This chapter covers a number of pathologic conditions that affect auditory function due to their effects on the inner ear. A brief overview of inner ear anatomy will orient the reader of auditory sciences to the specific site of dysfunction, as well as to the treatment of these conditions. Recall from Chapter 2, “Structure and Function of the Auditory and Vestibular Systems,” that the cochlea is a sensory auditory organ encased in the dense bone of the petrous portion of the temporal bone. The bone surrounding the cochlea is formed via an endochondral ossification process and is referred to as the otic capsule. This bone is extremely dense compared with the surrounding temporal bone; however, it has poor reparative capacity. The cochlea is a bony canal that spirals 2.5 times around the modiolus, which contains the spiral ganglion and myelinated and unmyelinated cochlear nerve fibers. This canal contains three distinct fluid-filled channels: the scala media, the scala tympani, and the scala vestibuli. The perilymph-filled flanking channels, which include the scala tympani and the scala vestibuli, are confluent at the cochlear apex, the helicotrema. The central channel, referred to as the scala media or cochlear duct, is filled with endolymph, a fluid that is secreted by the stria vascularis. This channel contains the acoustic sensory apparatus called the organ of Corti. The organ of Corti rests on the basilar membrane, which separates the scala media and scala tympani. This structure is lined with one row of inner hair cells and three rows of outer hair cells along the entire length of the cochlea. A second membrane, Reissner’s membrane, separates the scala media from the scala vestibuli and facilitates the translation of a cochlear fluid wave between the two compartments.
Sound waves within the external auditory canal are converted to vibratory mechanical energy by the tympanic membrane and the ossicles. This mechanical energy is conducted by the stapes into the scala vestibuli. A fluid wave is then propagated within the cochlea, which travels from the base to the apex. The fluid wave leads to deflection of the hair cells’ stereocilia, which results in depolarization of the hair cells and neural transmission via the cochlear portion of the eighth cranial nerve. The cochlea is tonotopically organized so that the fluid wave within the cochlea transduces high-frequency acoustic information at the cochlea’s base and low-frequency information at the apex.
The otic capsule bone also encases the vestibular apparatus of the inner ear, which is composed of the utricle, the saccule, and the semicircular canals. These structures are filled with endolymph and are confluent with the scala media of the cochlea. Disease processes that affect the cochlea may also affect the vestibular system due to the close anatomic relationship of these structures and the confluence of endolymph, which may elicit vestibular symptoms along with auditory deficits. A wide variety of diseases, both acquired and congenital, can affect the inner ear and result in significant hearing and/or vestibular dysfunction.
There are numerous disorders that can affect the normal functioning of the inner ear and can result in a variety of otologic/audiologic symptoms. The discussion that follows is not intended to be an exhaustive coverage of the otologic disorders that may result in inner ear impairment. Rather, it is intended to highlight the more commonly encountered disorders that may affect the cochlea and result in auditory dysfunction.
The otic capsule is extremely dense and relatively resistant to fracture; however, injury to the cochlea may be caused by blunt or penetrating trauma. Blunt trauma is typically a result of automobile accidents, recreational vehicle accidents, falls, or assaults. Penetrating injuries are commonly related to gunshot injuries or stabbings and can carry a high mortality rate. Sudden changes in pressure within the external or middle ear can also lead to traumatic perilymph fistulas as discussed later in this chapter. This section of the chapter deals with direct and indirect trauma to the otic capsule structures and the resulting effects on auditory function.
Temporal bone injury typically is a small part of a multisystem trauma that often affects patients. Patients with temporal bone trauma frequently suffer concomitant intracranial injuries with severe mental status changes and neurologic deficits, which may delay accurate audiologic assessment. Violation of the otic capsule bone results in sensorineural hearing loss, which usually is profound and irreversible. Vertigo, which often accompanies hearing loss, may be severe in the acute stages and may require weeks to months to abate. Bone fragmentation also may lead to facial nerve injury or even transection, and facial weakness may present in a delayed fashion due to hematoma or edema of the facial nerve. The external auditory canal and the tympanic cavity structures can also be damaged, but these sites of damage are not discussed in this chapter as they are outer and middle ear structures (see Chapter 4). Damage to major blood vessels that traverse the temporal bone may present with massive hemorrhage from the ear and represents a true emergency requiring immediate medical attention.
Automobile accidents are the leading cause of head trauma, representing up to 75% of all cases of temporal bone fractures. Other common causes include assaults, falls, motorcycle accidents, bicycle accidents, and gunshot wounds. Temporal bone fractures resulting from these types of traumatic events are more common in men than in women, with a peak incidence in the third and fourth decades of life (Saraiya & Aygun, 2009).
A temporal bone fracture is the result of significant force to the skull. Fractures that are oriented longitudinally to the long axis of the petrous temporal bone are due to blunt force to the lateral skull and are more prevalent than other types of fractures, accounting for 70% to 80% of all temporal bone fractures. Fractures transverse to the petrous bone are less common, but facial nerve injury occurs approximately 50% of the time in this type of fracture. Often, fractures involve both longitudinal and transverse fracture lines. Penetrating trauma results in comminuted fractures and classic fracture patterns are typically not seen. The location of the fracture line in relationship to the otic capsule is clinically significant (Dahiya et al., 1999).
An otic capsule sparing fracture is similar to the longitudinal fracture line discussed previously, and the cochlea and vestibule typically remain intact. An otic capsule violating fracture has a fracture line extending into the cochlea or vestibule and is associated with a significantly higher incidence of hearing loss and/or vertigo. This latter type of fracture line typically disrupts the delicate membranous labyrinth and results in profound, irreversible sensorineural hearing loss.
Fracture lines may also cause avulsion and separation of the spiral ganglion acoustic nerve fibers at the fundus of the internal auditory canal. In addition, blunt trauma to the temporal bone may open abnormal fistulae of the labyrinth in the area of the oval window or the round window, resulting in what is referred to as a perilymph fistula. Hearing losses without discrete fracture lines can also be seen after blunt trauma due to concussive trauma; however, the exact mechanism for this type of insult is poorly understood.
As mentioned previously, fractures of the temporal bone adjacent to the otic capsule may involve injury to the facial nerve and an abnormal fistula within the ear may occur. In addition, a major vascular injury to the sigmoid sinus, jugular bulb, or carotid artery can occur as a result of penetrating trauma or impinging bony fragments from blunt trauma.
Audiologic testing of the patient who has sustained head trauma is performed following stabilization of the patient. Many approaches can be taken with respect to audiologic evaluation, but at a minimum, comprehensive pure-tone and speech recognition testing along with tympanometry should be performed. Eventually, it may prove useful to determine both peripheral (cochlear and vestibular nerve) and central hearing status in these patients. Prior to initiating any audiologic testing, otoscopy should be performed as the external auditory meatus may need to be cleaned if fluid and/or blood has accumulated in the canal. Clinicians must take extra caution when performing an audiologic evaluation on a patient with temporal bone trauma as these patients often are in an altered state, they may be uncooperative, and they may be experiencing significant pain. In addition, in some cases it will be difficult, if not impossible, to perform bone-conduction testing due to the presence of the fracture.
Results from the audiologic evaluation may present as a conductive hearing loss, a sensorineural hearing loss, or as a mixed hearing loss with both conductive and sensorineural components. The type of loss often is correlated with the fracture characteristic. Fractures that are longitudinal in nature often result in more conductive involvement as those fractures run through the petrous pyramid and may extend as far as the mastoid bone, whereas sensorineural hearing loss tends to be more common in cases with transverse fractures (Jerger & Jerger, 1981).
A large-scale study by Brodie and Thompson (1997) examined complications from temporal bone fractures. They found that 7% of temporal bone fractures resulted in facial paralysis. Of those resulting in facial paralysis, 27% had an immediate onset of the paralysis, while 73% had a delayed onset. If a patient presents with facial paralysis, electroneurography (ENoG) would be an appropriate test to administer (i.e., if this procedure is done in the audiology/otolaryngology clinic; if not, referral to the department that performs this testing is recommended) so that a determination of the extent of facial nerve involvement can be made. This becomes a critical tool in determining if surgical intervention to explore and decompress the nerve is needed. There is a high probability that auditory and vestibular nerve disruption may coexist with facial nerve injury. In these cases, additional audiologic and vestibular testing for potential auditory and/or vestibular nerve involvement would be indicated.
Trauma effects are rarely isolated to the temporal bone, and patients who present with temporal bone trauma typically experience compromise of other systems in addition to the auditory system. These patients frequently require stabilization and evaluation according to cardiopulmonary resuscitation protocols. Many may be intubated and mechanically ventilated due to their neurologic status and/or other injuries. This may delay an accurate and thorough examination for days; however, efforts should be taken to perform an initial assessment as close in time to the insult as is possible. This assessment should include examination of the pinna and ear canal. Periauricular bruising may indicate temporal bone fracture and extravasation of blood in subcutaneous tissue planes. Bony fragments, blood, and/or debris may hinder inspection of the ear canal. If the tympanic membrane can be visualized, one should inspect for a bluish bulge of the membrane, which results from blood filling the middle ear space, a condition referred to as a hemotympanum. Pneumatic otoscopy should also be performed as this can evaluate the integrity of the tympanic membrane and also test for a perilymph fistula. Positive and negative pressure within the external canal can elicit nystagmus and support the diagnosis of a fistula. Cerebral spinal fluid may be found leaking from the ear, which will appear as clear watery otorrhea, or it may leak from the nose (a condition referred to as rhinorrhea). A thorough cranial nerve exam as a part of the head and neck exam may reveal focal cranial neuropathies. Facial nerve function and the onset of facial weakness should be documented. Other injuries may postpone a thorough audiometric evaluation, thus a tuning fork evaluation (Weber and Rinne tests), which can provide information regarding the conductive and sensorineural function of the ear, should be included with every temporal bone trauma evaluation. Typically, radiographic imaging is performed via a high-resolution computed tomography (CT) of the temporal bone with thin slices. This type of scan quickly provides the information necessary to guide clinical decisions and predict outcomes. The fracture pattern can be identified and surrounding anatomy can be delineated with careful inspection of the CT scan (Saraiya & Aygun, 2009).
Recovery of auditory function typically ranges from 3 weeks to 6 months (Shulman, 1979). Due to the likelihood of fluctuations in hearing sensitivity, it is important to continually monitor hearing thresholds over time. Obtaining stable audiologic results is essential. If the patient presents with sustained hearing loss, appropriate audiologic management, including the fitting of hearing aids, would be warranted.
At times, the hearing loss may be so severe that traditional amplification will not be useful. A profoundly deafened ear may utilize a contralateral routing of signal (CROS) aid (with a microphone on the involved ear and the receiver on the contralateral ear). This assists in transmitting information from the involved ear to the uninvolved ear. In addition, osseointegrated devices have also been used in some cases of single-sided deafness. If bilateral sensorineural hearing loss is present, cochlear implantation may be considered (Morgan, Coker, & Jenkins, 1994); however, these patients represent a special challenge for cochlear implants due to the possibility of intracochlear fibrosis and the remote possibility that the cochlear nerve itself could have been injured medial to the cochlea.
Vertigo following temporal bone trauma should be evaluated by videonystagmography (VNG). Patients may have a concussive membranous labyrinth injury that presents with positional vertigo with an otherwise normal VNG. Traumatic cupulolithiasis can be managed by repositioning maneuvers. A VNG test administered with the insertion of positive and negative pressure within the ear canal via a tympanometer may also identify a positive fistula test and may support a middle ear exploration with closure of the fistula.
The role of medical and surgical intervention is to manage complications caused by temporal bone fractures. Some cases may only involve concussive injuries to the inner ear structures and these patients may regain function spontaneously over time. Injury to the otic capsule, however, is unfortunately permanent. Vertigo that may be present due to vestibular injury can be effectively suppressed with benzodiazepines and antiemetics. Compensation of the vestibular system typically occurs in patients with temporal bone fractures affecting the vestibular structures. This compensation usually evolves over the first few weeks and is expedited by physical activity and limiting CNS-depressing medications. There is currently no role for medical or surgical intervention for sensorineural hearing loss related to temporal bone trauma.
Case 5–1: Temporal Bone Trauma
History
A 33-year-old female was seen for a hearing evaluation due to complaints regarding hearing sensitivity, particularly in the presence of background noise, following a head injury. She was a horse trainer who was thrown from a horse and subsequently kicked in the head, resulting in a basilar skull fracture on the right side. Following her head injury, she experienced a general decrease in her hearing sensitivity. She also experienced constant tinnitus, which she described as being centralized in her head, and significant imbalance, particularly when riding horses. The patient additionally reported that she often found herself asking for repetition and relying heavily on visual cues to aid in comprehension of spoken messages. Prior to her accident, she noted that she had no difficulties with her hearing. Although she has consistently reported a loss of balance when riding horses since the time of her accident, her “balance” deficit was recently made very clear to her when exiting a boat and returning to land following a boat trip. During this event, she reported that she was unable to move due to fear of falling. She also reported deficits in short-term memory, which she attributed to her head trauma. No other significant audiologic or otologic history was reported at the time of this patient’s evaluation.
Audiology
An otoscopic check was unremarkable bilaterally. Tympanometry revealed normal pressure, volume, and compliance bilaterally (Figure 5–1A). Responses to pure-tone audiometry indicated normal peripheral hearing sensitivity for the left ear. Hearing sensitivity in the right ear was within normal limits through 1000 Hz and sloped to a mild sensorineural hearing loss for the remaining frequencies. Speech recognition thresholds were in good agreement with the pure-tone averages for both ears and word recognition ability was excellent bilaterally (see Figure 5–1A). Reliability was considered to be good. As the traditional audiologic evaluation did not fully explain the patient’s complaints, a central auditory processing evaluation was initiated.
The central auditory processing (CAP) evaluation revealed a right ear deficit in temporal resolution (abnormally large gap detection threshold) and a bilateral deficit for temporally degraded speech (Figure 5–1B). Although one cannot rule out peripheral involvement as a contributory factor to the abnormal performance noted on these central tests, it is unlikely given the presence of a “very” mild hearing loss in the high frequencies in only the right ear, the excellent word recognition scores noted bilaterally, and the finding of a “bilateral” deficit on the temporally degraded speech test. In addition, a combined auditory brainstem (ABR) and middle latency response (MLR) evaluation was completed to assess the physiologic status of the central auditory nervous system (CANS) from the brainstem to the level of primary auditory cortex (Figure 5–1C). Waveform morphology and repeatability were relatively good. The MLR testing revealed an abnormal asymmetry (ear effect) with a stronger right-ear response. This can be interpreted as an abnormal MLR, but it does not lateralize the problem.
Figure 5–1. Pure-tone thresholds, speech audiometry, and tympanometry results (A), behavioral central auditory tests results (B), and ABR–MLR tracings (C) for a 33-year-old female who sustained trauma to the temporal bone (Case 5–1).
A vestibular evaluation also was performed. Computerized dynamic posturography (CDP) was completed in order to further assess the balance and vestibular complaints reported by the patient. Results from the CDP indicated a vision-preference pattern. This pattern is often observed in patients who have experienced central insult resulting in balance problems (Black & Nashner, 1985). Patients with this type of pattern are more likely to demonstrate normal VNGs or only subtle deficits on VNG testing. In the current patient, the VNG testing demonstrated subtle abnormalities on ocular motor testing. This was likely the reason why this patient was having difficulty training and riding horses following her accident. This type of problem may be improved through the use of physical therapy designed to reduce the abnormal reliance on visual information.
Medical Examination
The patient presented with an essentially normal otolaryngologic examination.
Impression
Temporal bone fracture resulting in mild sensorineural hearing loss in the right ear with comorbid auditory processing and vestibular deficits secondary to head trauma.
Audiologic Recommendations and Management
It was recommended that the patient undergo both audiologic and vestibular rehabilitation. The aural rehabilitation procedures recommended included intense training exercises to build skills in the areas of temporal resolution and the processing of rapid and/or degraded speech. In addition, mild gain hearing aids were recommended in order to assist with both the peripheral hearing loss and auditory processing deficit.
Medical Recommendations and Management
None indicated.
Additional Comments
This case highlights the common finding that in cases of temporal bone trauma, deficits are not necessarily limited to the inner ear but that trauma often involves multiple systems. This patient’s injuries resulted in peripheral hearing loss, as well as central vestibular disturbances and retrocochlear auditory dysfunction.
Noise-induced hearing loss (NIHL) is a disorder that is ubiquitous and related to occupational or recreational noise exposure. Approximately 22 million U.S. workers are at risk for hearing loss due to significant occupational noise exposure (Tak, Davis, & Calvert, 2009). Of the 22 million, 34% report nonuse of personal hearing protection devices. The Occupational Safety and Health Administration (OSHA) of the U.S. Department of Labor has developed standards for noise exposure in the workplace in order to decrease NIHL (OSHA, 1983). Unfortunately, these same standards do not apply to most recreational activities such as sporting events and firearm use. These activities often result in NIHL that either can be caused by the recreational activities alone or can contribute to NIHL that is a function of both occupational and recreational noise exposure (Ivory, Cane, & Diaz, 2014). Sporting events alone have been found to yield peak levels of 105 dBA to 124 dBA (Cranston, Brazile, Sandfort, & Gotshall, 2013). Both the intensity and duration of noise exposure can have an impact on auditory function. Hearing loss related to noise exposure may be caused by exposure to an isolated sudden high-intensity acoustic signal and is classified as acoustic trauma, or to exposure to elevated noise levels over time causing a more gradual onset of hearing loss, which is commonly referred to as a threshold shift that can either be temporary or permanent (Daniel, 2007).
The Occupational Safety and Health Administration (1983) has provided regulations regarding occupational noise exposure and has determined that habitual exposure to high-noise levels can cause a gradual hearing loss. Thus, unprotected exposure to steady-state noise is limited to 8 hr per day at 90 dBA. However, exposure levels of 85 dBA for 8 hr can cause hearing loss, and it is at this level that the employer must institute a hearing conservation program including yearly audiograms and the provision of ear protection. No unprotected steady-state exposure above 115 dBA is permitted. Also, unprotected exposure to impulse noise levels above 140 dB SPL is not allowed by these regulations.
Patients with a history of NIHL frequently present with tinnitus, typically a high-pitched constant tinnitus that is nonpulsatile in nature and develops gradually over time (Eggermont & Roberts, 2004). Like any sensorineural hearing loss, NIHL may result in recruitment (the abnormal growth in loudness perception as intensity increases). The hearing loss in the majority of cases with NIHL is gradual in onset and typically involves primarily the high frequencies, often creating a “noise notch” around 4000 Hz. Patients exposed to high-intensity noise may experience a temporary threshold shift (TTS) in hearing that may last 12 to 36 hr. This shift can be significant and repeated exposures will likely lead to the loss of hair cells and neural fibers producing a permanent threshold shift (PTS). A sensation of aural fullness or pressure is often associated with NIHL. Vestibular symptoms, on the other hand, are notably absent in NIHL of gradual onset, but they may be present in cases of acoustic trauma.
Clinicians should be keenly aware of the rising trend in audiologic and otologic conditions associated with blast-related injuries. Most notably, there has been a significant increase in blast-related injuries among military personnel during deployments, which has resulted in both peripheral and central auditory and vestibular dysfunction (Fausti, Wilmington, Gallun, Meyers, & Henry, 2009; Gallun et al., 2012; Gallun, Papesh, & Lewis, 2017).
Noise-induced hearing loss is the second most common cause of sensorineural hearing loss. It is estimated that 10 million adults under the age of 70 are reported to have hearing loss related to excessive noise exposure (National Institute on Deafness and Other Communication Disorders, 2019). Nearly 24% of adults ages 20 to 69 who participated in a large survey study were found to have noise notches suggesting a high prevalence of NIHL (Carroll et al., 2017). Unfortunately, NIHL is not only isolated to the adult population. It has also been estimated that 17% of teens present with evidence of NIHL (Henderson, Testa, & Hartnick, 2011). Hearing loss of this scale carries a cost of billions in health-care dollars for coverage of diagnostic services and therapeutic management (Daniell, Fulton-Kehoe, Smith-Weller, & Franklin, 1998). In an effort to mitigate the damaging effects of excessive occupational noise exposure, OSHA instituted workplace restrictions and regulations of noise exposure in the Hearing Conservation Amendment of 1983. However, a generation of workers who were employed prior to this amendment is at significant risk for NIHL. In addition, recreational noise exposure (music concerts and portable music players) has placed young adults at significant risk for NIHL. In fact, in a study by Mostafapour, Lahargoue, and Gates (1998) some degree of high-frequency hearing loss was found in nearly one-third of a cohort of college students.
There are a number of well-established risk factors related to NIHL. These factors include smoking (Palmer, Griffin, Syddall, & Coggon, 2004), male gender, diabetes mellitus, cardiovascular disease (Daniel, 2007), exposure to toxins such as carbon monoxide or hydrogen cyanide (Fechter, 2004), as well as various medications that are considered to be ototoxic (Li & Steyger, 2009). Although these risk factors are not the direct cause of NIHL, they can increase an individual’s susceptibility to the damaging effects of noise and also increase the degree of hearing loss resulting from noise exposure. In addition, there is evidence to suggest that genetics plays a role in increasing an individual’s susceptibility to NIHL. The gene Cdh23ahl has been studied extensively and found to promote noise injury. In addition, MNSOD, KCNQ4, KCNE1, PCDH15, MYH14, and HSP70 have been identified as NIHL-susceptibility genes (Wang & Puel, 2018).
The mechanisms by which acoustic energy damages the cochlea is via excessive stimulation of inner ear tissues, which leads to the generation of toxic metabolic by-products known as reactive oxygen species and free radicals (Prasher, 1998). These free radicals can overwhelm the inner ear defenses, damaging hair cells and cochlear nerve fibers (Huang et al., 2000; Kopke et al., 1999; Kopke, Coleman, Liu, Campbell, & Riffenburgh, 2002). The mechanism whereby cells are destroyed by reactive oxygen species involves damage to the mitochondria of the cell and the initiation of a “programmed cell death pathway,” referred to as apoptosis. The damage caused by exposure to high-noise levels is dependent on a number of factors including the intensity of the noise, the frequency of the noise (higher frequencies result in more damage), the period of time the individual is exposed to noise, the type and amount of hearing protection worn by the individual, the time spent away from noise, and genetic factors. It has been postulated that when exposed to high-intensity noise, capillaries in the inner ear constrict and a reduced blood supply results (Thorne & Nuttall, 1987). This leads to metabolic exhaustion, which is related to the free radical action mentioned earlier (Møller, 2000). Bohne and Harding (2000) also report that high-intensity sound can damage the reticular lamina, which results in the intermingling of cochlear fluids, creating metabolic dysfunction and hearing loss. The effects of repeated noise exposure are additive. This is likely a result of the fact that stereocilia can either collapse or become fused over time, resulting in nonoperational tip-links (Harrison, 2001). If this occurs, the pores cannot open and the neural firing of the hair cell is not triggered due to the lack of the necessary ionic exchange, which in turn results in hearing loss. Additionally, significant damage can arise from compromise of the supporting cells (Bohne & Harding, 2000), which also leads to hearing deficits over time.
The primary cochlear structure affected by noise exposure is the organ of Corti. Specifically, the outer hair cells are damaged by oxidative stress from free radical formation, as well as from the direct shearing trauma caused by the movement of the high-intensity fluid waves through the cochlea. As the intensity of noise exposure increases, inner hair cells also become involved, and at high intensities, there may be excessive neurotransmitter release that could damage nerve cells on the receiving end of this action (Bohne & Harding, 2000). Sound transmission into the inner ear creates a cochlear fluid wave, which causes a shearing force on the stereocilia of the hair cells. Interestingly, for high-intensity sound, there is a half-octave shift of the main damage site on the basilar membrane in reference to the specific frequency of the stimulus (Møller, 2000). This shift is a result of the maximum point of displacement of the traveling wave on the cochlea, moving in a more basal direction than for lower intensity stimuli. As Møller (2000) summarized in his review, in most cases of NIHL, the greatest loss of hearing sensitivity occurs at approximately 4000 Hz. This commonly observed finding usually results from exposure to broad spectrum and/or impulse noise stimuli. This finding is likely related to the fact that the resonant frequency of the ear canal, which typically occurs around 3000 Hz in the adult, augments the intensity of the stimulus at this frequency. If one considers this frequency and adds the half-octave shift, the resultant frequency of peak damage is typically at or around 4000 Hz, where the greatest amount of hearing loss occurs.
There are other extraneous factors that have been found to contribute to and/or compound hearing loss in cases of noise exposure. For example, exposure to ototoxic substances may increase the injury caused by noise (Morata, Dunn, Kretschmer, Lemasters, & Keith, 1993). It should also be noted that there is significant evidence that insult at the level of the cochlea will result in transsynaptic retrocochlear degeneration, resulting in CANS involvement (Fabiani, Mattioni, Saponara, & Cordier, 1998). This should be kept in mind when evaluating these patients.
Noise-induced hearing loss is becoming an ever-increasing and widespread hearing health problem. Noise exposure has traditionally been linked to occupational exposure to high-noise levels, but an increasing number of patients are being seen today for NIHL related to recreational activities. In particular, the use of personal listening devices has become even more problematic today than it ever has in the past. A recent systematic review of the literature revealed that 58.2% of adolescents and young adults exceed safety standards (100% of daily noise dose) with respect to acceptable levels of noise exposure (Jiang, Zhao, Guderley, & Manchaiah, 2016). As a result, patient populations once believed not to be at risk for noise exposure have become target populations. Therefore, prior to any audiologic evaluation, a carefully elicited patient history becomes critical in determining if the patient may be at risk for NIHL.
Audiologic evaluation of patients with noise exposure is essential for the identification and monitoring of NIHL. It is important to be sure the patient has recovered completely from any noise exposure that may have resulted in TTS before testing for permanent hearing loss. Usually, there is recovery from TTS within approximately 16 hr after last exposure to noise (i.e., assuming the TTS was not more than 40 dB) (Gelfand, 2001). The traditional evaluation should include—but not be limited to—otoscopy, comprehensive audiometry, and immittance testing. However, it is also recommended that the use of ultra-high-frequency audiometry be considered as part of the battery (Korres, Balasouras, Tzagaroulakis, Kandiloros, & Ferekidis, 2008) as it has been demonstrated that these patients will present with greater degrees of involvement in the high-frequency range when compared to patients who are not exposed to significant noise levels. In addition, it also has been recommended that patients with histories of noise exposure undergo otoacoustic emissions testing as this measure often demonstrates increased sensitivity to NIHL over that provided by the pure-tone audiogram (Helleman, Jansen, & Dreschler, 2010). Thus, the administration of otoacoustic emission testing may allow earlier detection of ears at risk for NIHL; however, it should be noted that the improved sensitivity of otoacoustic emissions over the pure-tone audiogram has not been convincingly demonstrated and further research is needed in this area.
The typical configuration of hearing loss associated with noise exposure is high frequency in nature with a “noise notch” observed in most cases. This notch typically occurs at or around 4000 Hz and the width of the notch (indicating involvement of more frequencies) will spread as the degree of hearing loss increases. The notch in the audiogram is believed to occur because the maximum damage occurs at approximately 5 to 15 mm from the oval window, which corresponds to this particular frequency range (Jerger & Jerger, 1981). Noise-induced hearing loss also can affect neighboring frequencies; therefore, the interoctaves frequencies of 3000 and 6000 Hz should be tested as the maximum hearing loss may occur at one of these frequencies and not only at 4000 Hz. For example, if the stimulation frequency is relatively narrow in spectrum and centered in the midfrequencies, the maximum loss will likely not be at 4000 Hz, but at a lower frequency (recall the half-octave shift) (Lim, Dunn, Ferraro, & Lempert, 1982). The hearing loss in patients with NIHL is typically symmetric unless there is a history of unilateral exposure through such activities as shooting, in which unpredictable patterns may occur (Sataloff, Hawkshaw, & Sataloff, 2010). At times, individuals with long-standing conductive loss actually can preserve their hearing as the conductive loss attenuates the intensity of noise exposure. This sometimes happens in only one ear, resulting in asymmetric sensory levels.
Another factor to consider (as has been alluded to earlier) in the evaluation of those with a history of noise exposure is the longer the exposure, the greater the degree of hearing loss and the greater the number of frequencies affected (see Gelfand, 2001). However, it is important to recognize that there is considerable variability in humans in regard to the degree of hearing loss related to noise exposure (Gelfand, 2001). Susceptibility to NIHL may be genetically determined, at least in part, in that some auditory systems are simply more or less resistant to the damaging effects of noise. Factors related to this could be how well the acoustic reflex and efferent auditory systems function, the noise exposure history (type, intensity, frequency, duration, etc.), the presence or absence of any comorbid toxic exposures, and the patient’s age and general well-being.
Any patient with hearing loss should undergo a thorough head and neck history and physical examination. The clinician must carefully document the type and duration of occupational and recreational noise that the patient is (and/or has been) exposed to, along with any protective measures the patient has utilized. Inspection of the external ear and tympanic membrane is typically unremarkable; however, acoustic trauma can cause spontaneous tympanic membrane perforation. Tuning fork evaluation should be performed. If an asymmetry in puretone audiometry, tuning fork evaluation, or word recognition testing is identified, then further testing such as ABR or magnetic resonance imaging (MRI) should be performed to rule out retrocochlear pathology.
The management of patients with noise exposure typically involves both counseling and amplification (depending on the severity of the hearing loss). Knowledge of hearing loss prevention and conservation practices is critical for any audiologist who sees patients at risk for noise exposure (occupational and/or recreational). Prevention and conservation of hearing using appropriate tools such as ear plugs, muffs, or a combination of these devices is always recommended for patients with significant histories of noise exposure. Devices such as custom musicians’ ear plugs may also be implemented depending on the specific needs of the patient.
In counseling and testing, it is important to consider the concept of damage risk criteria and the recommendations offered by OSHA (1983) and the National Institute of Occupational Safety and Health (NIOSH, 1998) for noise exposure criteria. Although a comprehensive discussion of damage risk criteria is beyond the scope of this section, a few comments will be made regarding the NIOSH (1998) and OSHA (1983) recommendations. NIOSH has a 3 dB exchange rate for its maximum noise exposure recommendation of 85 dBA for 8 hr. This exchange rate means that for every increase of 3 dB in the noise level, the time of permissible exposure is halved. For example, using this exchange rate, the maximum exposure would be 4 hr at 88 dBA, 2 hr at 91 dBA, and only 28 sec at 115 dBA. The OSHA regulations have a 5 dB exchange rate starting at 90 dBA for 8 hr. Applying the exchange rate specified in the OSHA regulations, the maximum exposure would be 4 hr at 95 dBA, 2 hr at 100 dBA, and 15 min at 115 dBA (see Gelfand, 2001). All employees are required to wear ear protection supplied by the employer when steady-state noise levels exceed these permissible exposure levels. However, for some employees (e.g., those who demonstrate significant changes in their hearing thresholds during their annual audiometric testing—see discussion in the following text), the threshold for action is 85 dBA for 8 hr of exposure (or its equivalent time-weighted average for higher intensities). The same 5 dB exchange rate begins at 85 dBA exposure for 8 hr, so that the permissible noise exposure at 90 dBA would be 4 hr and so forth (OSHA, 1983).
Criteria are also suggested for the degree of permanent threshold shift. There have been several approaches over the years. These approaches account for aging and permanent threshold shift. In evaluating individuals who have had noise exposure, NIOSH (1998) defined a significant threshold shift as equal to or greater than a 15 dB shift at 500, 1000, 2000, 3000, 4000, or 6000 Hz in either ear. This criterion is not employed as much as the OSHA criteria for pure-tone average (PTA), which is an average threshold shift of 10 dB or more at 2000, 3000, and 4000 Hz in either ear. Counseling should include information on hearing protection, which should be recommended for individuals working in 85 dBA or greater of noise. Protection commonly consists of ear plugs or muffs or a combination of the two. Ear muffs (especially those that are fluid-filled) generally provide a better noise-reduction rating than ear plugs when worn on the job, but this is not necessarily the case in laboratory studies. It is important to know that noise attenuation measured for both plugs and muffs are greater in the lab than in the field. For example, E-A-R foam plugs have been reported to provide a noise reduction rating of 14 dB in the field and 30 dB in the lab (Berger, 1993). This obviously reflects (among other things) the difference between what might be considered an ideal fitting based on the research lab findings and a less-than-ideal fitting in the workplace.
For patients who demonstrate hearing loss severe enough to warrant intervention beyond the fitting/provision of personal hearing protection, traditional amplification is typically appropriate. However, in extreme cases of hearing loss where hearing loss is too severe for traditional amplification, cochlear implantation may be considered.
At the current time, there is no known treatment for NIHL other than better hearing protection, reduction in exposure, and better attention to good aural health. Because the injury caused by noise is oxidative in nature, some researchers have suggested the use of antioxidants, such as N-acetylcysteine, magnesium, salicylate, and vitamins B and E (Kopke et al., 2007; Kramer et al., 2006; Le Prell, Yamashita, Minami, Yamasoba, & Miller, 2007; Lynch & Kil, 2005; Sendowski, 2006; Suckfuell, Canis, Strieth, Scherer, & Haisch, 2007). A variety of otoprotectants have been shown to reduce cell death in laboratory trials and are being investigated in preclinical trials (Le Prell, 2019). These preclinical trials are focused on the use of medications prior to or immediately following noise exposure to prevent NIHL and the results are encouraging, but they currently do not seem to have an effect on reversing long-standing NIHL. Clearly, this work holds promise and, in the not-too-distant future, it is possible that medications may be available to prevent NIHL.
Case 5–2: Noise-Induced Hearing Loss
History
A 46-year-old male was seen for evaluation of work-related hearing loss. He reported a 28-year history of occupational noise exposure from working as a heavy equipment operator in underground mines and noted a gradual decrease in hearing sensitivity with particular difficulty hearing in the presence of background noise. He denied the routine use of hearing protection as well as any history of recreational noise exposure, family history of hearing loss, or ototoxic medicine exposure, but reported constant tinnitus bilaterally with no other significant history.
Audiology
A comprehensive audiologic evaluation indicated a mild sloping to severe sensorineural hearing loss bilaterally (Figure 5–2). The configuration of the hearing loss was consistent with noise exposure with a “noise notch” observed at 4000 Hz for both ears. Speech recognition thresholds were in good agreement with pure-tone averages bilaterally and word recognition was excellent for the right ear and good for the left ear. Tympanometry indicated normal pressure, volume, and compliance bilaterally, suggesting normal middle ear status (see Figure 5–2). Acoustic reflex thresholds were noted at reduced sensation levels at 500, 1000, and 2000 Hz in the right ear and at 500 and 1000 Hz in the left ear (a reflex was not obtained at the limits of the tympanometer at 2000 Hz in this ear). These results were consistent with a cochlear site of involvement for both ears.
Medical Examination
The patient presented with an essentially normal otolaryngologic examination.
Impression
Bilateral noise-induced hearing loss.
Audiologic/Medical Recommendations and Management
It was recommended that the patient obtain binaural amplification to assist with hearing loss and difficulties. In addition, the patient was counseled regarding the importance of using hearing protection both occupationally and recreationally.
Case 5–3: Noise-Induced Hearing Loss
History
An 11-year-old female was seen following a recent failure on a school hearing screening test. The child’s mother provided background information and reported significant concerns regarding her daughter’s hearing health, indicating that her daughter had failed the school hearing screening for 2 consecutive years. However, the mother’s most significant concern was the level at which her daughter listened to her MP3 player, stating that both she and her husband could hear it easily from across the room. No other significant audiologic or otologic history was reported.
Figure 5–2. Pure-tone thresholds, speech audiometry, and tympanometry results for a 46-year-old male who had sustained a work-related noise-induced hearing loss (Case 5–2).
Audiology
An otoscopic check was unremarkable bilaterally. Tympanograms indicated normal pressure, volume, and compliance, suggesting normal middle ear function for both ears (Figure 5–3A). Results from the audiologic examination revealed essentially normal peripheral hearing sensitivity in the low to mid frequencies with a characteristic “noise notch” in the higher frequencies with a maximum hearing loss of 30 dB HL observed for 4000 Hz in both ears. Word recognition scores were excellent bilaterally and speech recognition thresholds were in good agreement with the pure-tone averages. Distortion product otoacoustic emissions were performed and demonstrated present responses through 2000 Hz with absent responses for the remaining frequencies bilaterally (Figure 5–3B).
Medical Examination
The patient presented with a normal otolaryngologic examination.
Impression
Bilateral noise-induced hearing loss.
Audiologic/Medical Recommendations and Management
The patient and her parents were counseled regarding the importance of protecting her hearing by listening to her music at reduced levels. It also was recommended that the patient undergo routine monitoring of her hearing sensitivity given the pure-tone findings of a mild hearing loss at 4000 Hz in both ears coupled with the fact that changes in cochlear sensitivity were seen on the otoacoustic emissions test at frequencies not reflected on the audiogram. The otoacoustic emissions test results implicated a decrease in cochlear function that eventually may appear on the audiogram; therefore, these findings were given serious consideration when determining the management and follow-up recommendations for this particular patient.
Ototoxicity has been defined as the effects of certain therapeutic agents and other chemical substances on inner ear structures, which result in cellular degeneration of the tissues of the inner ear, with a particular propensity for damage to the end-organs of the inner ear and the neurons of the cochlear and vestibular branches of the eighth cranial nerve (Hawkins, 1976). The ototoxic side effects of medications have been known for more than a century and were first noticed with the use of the antimalarial medication, quinine (Roosa, 1875). Since then, many medicines have been implicated as being ototoxic; however, the majority of the ototoxic medications fall into five categories and include antibiotics, analgesics, antimalarials, antineoplastics, and diuretics. This segment of this chapter is limited to an overview of toxicity caused by drugs in these categories; however, a more exhaustive background on medications/drugs can be found elsewhere (Campbell, 2007; Offerdahl & Mishra, 2019). The term ototoxicity refers to a general toxic effect on the inner ear, but certain medications can be specifically cochleotoxic or vestibulotoxic. In addition, the effects of ototoxic agents may either be temporarily ototoxic (i.e., the damage is reversible) or largely irreversible, depending on the specific agent or medication. Some prescribed medications represent the standard of care for treating life-threatening conditions (i.e., serious infections or cancer); thus, the benefit of using these medications outweighs the potential toxicity. Other commonly used medications may also carry the risk of ototoxicity, but that risk is lowered when careful prescription and monitoring practices are observed. Although the majority of ototoxic medications are administered orally or intravenously, some are used in ototopic preparations and the potential exists for ototoxicity to develop if the drugs should enter the middle ear through a perforation of the tympanic membrane and be absorbed through the round window into the inner ear.
Figure 5–3. Pure-tone thresholds, speech audiometry, and tympanometry results (A) and distortion product otoacoustic emissions (DPOAEs) (B) for an 11-year-old female with noise-induced hearing loss (Case 5–3).
Note: The heavier (upper) solid line on the DPgram indicates the cutoff criteria for normal DPOAE values and the thinner (lower) solid line represents the average noise floors (see text for further explanation). Key: X = left ear response, O = right ear response, triangle = recorded noise floor response.
The primary antibiotic class implicated in ototoxicity are the aminoglycosides, which include gentamicin (Moffat & Ramsden, 1977), tobramycin (Walker, Fazekas-May, & Bowen, 1990), amikacin (Beaubien et al., 1990), neomycin (Murphy, 1970), kanamycin (Edson & Terrell, 1987), and streptomycin (Hinshaw, Feldman, & Pfueteze, 1946). These medications have been used systemically in the treatment of bacterial sepsis and resistant infections. Topical preparations have been utilized in otologic and ophthalmologic infections as well. Some aminoglycosides, such as gentamicin and streptomycin, have a predilection to selectively ablate the vestibular system, which can be used therapeutically in cases of vestibulopathy. Other ototoxic antibiotics include erythromycin and vancomycin.
The primary ototoxic analgesics are salicylates, which include such agents as aspirin (Schwabach, 1884). The hearing loss associated with high doses of aspirin is often reversible. Quinine is an ototoxic, naturally occurring compound derived from the bark of the cinchona tree that has been used for centuries as an analgesic, but it also has been utilized to treat and prevent malaria.
Antineoplastic drugs typically have an unfavorable side effect profile and can be toxic to multiple systems. Cisplatinum is a chemotherapeutic agent used to treat a variety of solid tumors, but it carries significant ototoxicity. Other ototoxic antineoplastics include 5-fluorouracil, bleomycin, and vincristine (Walker et al., 1990). Loop diuretics, such as furosemide and ethacyrinic acid, produce diuresis of excess body fluid to treat a variety of conditions ranging from pulmonary edema to hypertension; however, they also carry significant ototoxicity, particularly when given in a high dose via an intravenous route (Kumra & Bargman, 2014). One important consideration clinicians should keep in mind regarding otoxicity is that while most drugs result in irreversible cochlear toxicity, some drugs such as loop diuretics are in fact reversible (Lanvers-Kaminsky, Zehnhoff-Dinnesen, Parfitt, & Ciarimboli, 2017).
The clinical presentation of ototoxicity generally involves sensorineural hearing loss and tinnitus and may also include vestibular dysfunction. The hearing loss is typically bilateral, involving primarily the high frequencies in the early stages of the ototoxic damage, with potential involvement of the mid and low frequencies if the ototoxic medication is continued. The tinnitus also tends to be high-pitched and is typically constant in nature. Patients may present with vertigo and nystagmus or may have global disequilibrium and poor balance when the vestibular system is affected. Salicylates and quinine present with reversible tinnitus and hearing loss that is dose dependent and abates when the medication is discontinued. Cochlear toxicity of aminoglycosides is more common with kanamycin, tobramycin, and neomycin, whereas vestibulotoxicity is more common with streptomycin and gentamicin; however, all of these drugs will have some toxic effects on both the cochlear and vestibular function. Antineoplastics, such as cisplatinum, initially result in a mild, reversible high-frequency hearing loss, but as the total drug dose increases, the loss may progress to involve all frequencies and become permanent. Hearing loss due to loop diuretic use typically is in the middle frequencies and it tends to be accompanied by tinnitus, with both of these symptoms being reversible with discontinued use of the medication (Rybak, 1993).
The incidence of ototoxicity due to aminoglycosides is reported to range from 2% to 15% (Kahlmeter & Dahlager, 1984) and may be related to concomitant use of other ototoxic drugs, the type of drug(s) used, the total dose and duration of the pharmacologic treatment, and the patient’s liver and renal function and age. Loop diuretics have an ototoxicity incidence of 1% to 6% (Tuzel, 1981), with incidence and the extent of the hearing loss typically related to the medication dosage, and in the case of intravenous administration, to the rate of infusion. Cisplatinum causes ototoxicity in 55% to 100% of patients receiving the drug (Paken, Govender, Pillay, & Sewram, 2016). The incidence of ototoxicity of salicylates and antimalarials is likely very low; however, considering the widespread worldwide use of these medications, inconsistent reporting leads to difficulty in prediction of toxicity. The effects of these medications does seem to be dose dependent.
A variety of mechanisms are involved in ototoxicity as these drugs affect a variety of inner ear structures. Aminoglycosides cause a disruption of neural function by causing degeneration of cochlear hair cells (Huizing & de Groot, 1987), endolymph electrolyte alteration (Mendelsohn & Katzenberg, 1972), and free radical formation (Forge & Schacht, 2000). As described previously, free radical formation can lead to cell death. Loop diuretics alter electrolyte composition in the inner ear fluids leading to sensory dysfunction of the organ of Corti (Arnold, Nadol, & Weidauer, 1981), and medications in the antineoplastic, analgesic, and antimalarial categories affect multiple cochlear structures. In recent years, advances have been made in further elucidating the mechanisms by which hearing loss is caused by ototoxic medications. For example, loop diuretics have been found to form edematous spaces in the stria vascularis and may induce vasoconstriction within the cochlea (Ding et al., 2016). It is beyond the scope of this chapter to discuss the mechanisms of drug-induced toxicity for all drug categories; therefore, the reader is referred to Lanvers-Kaminsky et al. (2017) for a thorough review of this topic.
There is significant overlap in the site of toxicity for many of these medications; therefore, they may act synergistically to damage the cochlea. Aminoglycosides have been shown to damage the inner hair cells, the stria vascularis, the marginal cells, and the spiral ganglion (Lanvers-Kaminsky et al., 2017). Initially, the cochlear hair cells are lost at the basal cochlea, with subsequent involvement of the hair cells toward the apex with continued use of the agent/medication. Platinum-containing chemotherapeutics cause degeneration of the outer hair cells of the cochlear basal turn (Strauss et al., 1983). Loop diuretics cause injury to the stria vascularis, which regulates electrolyte balance of the fluids within the cochlear duct (Quick & Duvall, 1970). Outer hair cell function is likely affected by antimalarial drugs such as quinine and macrolides. In addition, drugs such as erythromycin and azithromycin that may be used to treat bacterial infections can demonstrate ototoxic effects; however, the exact location of injury has yet to be elucidated (Lanvers-Kaminsky et al., 2017).
Ototoxicity typically yields an audiometric configuration of high-frequency hearing loss that affects both ears. In advanced cases, the mid to low frequencies can become progressively involved. As with NIHL, otoacoustic emissions may be useful in heralding hearing loss before audiometric evidence of the hearing loss can be documented.
The evaluation of these patients is entirely dependent on a number of factors, which include the age of the patient, his or her ability to respond to testing, and the medication itself. The authors suggest using the model recommended by Jacob, Aquiar, Tomiasi, Tschoeke, and Bitencourt (2006) for initial evaluation, as described in the following outline. The subsequent schedule for audiologic monitoring typically is decided by the team of individuals managing the patient and is based on a consideration of the variables mentioned previously (see also Campbell & Le Prell, 2018).
I. For patients who cannot respond behaviorally
a. OAEs (distortion product or transient stimulus)
b. Immittance audiometry
c. ABR
II. For patients who can respond behaviorally
a. Threshold audiometry (including ultra-high-frequency testing)
b. Immittance audiometry
c. OAEs (distortion product or transient stimulus)
d. ABR (when indicated)
Patients who are taking medications that are known to be ototoxic may be doing so to treat a serious condition for which the medication is necessary. Tuning fork evaluation should be performed. If the vestibule has been compromised, spontaneous or gaze-evoked nystagmus may be present. Tests of central balance function such as the head-shake maneuver or the Romberg test may indicate global balance dysfunction. The head-shake maneuver is performed by having the patient vigorously shake his or her head back and forth for approximately 20 to 30 sec. The patient then abruptly stops shaking his or her head. A positive head-shake result occurs when a nystagmic response is observed even after the head-shake has stopped, indicating an uncompensated imbalance of the vestibulo-ocular system. Videonystagmography may further be used to confirm clinical findings of vestibulopathy. If asymmetry in pure-tone audiometry, tuning fork evaluation, or word recognition testing is identified, then further testing such as imaging (MRI) and/or ABR should be performed to rule out retrocochlear pathology.
The clinical course for the progression of hearing loss varies significantly among patients with ototoxic exposure. Certainly, periodic testing when patients are receiving ototoxic agents for either treatment or ablation is necessary. The interval at which this occurs varies from individual to individual and is often dependent upon the patient’s treatment protocol. As discussed earlier, the hearing loss in patients with ototoxic exposure typically begins as a high-frequency sensorineural hearing loss that progresses to varying degrees, often spreading to involve the mid to low audiometric frequency range. Tympanometry is typically normal unless comorbid otitis media or some other middle ear condition is present. Patients undergoing radiation to the head and neck may often demonstrate abnormal tympanometry secondary to the effect of radiation on the Eustachian tube. In these cases, if a hearing loss is present, there is often a conductive component to the hearing loss noted (Akazawa et al., 2018). The primary treatment for patients with sensorineural hearing loss following treatment involving ototoxic agents is amplification. However, in extreme cases of hearing loss where the hearing loss is too severe for traditional amplification, cochlear implantation may be considered.
The primary treatment of ototoxicity is discontinued use of the offending agent. As stated previously, this may not be possible if the medication is the primary treatment for serious disease. However, prevention of ototoxicity for many patients without life-threatening disease is a realistic goal for clinicians; therefore, health-care providers in all disciplines should be aware of steps that can be taken to minimize inner ear injury. Aminoglycoside blood serum levels have been used to measure peaks and troughs in the amount of medication present in the patient’s blood; however, ototoxicity can be most accurately predicted by the total dose of drug given over the course of treatment (Beaubien et al., 1991). Generally, loop diuretics and antineoplastics should be administered slowly and rapid intravenous push administration should be avoided. Often, salicylate and quinine toxicity can be reversed by stopping usage of the drug(s). Patients who are able to be tested audiologically and for whom a prolonged course of treatment is anticipated are often evaluated with a baseline audiogram and pretreatment renal function tests, and then these tests are repeated during therapy to identify toxicity at its early stages. This pretreatment assessment and then monitoring with regular audiograms during therapy is commonly done for patients receiving certain chemotherapeutic agents, such as cisplatinum. The administration of fosfomycin, an antibiotic, has been shown to protect the cochlea during cisplatinum administration (Schweitzer, Dolan, Abrams, Davidson, & Synder, 1986). The use of antioxidants may also have a role in preventing hearing loss in some patients receiving ototoxic medications, but application of antioxidants as a preventive intervention for ototoxic hearing loss has not yet reached clinical practice. In addition to the monitoring schedule, it is important to counsel patients to notify their medical team should they notice any changes in hearing or should they develop symptoms such as tinnitus and/or imbalance (see Campbell & Le Prell, 2018, for review).
History
A 36-year-old female was seen for an audiologic evaluation prior to beginning chemotherapy for tonsillar cancer. The patient presented with no reported history of audiologic or otologic involvement.
Audiology
An otoscopic check was unremarkable bilaterally. Prechemotherapy responses to pure-tone audiometry indicated normal peripheral hearing sensitivity bilaterally and excellent word recognition scores for both ears (Figure 5–4A). Test reliability was considered to be good. Postchemotherapy results demonstrated normal peripheral hearing sensitivity sloping to a mild sensorineural hearing loss for the high frequencies in both ears (Figure 5–4B).
Medical Examination
The patient presented with an essentially normal otologic examination.
Impression
Bilateral sensorineural hearing loss secondary to ototoxicity.
Audiologic Recommendations and Management
Results of the audiologic evaluation were reported to the oncologist. The chemotherapy regimen was adjusted given the ototoxic effects of the chemotherapy. The patient’s hearing stabilized following the adjustment to her medication and she continues to be monitored throughout her treatment.
Medical Recommendations and Management
Continue to monitor hearing.
MÉNIÈRE’S DISEASE (ENDOLYMPHATIC HYDROPS)
The clinical constellation of episodic vertigo with unilateral hearing loss, tinnitus (often described as roaring), and aural fullness/pressure is referred to as Ménière’s syndrome (also referred to as idiopathic endolymphatic hydrops). This condition may be caused by infectious, immunologic, or traumatic insults to the inner ear, which result in increased endolymphatic fluid pressure. The idiopathic form of this syndrome is referred to as Ménière’s disease. The French otologist Prosper Ménière first described this disorder in 1861 (Ménière, 1861a, 1861b) and this disease bears his name. Although the clinical presentation of this disease has been described, the etiology is poorly understood and this disease continues to be a clinical challenge for physicians, audiologists, and patients.
Figure 5–4. Pure-tone thresholds and speech audiometry results prior to (A) and after chemotherapy (B) for a 36-year-old female with tonsillar cancer (Case 5–4). continues
Four classic symptoms are seen as a cluster in patients with Ménière’s disease (Clemmens & Ruckenstein, 2012). These include intermittent attacks of vertigo lasting 20 min to 2 hr in duration along with the sensation of aural fullness or pressure, the experience of tinnitus, and sensorineural hearing loss in the involved ear (Gates, 2006). Initially, the hearing loss presents as a low-frequency sensorineural hearing loss that may fluctuate over an unpredictable amount of time. However, over time, the hearing loss gradually beings to involve the middle and higher frequencies, often leaving the patient with a permanent moderately-severe to severe sensorineural hearing loss in the affected ear. Severe, even debilitating, episodic vertigo is the primary symptom that leads to medical evaluation. This spinning sensation is accompanied by nausea and vomiting, and following an attack, patients typically experience an overwhelming sense of fatigue and exhaustion. The interval between episodes is quite variable and may change during the course of the disease. Around the time of each attack, a sensation of aural fullness is often experienced, and the severity of the tinnitus is typically amplified. These episodes may vary from as frequently as several times a week to as rarely as once or twice in a lifetime. A significant number of patients with Ménière’s disease will experience a phenomenon known as “burnout” in which they no longer experience attacks of vertigo but are left with a stable hearing loss, tinnitus, and varying degrees of aural fullness. Patients also may experience a sudden drop attack, also referred to as a crisis of Tumarkin, in which they fall suddenly without provocation or warning but maintain consciousness. These drop attacks may be related to otolithic stimulation by the increased inner ear fluid pressure (Baloh, Jacobson, & Winder, 1990). Lermoyez variant of Ménière’s disease is characterized by a hearing loss that briefly improves around the time of a vertigo attack (Xenellis, Linthicum, & Galey, 1990). Ménière’s disease initially affects one ear, but may involve both ears in 17% to 50% of patients (Silverstein & Rosenberg, 1992; Stahle, Friberg, & Svedberg, 1991).
As diagnosis of Ménière’s disease is made based primarily on reported symptoms, it is important for the clinician to understand the diagnostic criteria set forth by the American Academy of Otolaryngology-Head and Neck Surgery’s Committee of Hearing and Equilibrium (1995), which were designed to aid in the diagnosis of this particular inner ear disorder. The criteria are based on the following major symptoms: (1) vertigo, (2) deafness [hearing loss], and (3) tinnitus or aural fullness. The vertigo must include recurrent, well-defined episodes of spinning lasting from 20 min to 24 hr. In addition, nystagmus associated with the vertiginous attacks must be observed, resulting in possible nausea and vomiting during the spells when the neurologic symptoms are experienced. The deafness [hearing loss] typically reveals a unilateral fluctuating sensorineural hearing loss. And finally, tinnitus as a symptom is often unilateral, subjective, variable, and low-pitched in nature. There are four categorizations of Ménière’s disease and patients are categorized based on their reported symptoms and test findings as detailed in Table 5–1. However, a certain diagnosis of Ménière’s disease can only be made postmortem through histology. Of note is that the AAO-HNS is currently in the process of developing new clinical practice guidelines for Ménière’s disease, which will be available on their website (http://www.entnet.org).
Table 5–1. Categorization of Ménière’s Disease Based on Reported Symptoms
Source: Based on “Committee on Hearing and Equilibrium Guidelines for the Diagnosis and Evaluation of Therapy in Meniere’s Disease,” by American Academy of Otolaryngology, 1995, Otolaryngology–Head and Neck Surgery, 113(3), 181–185.
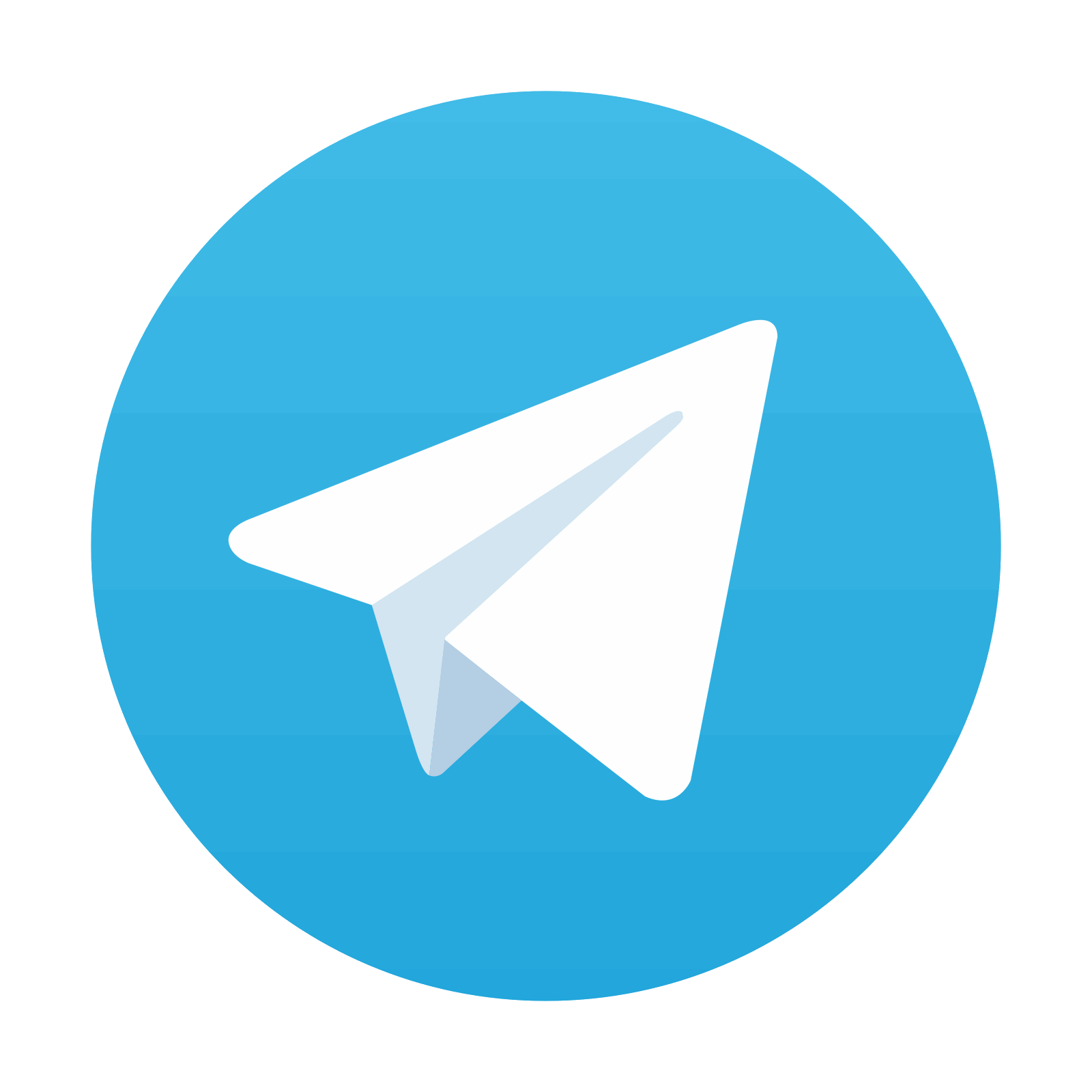
Stay updated, free articles. Join our Telegram channel

Full access? Get Clinical Tree
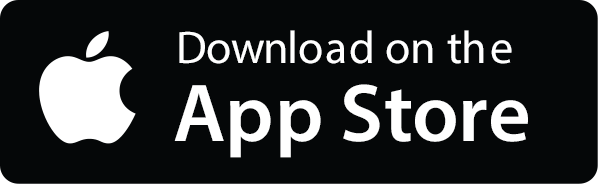
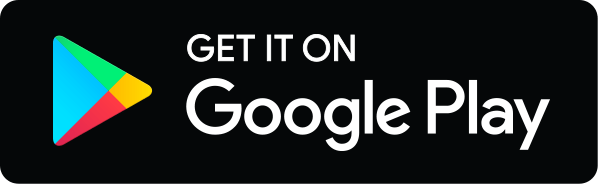