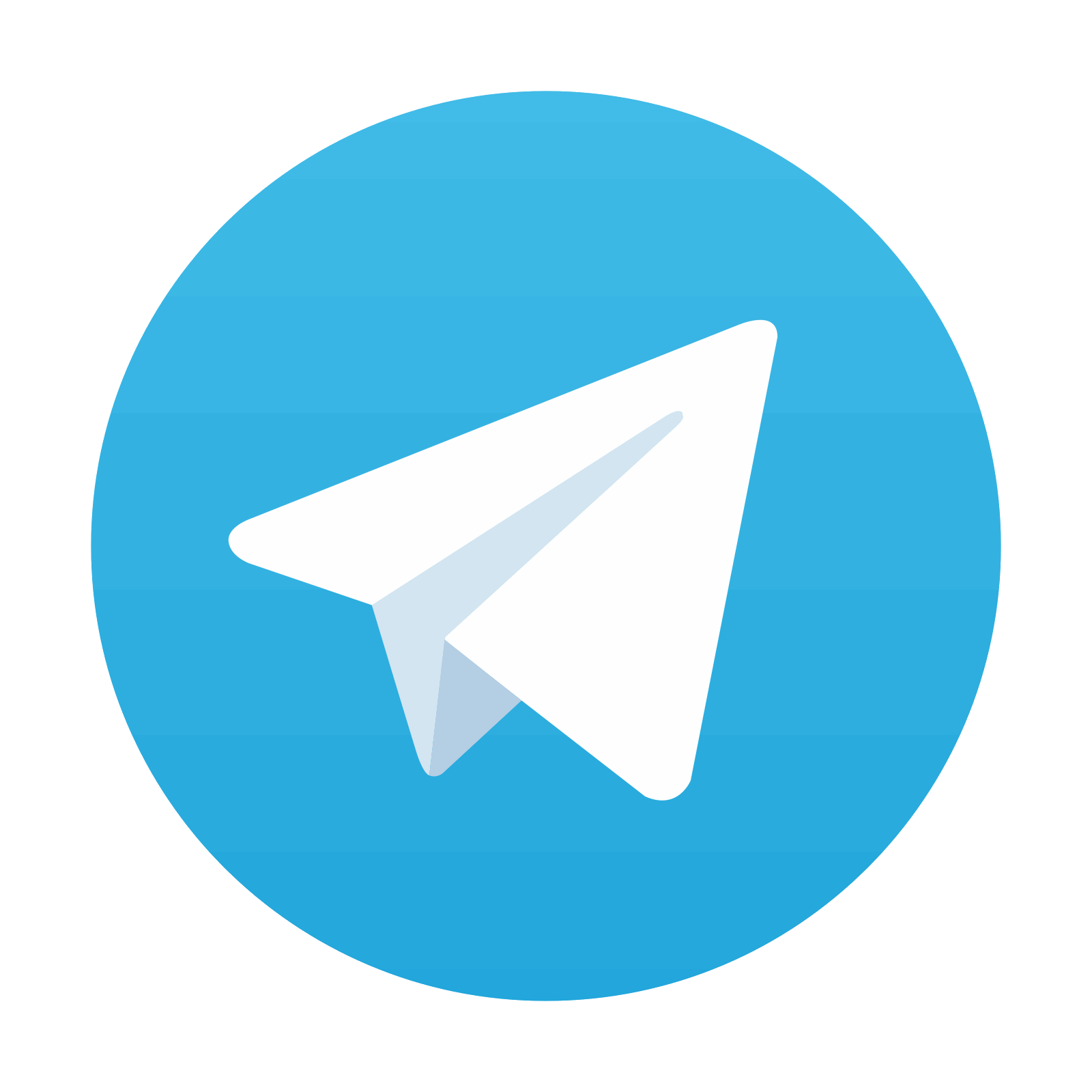
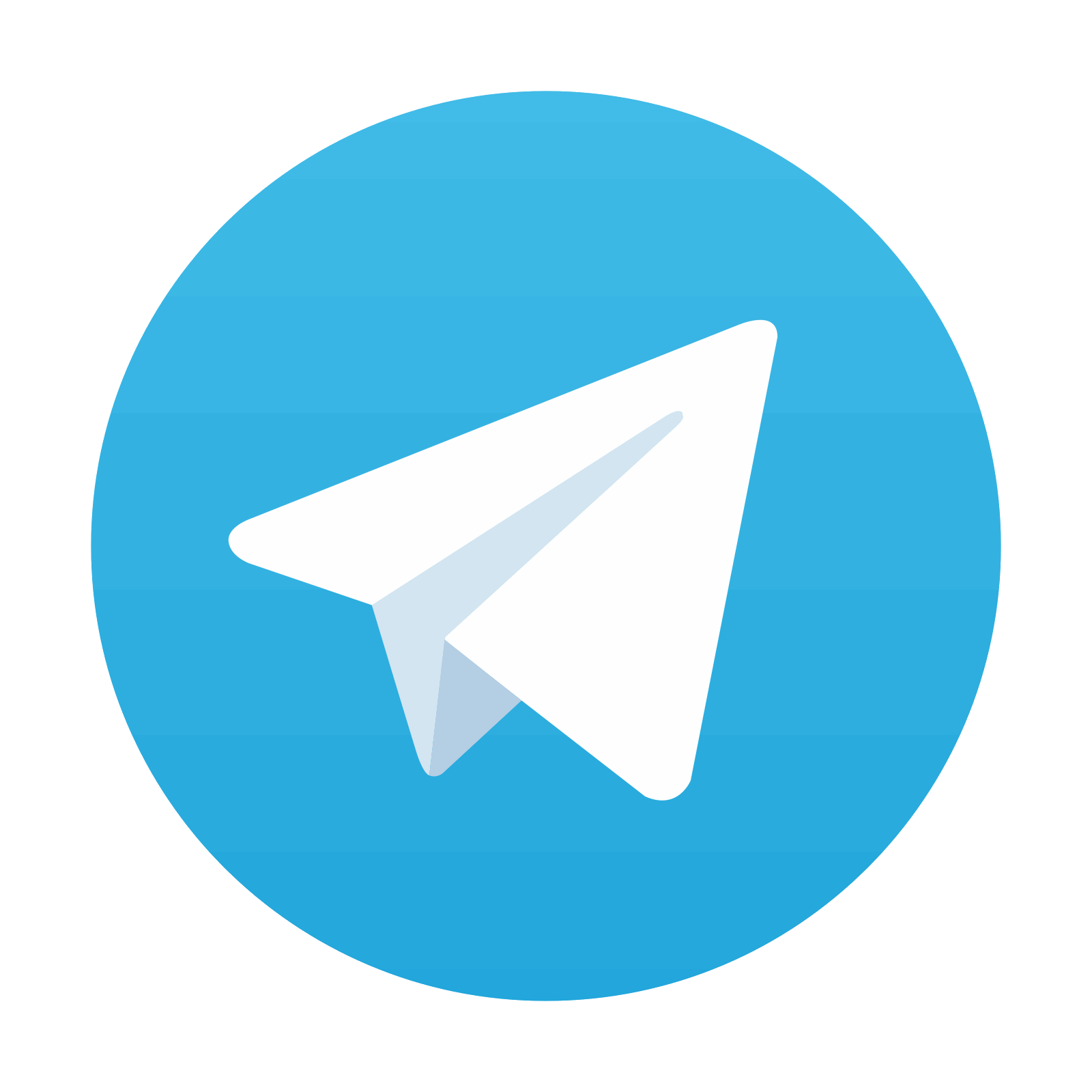
Stay updated, free articles. Join our Telegram channel

Full access? Get Clinical Tree
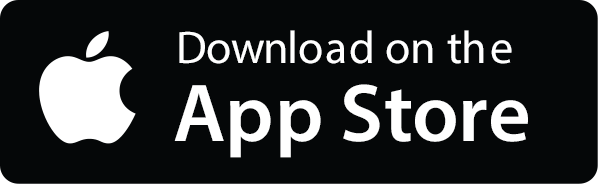
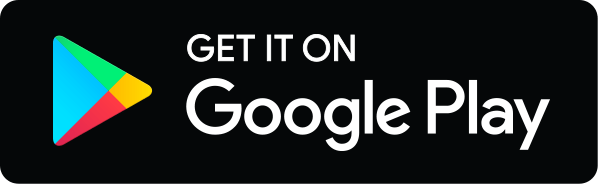
