Disorders of the Central Auditory Nervous System
When considering disorders of the central auditory nervous system (CANS), it must be understood that it is not necessarily the type of disorder but rather the location of the lesion and the specific effects of the disorder on the CANS (i.e., the disease mechanisms) that are the crucial issues. Anatomically, the CANS begins at the level of caudal pons, specifically at the cochlear nucleus. At a similar level, but located deep in the pons, is the next major group of nuclei: the superior olivary complex (SOC). The SOC projects fibers along the lateral lemniscus, a major brainstem pathway that also has a group of nuclei in the upper half of the pons. The next nucleus in the CANS is the inferior colliculus. This nucleus is located in the midbrain and receives input from practically all of the more caudally located auditory nuclei and projects to the underside of the thalamus to the medial geniculate body (MGB). The MGB sends fibers to the auditory cortex, specifically Heschl’s gyrus and secondary auditory areas such as the insula. The corpus callosum connects the right and left hemispheres of the brain and has a specific auditory region where impulses are exchanged between the two hemispheres.
Many disorders can result in audiologic deficits if there is insult to the auditory neural substrate within the CANS. However, the effects of CANS damage often result in audiologic findings that are not unique to a particular disorder but rather to the site of the CANS lesion. Some of the more significant disorders that can result in central auditory deficits are discussed in this chapter. Many of these disorders are often overlooked by healthcare professionals due to the fact that other comorbid conditions (e.g., paralysis, vertigo) often overshadow the auditory symptoms. In some cases, these disorders may not result in hearing deficits, but in others, they certainly can and do. When they do, it is important to determine the nature and degree of the deficit(s) so that optimal medical and/or audiologic treatment can be realized.
The audiology sections in this chapter are organized in a slightly different manner than in previous chapters. This was done for efficiency. We discuss the audiologic profiles associated with various types of CANS disorders as has been done in other chapters; however, due to the similarity of the audiologic findings in mass and vascular lesions, rather than dedicating a separate section to the audiologic findings for each of these lesion sites, the discussion is combined. Audiologic findings are provided separately for the following lesion types: degenerative disorders, neurotoxicity, traumatic brain injury, temporal lobe epilepsy, surgical compromise of the CANS, and learning difficulties.
In the assessment of various disorders of the CANS, it is useful to understand that various tests have anatomic limitations. Some central tests are efficient for the assessment of brainstem involvement, whereas others may be better suited for use with cortical or interhemispheric compromise. Also, some disorders may manifest their dysfunction primarily in one of these three anatomic regions, whereas others may affect multiple areas. This, of course, influences the types of tests selected for administration during evaluation of the patient. For example, a small tumor of the low brainstem would have a focal effect in the pons. On the other hand, heavy metal neurotoxicity could involve the entire auditory system. Therefore, the selection of the tests to be administered to a particular patient will depend on the patient’s case history and presenting symptomatology, as well as the results of any tests that are initially administered during the patient’s evaluation, as these may indicate that additional testing is needed to explore different (or potentially additional) auditory processes and/or sites of lesion.
Two main categories of central tests are discussed in this chapter. These include psychophysical (i.e., behavioral) tests and electrophysiologic tests. The psychophysical test category includes dichotic listening, temporal processing, low redundancy speech, and binaural interaction tests, whereas the electrophysiologic procedures to be discussed include the auditory brainstem response (ABR), the middle latency response (MLR), the N1 and P2 late potentials, and the P300 (also referred to as the P3 potential). On occasion, a brief discussion of other auditory evoked potentials also is included. Finally, there are some electroacoustic tests (e.g., otoacoustic emissions) that are often used to help differentiate a central site of lesion from a peripheral one. Although the otoacoustic emissions test does not assess the integrity of the CANS, it can be used to rule out a significant peripheral hearing loss.
In cases of CANS involvement, the goal of testing generally is not to make the diagnosis as in many instances, the disorder is already known and many medical procedures are better at defining the lesion than are the central auditory tests. However, there are three major goals of audiologic testing when CANS involvement is either suspected or confirmed. The first is as a screener. The audiologist may be the first professional to see a patient with a CANS disorder and therefore has the responsibility to make the appropriate referral for medical and/or psychological/psychiatric follow-up. This, of course, cannot be done unless central dysfunction is determined. Although not a common occurrence, this situation does happen and when it does, appropriate follow-up and management of the patient is essential. The second major goal in utilizing central tests in CANS disease is to determine if the central auditory system is involved. For example, a patient with a long-standing diagnosis of multiple sclerosis might seek audiologic assessment because of a new symptom of hearing difficulty. The key here is to determine if this new symptom is really auditory in nature or not, and if it is, whether it is due to peripheral or central system compromise. The third goal of central auditory assessment is to corroborate the medical and communicative symptoms and/or complaints of the patient with test measures and to determine the degree of the patient’s functional deficit(s). All three of these goals lead to another important aspect of audiology; that is, the appropriate management of the patient. Without the appropriate diagnostic information, proper management of the patient with confirmed or suspected CANS involvement is difficult.
Plasticity of the CANS is a factor in all brain lesions. Natural compensation by the brain for central dysfunction often occurs over time. Therefore, some lesion effects may not be as severe if the patient is assessed some time after the initial occurrence of the CANS disorder or insult as they would have been had the patient been assessed closer in time to the original disease process or CANS compromise. On the other hand, some CANS lesions may progress, creating greater problems over time, as potentially would be the case in progressive CANS disorders such as multiple sclerosis. Given these considerations, central auditory testing can be used not only to initially document the auditory deficits associated with CANS involvement, but also to monitor subsequent changes in the patient’s audiologic profile that may result as a function of brain plasticity, audiologic intervention, and/or disease progression.
Mass lesions are space occupying lesions located within the brain. Lesions that are located within or close to the auditory areas of the brain, of course, are at risk for influencing central auditory function. Sometimes, vascular lesions such as aneurysms and hematomas could be viewed as mass lesions in that they can be space occupying as they often are quite large. However, these types of lesions are classified as vascular disorders. In the brainstem, mass lesions are divided into intra-axial and extra-axial categories depending on whether the tumor resides primarily within (intra-axial) or outside (extra-axial) the brain tissue. The first reports on central auditory disorders by Bocca, Calearo, and Cassinari (1954) were on patients with temporal lobe tumors. In many cases, these patients reported varying auditory symptoms, depending on the characteristics of their tumors.
The symptoms related to mass lesions of the central nervous system depend on the size, location, type, and consistency of the tumor (Young, 1983). In terms of general symptoms, headaches, nausea and vomiting, seizures (usually at later stages), dizziness, and altered mentation can occur (Perkins, 2002). Tumors that specifically affect the CANS can result in the same symptoms, but they also may create subtle complaints of difficulty hearing in noise, tinnitus, auditory hallucinations, difficulty understanding speech, decreased appreciation of music, sound distortions, and so forth. In some cases, however, patients with CANS compromise may experience no auditory symptoms. The specific auditory symptoms experienced depend on such factors as the location of the lesion, its size and duration, the presence and amount of brain plasticity, and the age of the patient. Often, auditory symptoms are overshadowed by more overt symptoms, and the patient may have to be asked specifically about hearing abilities before any auditory difficulties are acknowledged (Bamiou et al., 2006; Musiek, Baran, & Pinheiro, 1994; Perkins, 2002).
The incidence of benign brain tumors is about 12.8 per 100,000 with females being slightly more commonly affected than males (i.e., 16 and 9.2 per 100,000 for females and males, respectively) (Howlader et al., 2019). The number of new cases of brain and nervous system cancers is reported to occur in approximately 6.4 per 100,000 individuals per year (National Cancer Institute, n.d.). When all tumor types are combined, there is definitely an age influence on the incidence of tumor occurrence, with greater occurrence reported for the elderly population than for children. However, certain tumor types are more prevalent in children. There is a monotonic function for age ranging from those under 20 years to those 85 years and older (Howlader et al., 2019). There also is an anatomic factor in the occurrence of cerebral tumors. Most tumors occur in the frontal lobe, followed by the temporal, parietal, and occipital lobes (Larjavaara et al., 2007).
In the brainstem, intra-axial tumors arise from within the brainstem structures themselves, often near the midline. Gliomas are the most common type of intraaxial brainstem tumor and include many subtypes such as astrocytomas, ependymomas, and medulloblastomas (McLeod & Lance, 1989). These mass lesions vary in prognosis, often are difficult to manage, and, because of their location, usually result in compromise of brainstem auditory function (Jerger & Jerger, 1981).
Extra-axial tumors often are meningiomas, astrocytomas, or schwannomas. These tumors occur outside the brainstem and encroach on the brainstem and cranial nerves. Probably the most common schwannoma that often affects auditory function is the vestibular schwannoma, which is actually a peripheral lesion, as was discussed in Chapter 6. However, a vestibular schwannoma larger than 2 cm will likely compress the brainstem and can be considered an extra-axial brainstem tumor. If this type of compression of the brainstem occurs, then central auditory effects are frequently observed.
Mass lesions and vascular disorders can take place anywhere in the CANS and can directly affect auditory function. Mass lesions also can result in indirect effects on CANS structures from compression or shifting of the brain. For example, due to its size and location, a large frontal lobe tumor could also compress the temporal and parietal lobes. Vascular lesions, such as strokes, tend to be more anatomically precise than mass lesions, but these also can have indirect influences. An example of this would be what is known as “vascular steal” effects. That is, a disorder in which one area of the brain “robs” another area of some of its blood supply. The area with reduced blood supply may be remote from the observed lesioned region. However, most of the time, mass and vascular lesions will result in the greatest amount of dysfunction in the anatomic area where the lesion is located.
Medical diagnosis is first concerned with determining whether the tumor is malignant or not. Approaches include a detailed general medical and neurologic history. This is generally followed by a neurologic exam. Radiologic investigation relies primarily on magnetic resonance imaging (MRI) with contrast enhancement, but occasionally computerized tomography (CT) is used (see Chapter 3, “Audiological, Vestibular, and Radiologic Procedures”). MRI can provide considerable information about tumor size, type, and location, which can inform the need for surgical intervention. Several tumors have such typical findings on MRI that a diagnosis can be made without a formal biopsy. This is particularly true for extraaxial tumors, such as vestibular schwannomas. However, on occasion, an intraoperative biopsy may be needed. Surgical approaches for biopsy can take a variety of forms, a discussion of which is beyond the scope of this book. However, it is important to note that surgical biopsy is often necessary for differentiating malignant from benign mass lesions.
As discussed by Perkins (2002), management of the patient with a mass lesion often depends on whether the lesion is benign or malignant. In the case of benign lesions, surgical excision is typically the most direct course of treatment. However, this approach is dependent on the age and overall health of the patient, as well as the location, type, and size of the tumor and its neurologic effects. Sometimes, careful observation is the most appropriate course of action, particularly in patients who are elderly or poor surgical candidates, as well as in cases where the tumor is slow growing and causing minimal neurologic disturbance.
For malignant tumors that are amenable to surgery, surgical resection is usually combined with radiation treatments and/or chemotherapy. Gamma knife surgery is another treatment option, which involves the targeted radiologic destruction of the tumor. Although the name would imply otherwise, this does not involve traditional surgical procedures (i.e., surgical incisions); therefore, the patient is not exposed to the side effects or risks of invasive surgery, and radiation damage to healthy tissue around the lesion rarely occurs. Gamma knife surgery can also be used to treat benign tumors as an alternative to surgery for small or medium-sized tumors if the patient prefers a nonsurgical approach. This is often the treatment of choice for vestibular schwannomas. Gamma knife surgery may also have applications for large tumors in some cases.
With the advances in radiology, diagnostic audiology is not the best approach to diagnose mass lesions. However, the audiologist may be the first person to see a patient with a mass lesion, especially if auditory symptoms (hearing loss, tinnitus, etc.) are the patient’s major complaint. If this is the case, assessment of the patient’s central auditory function is key to the diagnosis of central auditory dysfunction, and ultimately should result in an appropriate referral for medical follow-up if such a diagnosis is rendered.
A role more commonly played by the audiologist when working with patients with diagnosed brain tumors is to determine if the tumor has compromised the auditory system. If surgery is being recommended for a patient, the audiologist may also be involved in a pre- and postsurgical central auditory assessment to determine the status of the patient’s auditory function following surgery. This determination is important for the overall welfare of the patient. In addition to patients who have undergone traditional surgical procedures, those who have undergone Gamma knife surgery, chemotherapy, and/or radiologic treatments for tumors that are located in or in close proximity to the auditory areas of the brain should be evaluated for potential postsurgical compromise of central auditory function and subsequent management as appropriate.
Vascular disorders are among the most common disorders that affect the CANS. These disease processes typically result in a reduction of the blood supply to the neural substrate served by the particular vascular system affected. Because blood supply provides oxygen and glucose to the cells, removes waste matter, and is essential to overall cell metabolism, normal or unrestricted blood supply to the brain structures is critical for the normal function of these structures. Pathologic conditions of blood vessels in the brain are referred to collectively as cerebrovascular disorders.
The major types of cerebrovascular brain disease have been reviewed by Kaufman (1990) and Perkins (2002). Vascular disorders can be broadly grouped into two major categories: ischemic and hemorrhagic. Ischemic disorders occur when blood flow to an area of brain tissue is diminished. If diminished blood flow is prolonged, the condition can result in tissue infarction (referred to as an ischemic stroke). Ischemic stroke typically occurs when cerebral arteries are occluded as a result of vessel narrowing due to atherosclerosis or lodging of a blood clot in a vessel. However, compromise of arterial supply due to tumor, infection, vessel spasm, or inflammation (vasculitis) also can cause ischemic stroke. Hemorrhagic vascular lesions occur when a blood vessel ruptures and bleeds into the brain. Intracerebral hemorrhage (hemorrhagic stroke) results from damage to blood vessels, often due to high blood pressure, and results in loss of blood flow to associated brain tissue, as well as a mass effect in the brain. Hemorrhagic vascular lesions also can occur as a result of an aneurysm, which is the “ballooning” of a vessel usually secondary to weakness or damage along the vessel wall. These aneurysms can bleed, resulting in intracerebral or subarachnoid hemorrhage. All types of stroke result in the loss of blood supply to the neural substrate and can lead to either reversible or irreversible neural damage depending on their extent and duration.
Symptoms of vascular disease are dependent on the locus of the problem, the number and types of vessels involved, the degree of involvement, and patient factors such as age, general health, and activity level. One of the key symptoms of vascular disease is transient ischemic attacks (TIAs), which are caused by temporary decreases in blood supply to an area of the brain that results in temporary or transient symptoms. These TIAs can be a warning of impending stroke in 10% to 30% of the cases in which they occur (McLeod & Lance, 1989). Other symptoms related to stroke can include hemiparesis, sensory deficits, diplopia, dysarthria, ataxia, and vertigo (Tadi & Lui, 2019). Specific auditory symptoms will result when the blood supply to areas of the CANS is decreased. Symptoms that may occur when the auditory areas of the brain are compromised include tinnitus (central), hallucinations, loss of hearing sensitivity, poor hearing in noise, confusion following directions and understanding speech, localization difficulties, and changes in music appreciation. In extreme cases, total central deafness can occur when major vascular deficits affect both sides of the central auditory tracts in the brainstem or cerebral hemispheres (see Musiek et al., 1994; Musiek & Lee, 1998).
Prevalence of vascular disease varies with age, occurring in only 1 in 100,000 for those under 35 years of age and 840 in 100,000 for those over 35 years. About 80% of vascular accidents occur in individuals 65 years of age or older. Vascular problems in younger age groups are more common in men, but in older age groups, the incidence of vascular involvement is essentially equal between genders. Transient ischemic attacks are also more common in men than women (Jerger & Jerger, 1981).
Occlusive disease often manifests as atherosclerosis, which narrows or occludes the arteries. This evolves over time due to the increasing amounts of fatty materials and fibrous tissue that progressively build up within the blood system, especially at the branching points of the arteries. Atherosclerosis can damage artery walls, which triggers thrombus formation from platelets and collagen. This often can be seen in the internal carotid and vertebral basilar systems but is not as common in the middle cerebral artery (MCA). Another occlusive problem can be a cardiac embolism, a type of clot that can travel from the heart to the brain where it becomes a brain embolism that arrests the blood supply to the brain structures supplied by the particular artery affected. Vasoconstriction also can affect small vessels by reducing the lumen of the vessel and restricting blood flow through the vessel. Medications, drugs, and even natural physiologic responses such as fear can cause this action (Chaves & Jones, 2005).
When blood supply is disrupted, time becomes a key factor. In only 30 sec, ischemia develops and brain metabolism is altered. After 1 min of no blood supply, neural tissue ceases normal activity, and after 5 min of arrested circulation, neural substrate becomes anoxic leading to infarction (Toole, 1979). Smoking, poor diet, drugs, drinking, hereditary factors, and lack of exercise all can contribute to occlusive disease by accelerating atherosclerosis (Chaves & Jones, 2005).
Intracerebral hemorrhage can result in hematomas and edema, which can compress brain tissue (see Chaves & Jones, 2005; Perkins, 2002). This condition evolves quickly (usually in hours) and is related to degeneration of the blood vessel walls, a condition that can be acquired or congenital. General weakness of the vessel walls is worsened by conditions such as high blood pressure, which can further weaken the vessels. In addition, emboli fragments can cause ischemia of artery walls, which would result in a weakening of these walls. Other conditions, such as tumors and trauma, also may result in intracerebral hemorrhage. Use of anticoagulants and antiplatelet agents can worsen this condition; hence, it must be differentiated from other disorders, such as occlusive disease (for which antiplatelet therapy is usually recommended) (Perkins, 2002).
Brain aneurysms are another vascular condition that can potentially affect the blood supply to regions of the brain and have serious consequences. A brain aneurysm is an abnormal bulge or ballooning in the wall of an artery that results in a weakening of the artery wall. There are five subtypes of aneurysms: saccular (sac-shaped; most common), fusiform (spindlelike outpouching), dissecting (longitudinal splitting of the artery), traumatic (secondary to trauma), and infectious (related to infection). These types of weakened blood vessels commonly form at branching sites, are space occupying, and can result in improper blood supply to certain areas of the brain. Importantly, they also can rupture, resulting in subarachnoid bleeding, which can be fatal, and they can be a site of emboli, which can result in occlusion of various vessels (Steel, Thomas, & Strollo, 1982).
Vascular disorders that are located near auditory regions of the central nervous system can affect hearing function. Sometimes a “vascular steal” takes place, which can affect regions far from the actual site of vascular damage. The vertebrobasilar vascular system supplies the auditory brainstem pathways via branches primarily from the basilar artery. These branches are circumferential proceeding to the posterior brainstem where the auditory structures are located. Branches of the middle cerebral artery (MCA) supply the auditory cortex, and the fronto-opercular artery supplies the insula.
Chaves and Jones (2005) outline the medical management of occlusive disease. This includes a neurologically oriented history and examination, as well as a determination of the anatomic site of involvement, usually with CT and/or MRI. Commonly, cardiac function assessment and ultrasound of carotid arteries or MRI or CT angiography to identify vascular occlusion are performed. On occasion, CT perfusion studies also are indicated. Treatment can be prophylactic, primarily with antiplatelet therapy (aspirin, Plavix) and aggressive management of cholesterol and blood pressure, or surgical (carotid endarterectomy, angioplasty, or the use of stents to keep the arteries open). In patients with atrial fibrillation (which can cause clots to form in the heart that can embolize to the brain) or disorders of normal clotting function (which also may result in clot formation), anticoagulation medication often is recommended. In unusual cases, neurologists may consider cerebral vasculitis as the cause of the vascular disorder. If this condition is suspected, it is evaluated with blood tests and angiography and it can be treated with immunosuppressive drugs.
In cases of potential hemorrhage or aneurysm, history and medical examination focusing on such factors as hypertension, smoking, and alcohol use should be considered. It also is important to watch for use of anticoagulants (e.g., warfarin, heparin) as urgent reversal of anticoagulation may be required to stop bleeding. Treatment for intracerebral hemorrhage usually involves lowering blood pressure and watching for signs of increased intracranial pressure or increased hematoma size. Surgical decompression and evacuation of hematoma is sometimes required. Treatment of a ruptured aneurysm involves surgical repair of the aneurysm, followed by close clinical observation for signs of cerebral vasospasms/arterial occlusion, which can occur after many aneurysmal bleeds (Hreib & Jones, 2005; Sung, 2006).
AUDIOLOGY: MASS AND VASCULAR LESIONS
Dichotic Listening Tests
There are several commonly used tests of dichotic listening such as dichotic digits (Musiek, 1983a), the dichotic word listening test (DWLT) (Meyers, Roberts, Bayless, Volkert, & Evitts, 2002), the staggered spondaic word test (Katz, Basil, & Smith, 1963), and many others (see Baran & Musiek, 1999).
Dichotic speech tests can be sensitive to brainstem involvement but are most noted for their sensitivity in detecting hemispheric and corpus callosum (interhemispheric) dysfunction (see Baran & Musiek, 1999). Typical findings are ipsilateral or bilateral deficits for low brainstem lesions, left ear deficits for corpus callosum lesions, and contralateral ear effects for cortical lesions. However, it is interesting to note that a paradoxical left ear deficit can sometimes be noted with a hemispheric lesion. The deficit is this case is considered to be unusual (i.e., a paradox) in that the typical contralateral ear deficit is not observed, presumably due to secondary compromise of the corpus callosum or interhemispheric pathways; hence, an ipsilateral left ear dichotic deficit is noted with a left hemisphere lesion that compromises the efficient transfer of neural information across the corpus callosum (see Baran & Musiek, 1999; Musiek & Chermak, 2014; Musiek & Weihing, 2011). Although tests vary in terms of their sensitivity, dichotic listening tests as a group are considered to have moderately good diagnostic power.
A large number of studies support the value of dichotic speech tests in the evaluation of mass and vascular lesions of the cortex and subcortex. Findings demonstrate a definite contralateral deficit in adults (i.e., unless the corpus callosum is involved, where left ear deficits are commonly noted) (Cohen, Hynd, & Hugdahl, 1992; Kimura, 1961; Lynn & Gilroy, 1975; Musiek, 1983b; Musiek et al., 1994; Raeder, Helland, Hugdahl, & Wester, 2005; Speaks, Gray, & Miller, 1975; and others). Cohen et al. (1992) also showed contralateral effects from hemispheric involvement in children similar to those noted in adults. Investigations of mass and vascular lesions of the brainstem have yielded somewhat mixed results as to the type of deficits typically detected, but predominately ipsilateral and bilateral deficits have been noted on these dichotic tests (Musiek, 1983b; Musiek & Baran, 2004; Musiek & Geurkink, 1982). However, the sensitivity of dichotic listening tests to brainstem involvement is less than the sensitivity of these tests to cortical and interhemispheric dysfunction (see Baran & Musiek, 1999, and Chermak & Musiek, 2011, for reviews).
Temporal Processing Tests
Temporal processing tests include tests that assess temporal sequencing, temporal resolution, temporal integration, and/or temporal masking. This chapter focuses on examples of the first three test categories; that is, pattern perception, gap detection, and integration of tonal stimuli. Frequency and duration patterns are commonly used tests that are highly dependent on temporal sequencing as well as other functions such as auditory discrimination and linguistic labeling (see Baran & Musiek, 1999). These tests appear to require processing of the acoustic stimuli in both hemispheres if a verbal response to the test stimuli is required, even though the tests commonly are administered monaurally (in some cases they can be administered in a sound field). When a verbal response to the test stimuli is required, the right hemisphere is involved in contour recognition and sequencing of the stimuli and the left hemisphere is involved in the linguistic labeling of the processed stimuli. In order for accurate processing and a response to occur, information processed in the right hemisphere must be transferred to the left hemisphere via the corpus callosum. Related to these concepts and observations is that dysfunction or compromise in either hemisphere or the corpus callosum typically yields bilateral deficits when a verbal response is required (Musiek, Pinheiro, & Wilson, 1980). If an alternative type of response is used, such as humming the patterns, then the test procedure taps primarily right hemisphere function as there is no need to transfer the processed auditory signal across the corpus callosum to the left hemisphere for verbal labelling. If a patient is unable to accurately hum the patterns being presented, then a right hemisphere compromise would be implicated.
A number of studies have shown frequency and duration pattern perception tests to be sensitive and specific tests for mass and vascular lesions of the auditory regions of each hemisphere. These studies demonstrate primarily, but not exclusively, bilateral deficits (Baran, Bothfeldt, & Musiek, 2004; Fallis-Cunningham, Keith, & Warnick, 1998; Musiek, Baran, & Pinheiro, 1990; Musiek & Pinheiro, 1987). Poor performance on pattern perception also has been observed in patients with vascular lesions of the insula (Bamiou et al., 2006). As would be expected, patients with corpus callosum damage typically show extremely low scores for verbal report, but they can often hum the patterns without difficulty (Musiek et al., 1980). Although auditory pattern perception is affected by hemispheric and interhemispheric mass and vascular lesions, it appears that these tests are not highly sensitive to brainstem involvement (see Musiek & Chermak, 2015; Musiek & Geurkink, 1982; Musiek & Pinheiro, 1987).
The Gaps-in-Noise test (GIN) (Musiek et al., 2005) is an example of a temporal resolution test. The ability to detect extremely brief interruptions of an ongoing broadband signal (gaps) requires integrity of the CANS. It has been shown that individuals with vascular lesions of the CANS (including the insula and brainstem) require longer silent gaps than controls for accurate perception, and as a result, do not perform well on gap detection procedures (Bamiou et al., 2006; Efron, Yund, Nichols, & Crandall, 1985; Musiek et al., 2005). In fact, recent research has shown the GIN test to be highly sensitive and specific to neurologically based lesions of the CANS and can be accurately used across different languages (see Filippini, Wong, Schochat, & Musiek, 2019).
Temporal integration is not a commonly used test procedure for mass and vascular involvement of the CANS, but in the authors’ opinion, it probably should be. This procedure requires the measurement of thresholds for long (500 ms) and short (20 ms) tones and the determination of the difference between these thresholds. This test procedure has been shown to be sensitive to CANS involvement secondary to vascular/mass lesions (Baru & Karaseva, 1972). However, Cranford, Stream, Rye, and Slade (1982) modified the test to include a frequency difference limen for short and long tones, which made the procedure even more sensitive to CANS problems. It is difficult to understand why this simple, straightforward procedure is not utilized more in clinical settings. To date, the available evidence suggests that the procedure is sensitive to cortical damage, but to our knowledge, no data are available on brainstem involvement.
Monaural Low Redundancy Speech Tests
Monaural low redundancy speech tests (often referred to as distorted speech tests) include speech stimuli such as filtered, compressed, or interrupted speech signals as well as speech signals presented in the presence of noise or other speech signals. Filtered (usually low-pass filtered) and compressed speech tests have been shown to yield contralateral ear deficits for individuals with mass and vascular auditory cortex lesions. However, as a group, these procedures are not as sensitive to cortical lesions as the tests discussed previously (Bocca et al., 1954; Karlsson & Rosenhall, 1995; Lynn & Gilroy, 1972, 1975; see also Baran & Musiek, 1999, for review).
Monaural low redundancy speech procedures are not highly dependent on interhemispheric transfer; therefore, even individuals with split brains typically do well on these tests (Musiek, Kibbe, & Baran, 1984). For brainstem involvement, speech-in-noise and the synthetic sentence identification with ipsilateral competing message (SSI-ICM) tests have been shown to be sensitive to a variety of central lesions, including mass and vascular disorders (Jerger & Jerger, 1974; Morales-Garcia & Poole, 1972; Rintelmann, 1985). In addition, filtered speech, interrupted speech, and compressed speech tests have been shown to statistically separate control groups from patients with confirmed vascular lesions of the brainstem (Karlsson & Rosenhall, 1995; see also Musiek & Chermak, 2015).
Binaural Interaction Tests
Auditory tasks that require functional interrelationships between the two ears that are not the classically defined dichotic speech tests fit into the category of binaural interaction tests. Masking level differences (MLDs), interaural timing tests, localization and lateralization tasks, as well as spatial perception of speech in noise are the primary test procedures in this test category. These tests require fine acoustic comparisons for different stimuli between the ears (see Cameron & Dillon, 2007; Musiek & Chermak, 2014). The auditory analysis needed to occur is for that of time, intensity, and/or phase cues. The use of these tests has been directed primarily toward assessment of brainstem functions, although the use of localization and lateralization tasks are also sensitive to cerebral dysfunctions.
The classic localization study on cortical lesions with primarily, but not exclusively, vascular etiology was conducted by Sanchez-Longo, Forster, and Auth (1957). These investigators used a simple bedside localization task and found deficits in the auditory field opposite the involved hemisphere. This finding also has been documented in a subsequent localization study (Cranford, Diamond, Ravizza, & Whitfield, 1971). In addition, Pinheiro and Tobin (1969) reported asymmetric lateralization findings in stroke patients for a clinically oriented task utilizing interaural intensity differences.
The MLD procedure has a long clinical history. In a mixed clinical population of both mass and vascular lesions of the brainstem, Lynn, Gilroy, Taylor, and Leiser (1981) showed high sensitivity and specificity for the MLD using consonants-vowels (CVs) as stimuli. This classic study also demonstrated that individuals with midbrain and cortical lesions revealed MLDs similar to those demonstrated by their control group.
Auditory Brainstem Response
Early on in its history, the auditory brainstem response (ABR) was shown to be sensitive to mass and vascular lesions of the brainstem (Starr & Achor, 1975). Subsequent reports have supported this early finding with larger numbers of patients. Sensitivity and specificity have been in the 80% range with some variability noted in the reports (Chiappa, 1988; Karlsson & Rosenhall, 1995; Musiek & Lee, 1995). The standard one-channel electrode array is sufficient to obtain these kinds of sensitivity and specificity rates. Waves III through V are generated by pontine auditory structures; hence, latency delays or the absence of these waves (especially with a normal wave I) likely indicate brainstem involvement as does the observation of an extended III–V interwave latency. However, a III–V extension could also indicate auditory nerve dysfunction, but this is unlikely. Amplitude measures have not been used widely with ABR to identify abnormal brainstem function as amplitude measures tend to be quite variable and not particularly sensitive to brainstem lesions. On the other hand, absolute and interwave latency measures have been shown to be much more sensitive and specific and tend to be used more commonly for diagnostic purposes. Mass or vascular lesions that are intra-axial often result in very high hit rates for ABR (Musiek, 1991).
Mass and vascular lesions of the thalamocortical tracts and auditory cortices are best assessed by the middle latency response (MLR) and the late potentials (N1, P2, P3), respectively. However, when entertaining the possibility of brainstem involvement, the auditory brainstem response (ABR) is the evoked potential of choice, but it is of little value for assessing lesions rostral to the pons.
Middle Latency Response
The middle latency response (MLR) has been shown to be relatively sensitive to lesions of the auditory cortex and thalamocortical connections. Hit rates in the mid-70% range or better have been reported (Musiek, Charette, Kelly, Lee, & Musiek, 1999; Musiek & Nagle, 2018; Scherg & von Cramon, 1986). When assessing the MLR, it is most useful to use electrodes positioned in midline (Cz) and lateral positions relative to the auditory cortex (e.g., C3, C4, or T3, T4) as this allows electrode comparisons from each side of the head (i.e., intrasubject comparisons). However, this may also be performed with fewer electrodes if the clinician is limited to a two-channel system. Usually, the electrode positioned closest to the lesion site will show a compromised response (i.e., an electrode effect) when the MLR is assessed; hence, the importance of using the type of electrode placement recommended previously when assessing patients at risk for auditory cortex or thalamocortical pathway compromise. It is clear that amplitude measures are better diagnostically than latency measures for the MLR and that this MLR measure benefits from the type of intrasubject comparison described previously (Kileny, Paccioretti, & Wilson, 1987; Musiek et al., 1999). Significant amplitude differences for hemispheric comparisons are a common finding with unilateral cerebral lesions, and investigators have demonstrated that the MLR results in the best sensitivity measures when measures such as the electrode effect with amplitudes are applied (Musiek et al., 1999; Scherg & von Cramon, 1986).
The MLR can be recorded simultaneously with the ABR, which can be a major advantage in diagnostic utility. Under these testing conditions, the ABR can readily serve as a benchmark for the MLR waves, indicating integrity of the more caudal auditory nervous system. However, the clinician may find it to beneficial to record the ABR separately as subtle abnormalities in the ABR waveform may not be detected when it is combined with the MLR.
Late Responses (N1, P2)
The N1 and P2 auditory evoked potentials are generated primarily from the auditory cortex (see Musiek & Lee, 1999, for review). In vascular and mass lesions, these potentials can be compromised by changes in their amplitude as well as latency. Electrodes should be placed laterally as was described previously for the MLR. A study on patients with left hemisphere strokes interestingly demonstrated reduced N1 amplitudes over both hemispheres for right but not for left ear stimulation (Ilvonen et al., 2001). The N1 also has been shown to have good differential capability. Studies have shown the N1 to be reduced in temporal lobe strokes significantly more often than when the parietal or frontal lobes are similarly affected (Knight, Hillyard, Woods, & Neville, 1980; Knight, Scabini, Woods, & Clayworth, 1988). As with the MLR, N1, P2 responses are generally smaller in amplitude for the electrode that is near the lesion site. In addition, the latency of the response for the electrode nearer the site of lesion is likely to be delayed compared to that observed for the other hemispheric electrode (see Musiek & Lee, 1999, for review).
P300 Response
The P300 generators are not well known, but it seems logical that there are many throughout the brain. Auditory regions of the temporal cortex play a role, as probably do the frontal lobe and the hippocampus (see Musiek & Lee, 1999). The P300 does not appear to provide distinct laterality information like the MLR and N1, P2. This may be because of the multiple generator sites for the P300 response (Musiek, Baran, & Pinheiro, 1992). The P300 is highly sensitive to aging and dementia. It is also sensitive to lesions of the auditory cortical areas of the brain. Both amplitude and latency effects are noted for P300s in individuals with mass and/or vascular lesions (Musiek et al., 1992; Obert & Cranford, 1990). The P300 has been shown to differentiate parietal from temporoparietal lesions with the latter lesions severely compromising latency and amplitude measures (Knight, Scabini, Woods, & Clayworth, 1989). The oddball paradigm that is used to acquire the P300 is a powerful approach and allows the simultaneous recording of the N1, P2 and P300 evoked potentials. The electrode arrangement for the P300 classically is midline anterior to posterior (Fz, Cz, Pz). However, because the N1 and P2 can be recorded with the P300 electrodes in lateral positions (C3, C4, and/or T3, T4), the use of these types of electrode arrays could prove useful.
History
This patient was a young adult who developed seizures secondary to a temporal lobe tumor. She reported left arm numbness for years and difficulty with motor movements on the left side. In addition, she complained of light-headedness and often feeling as if she was about to pass out. She stated that the hearing in her left ear was worse than that in her right ear, yet hearing sensitivity was better in her left ear on the audiogram.
Audiology
The patient had normal hearing sensitivity for pure tones in the left ear and a very mild conductive loss for the right ear with excellent speech recognition bilaterally. Central auditory behavioral tests showed rather marked left ear deficits for dichotic listening tests (digits and rhyme tests) and a bilateral deficit for duration patterns (Figure 7–1A).
Figure 7–1. Central behavioral tests results (A) and computerized tomography (CT) scan (B) for a young adult with a right temporal lobe tumor (Case 7–1).
Note the temporal lobe tumor at 8 o’clock.
Medical Examination
Neurologically, there was documentation of a seizure disorder. Radiologically, a CT scan showed a right posterior temporal lobe tumor that can readily be seen in Figure 7–1B. This tumor was approximately 2 × 2 × 4 cm in size. Note how the tumor has affected the right lateral ventricle by compressing it (see Figure 7–1B).
Impression
This patient has central auditory involvement secondary to a right temporal lobe tumor and the patient’s related subjective complaints of hearing difficulty for the left ear. A very mild conductive loss is present in the right ear. Interestingly, the left ear (i.e., contralateral to the involved hemisphere) was reported by the patient as being poorer than the right ear (i.e., the ear with the mild conductive loss). These central test findings are consistent with expectations for a right posterior temporal lobe lesion in that they revealed a classic contralateral ear effect.
Case 7–2: Temporoparietal Stroke
History
At the time of evaluation, this middle-aged woman was several months post right temporoparietal stroke. She was bothered by dizziness episodes, difficulty hearing soft voices, and difficulty hearing in the presence of background noise to the point that audiologic consultation was sought.
Audiology
This patient’s pure-tone thresholds were normal and her speech recognition ability was excellent bilaterally. Behavioral central auditory test results showed the classic contralateral effect on dichotic speech tests and a bilateral deficit on frequency patterns (Figure 7–2A). Central auditory electrophysiologic tests included ABR-MLR testing performed simultaneously using a click stimulus (Figure 7–2B). The ABR was normal bilaterally, whereas the MLR showed an ear effect with the right ear Pa responses essentially present at all electrode sites and the left ear showing an absent Pa response with a meager Na that, if present, would be delayed at all recording sites.
The ENG test results for this patient showed some mild smooth pursuit tracking abnormalities and a stronger caloric response for the right side that did not reach clinical significance. The CT scan revealed a lesion site in the right hemisphere (temporoparietal area) (see arrows in Figures 7–2C and 7–2D).
Impression
Central auditory involvement consistent with a right temporoparietal stroke.
Medical Examination and Management
This patient was being followed and managed neurologically poststroke. She also had weakness in the left extremities but was ambulatory.
Audiologic Management
At the time of her evaluation, the patient was counseled on the use of assistive listening devices, enhancing the listening environment, and home auditory training techniques and arrangements were made for follow-up and consultations closer to her home.
A variety of degenerative disorders can compromise the CANS. However, in this chapter, the focus is on two relatively common degenerative disorders that can affect the CANS: multiple sclerosis (MS) and Alzheimer’s disease (AD). Multiple sclerosis is a chronic, progressive autoimmune disease that results in myelin destruction, which primarily affects young adults. The severity is highly variable, and some patients have prolonged periods of total remission. Patients with MS can have flares (i.e., transient episodes of focal demyelination) that can last days to weeks. Thus, the symptoms can be relapsing and remitting over time, but eventually an overall progressive decline is typically observed. Because it is a disease affecting the myelin sheathing of nerve fibers, MS can affect any motor or sensory system or region of the nervous system that has myelinated nerves. Of note is the fact that only a portion (medial) of the auditory nerve is myelinated. Therefore, this disease more commonly involves the CANS rather than the peripheral auditory system.
Figure 7–2. Central behavioral test results (A), combined ABR-MLR tracings (B), and computerized tomography (CT) scans (C and D) for a middle-aged woman with a right-sided temporoparietal stroke (Case 7–2). Key for (B): V = ABR wave V; Pa = MLR wave; Cz, C3, C4 = electrode sites.
Alzheimer’s disease (AD) is the most common cause of dementia and is classified as a degenerative cognitive disorder, but it also affects other systems in the central nervous system. Generally, older adults are affected by the disease, but it can attack middle-aged individuals as well. There is a tendency for the disease to run in families (see Zabar, 2005).
In MS, the symptoms are related to the locus of the disease. That is, wherever the myelin is attacked and plaques (a type of scarring of myelin) form, symptoms related to that area of the brain can emerge. As mentioned previously, the motor and sensory symptoms associated with MS can come and go. When the symptoms become worse, it is called an exacerbation. This situation is accompanied by swelling around the plaque(s) and a related increase in dysfunction. All neural systems can be affected. Auditory symptoms are often overlooked by both the patient and the clinician because they tend to be more subtle than other motor or sensory symptoms. Initial onset is usually in the 20- to 40-year-old age range and common symptoms include paresthesia, motor weakness, diplopia, blurred vision, ataxia, vertigo, balance disturbances, and hearing difficulties (Jerger & Jerger, 1981; Merritt & Antunes, 1979).
In patients with AD, a variety of symptoms emerge, and in the early stages of this neurodegenerative disease process, it is quite likely that the symptoms may go unnoticed. However, in time, short-term memory loss, loss of organizational skills, decreased language function, the need for frequent repetition of conversational statements and verbal requests, impaired visual spatial skills, and hearing difficulties will become obvious and progress to the point where the patient requires significant care (see Zabar, 2005).
It is interesting to note that MS is more common in cold as opposed to warm geographic regions. For example, in Southern states, the incidence is 6 to 14 per 100,000, whereas in Northern states, it is 40 to 60 per 100,000. MS is not found in native-born Africans and is rare in the populations of Japan, Taiwan, and India. It is more common in women than men, and the mean age of onset is usually between 20 to 30 years of age (Poser, 1979).
Alzheimer’s disease is present in about 50% of the population over 85 years of age. There are about 4 million cases in the United States and the risk of developing the disease doubles every 5 years after age 65 (Zabar, 2005).
The etiology for MS is unknown but results in the demyelination of nerves (with secondary axonal degeneration occurring as a result). MS is classified as an autoimmune disease, and it appears that lymphocytes and macrophages play a role in the disease process by attacking myelin (Calabresi, 2006). Demyelination due to inflammatory plaques results in reduced and/or delayed neural impulses. In turn, this affects the specific system that is involved. The brain is capable of repairing areas of demyelination, which is why periods of exacerbation and remission (i.e., a period of time when the symptoms are not manifested) are noted in many patients with MS, especially those in the earlier stages of this disease. However, if the inflammation is severe, recurrent, or prolonged, it can cause axonal damage that becomes permanent.
The exact etiology of AD is not known. However, a common finding with this disease is an excess of beta-amyloid protein, which can impair synaptic function. It appears that the excess of beta-amyloid protein is a factor in the development of amyloid plaques, which are commonly seen in brains affected by AD. However, it is unclear if beta-amyloid alone is enough to trigger AD. Inflammation and free radical damage also appear to play a role. One hallmark of AD is the presence of neuronal inclusions called neurofibrillary tangles that contain tau, a protein that can cause cell damage. Another factor in AD is the reduction in acetylcholine, which is observed in patients with AD and may be related to some of the conditions mentioned previously (Zabar, 2005).
Risk factors associated with AD include age, family history, hypertension, stroke, diabetes, and head injury (Zabar, 2005). In addition, patients with Down syndrome also commonly develop AD, with the onset of the degenerative processes frequently occurring in these individuals when they are in their 40s (Zabar, 2005).
In MS, there is not a relationship between the disease and any particular nerve tract. Because MS is a disease affecting myelin, it will often be found where there is an abundance of white matter, such as in the corpus callosum, the medial longitudinal fasciculus, and a periventricular region of the brain referred to as the trigone (Muriello, Jones, & Chaves, 2005; Rubens, Froehling, Slater, & Anderson, 1985).
In AD, the frontal, temporal, and parietal lobes often are the first structures to become involved. However, with time, the entire cerebrum eventually undergoes degenerative processes. When this cortical degeneration occurs, the gyri become thin, the sulci become large, and the subcortical areas also degenerate (Zabar, 2005).
In MS, a neurologic history will check for intermittent and relapsing symptoms, such as ocular, vestibular and/or auditory problems, “electric shock” sensations, numbness, tingling, weakness, motor problems, and fatigue. Several tests are used to make the diagnosis including MRI (to visualize plaques), lumbar puncture, and evoked potentials. Usually, evidence of lesions in at least two areas of the central nervous system and a minimum of two separate neurologic episodes must have occurred for the diagnosis to be made (Muriello et al., 2005).
There are no definitive tests for AD. However, careful evaluation of mental status is critical. Trends of cognitive impairment and decline are important to document. Neuropsychologic studies in regard to cognitive (reasoning) and memory functions are central in the evaluation of AD. In advanced AD, CT or MRI can show cortical atrophy. In addition, other studies such as positron emission tomography (PET), cerebrospinal fluid analysis for beta-amyloid and tau, single photon emission computed tomography (SPECT), functional MRI (fMRI), electroencephalography (EEG), and P300 evoked potentials occasionally are used for diagnosis (Musiek et al., 1994; Zabar, 2005).
In the treatment of MS, pharmaceutical management is the main course. The mainstay of MS therapy is treatment with injectable interferon-beta or glatiramer acetate, which has been shown to be effective in reducing exacerbations. Several new drugs that modulate the immune system are becoming available. In severe cases, azathioprine, cyclosporine, cyclophosphamide, and methotrexate have been recommended (Muriello et al., 2005).
Alzheimer’s disease is managed by initiating good all-around health habits such as exercise, good nutrition, and social activities. Current medications such as Aricept, Exelon, and Razadyne seem to focus on arresting the breakdown of acetylcholine. These medications are not a cure for AD, but there are some indications of improved mental function for patients taking these drugs (WebMD, 2005–2019).
A wide variety of degenerative disorders can affect the CANS. However, in this section of this chapter, we focus on MS and AD. It is important to realize that hearing difficulties occur much more often in patients with MS than was originally believed (Jerger, Oliver, Chmiel, & Rivera, 1986). Musiek, Gollegly, Kibbe, and Reeves (1989) reported that approximately 40% of the patients with MS in their study had some type of hearing complaint, but only 18% had abnormal pure-tone audiograms. In another study, Luxon (1980) showed abnormal audiograms in over 50% of the MS patients she studied.
Although not a common occurrence, sometimes hearing difficulties can be the first sign of MS. Usually, the hearing problems are subtle and become more obvious in noisy listening situations (Luxon, 1980; Musiek et al., 1989). Tinnitus and vestibular symptoms can also be experienced by patients with MS.
Alzheimer’s disease has come to the forefront of audiology in the past 10 years or so due to the interesting findings that have been reported by George Gates. Gates and his colleagues have demonstrated that central auditory test results are often abnormal in patients with AD compared to matched controls (Gates, Anderson, Feeney, McCurry, & Larson, 2008). In addition, it appears that poor performance on central auditory tests may precede the onset of AD, or at least the diagnosis of AD. This in turn has raised the question that perhaps central auditory testing may have some predictive value in regard to AD. Based on their findings, Gates and colleagues (2008) have argued strongly for including central auditory tests in the hearing evaluation of the elderly.
Behavioral Test Procedures in MS
Dichotic Listening Tests. There is a generous amount of literature pertaining to MS and dichotic listening. It is well known that dichotic listening relies on normal function of the corpus callosum, a structure that is highly myelinated. As myelin is the target of MS, this is a likely locus for the manifestation of the disease. Both recent as well as early reports support the claim that the corpus callosum is a common lesion site in MS, as do studies that document the classic finding of left ear deficits on dichotic listening in patients with MS (Gadea et al., 2009; Rubens et al., 1985). The left ear deficit can be variable and depends on the amount and locus of the interhemispheric neural disruption caused by the MS plaques. Dichotic listening to speech stimuli is one of the best procedures for detecting auditory dysfunction in MS (Gadea et al., 2009; Musiek et al., 1989).
Temporal Processing Tests. Temporal processing tests have not been utilized as much as dichotic speech tests in MS. At best, auditory pattern perception and gap detection procedures have revealed only moderate sensitivity for detecting dysfunction related to MS (Hendler, Squires, & Emmerich, 1990; Musiek et al., 1989; Musiek & Weihing, 2011). However, recent research has shown these temporal processing tests to be of significant value in defining auditory dysfunction in MS patients (Valadbeigi et al., 2014). Given these findings, it appears more testing of temporal processing procedures needs to be completed before a final determination regarding the value of these tests in the assessment of central auditory function in MS patients can be made.
Monaural Low Redundancy Speech Tests. Low redundancy speech tests such as filtered speech have not shown particularly notable hit rates for identifying auditory involvement in patients with MS (Musiek et al., 1989). However, in a study evaluating a mixed population of patients with MS and brainstem tumors, Karlsson and Rosenhall (1995) demonstrated that both filtered and compressed speech tests separated the involved group from a group of normal controls. They also noted a greater deficit for the ear ipsilateral to the lesion site than for the contralateral ear in the patients with brainstem involvement. In another study, Jerger and his colleagues (1986) found that the synthetic sentence identification with ipsilateral competing message (SSI-ICM) was moderately sensitive to auditory involvement related to MS.
Binaural Interaction Tests. Two binaural interaction tests will be mentioned here: the MLD test and an interaural timing task. The MLD procedure has a long history of use in patients with MS and has been shown to be reasonably sensitive to central auditory involvement (see Hendler et al., 1990; Jerger et al., 1986; and Musiek et al., 1989). Although both speech and tonal stimuli can be used, low-frequency tonal stimuli (usually 500 Hz) have been more commonly utilized with patients with MS. Interaural timing tasks also have been shown to be highly sensitive to auditory involvement from MS. In fact, this procedure has been shown to be even more sensitive than the ABR in MS (Levine et al., 1994). Unfortunately, this procedure has not made its way into common clinical use.
Electrophysiologic Test Procedures in MS
Auditory Brainstem Response. Perhaps the most utilized audiologic test in the MS population is the ABR. Hit rates vary widely because without detailed radiology, one cannot be sure if the auditory tracts are involved. When the brainstem auditory tracts are involved, the ABR is abnormal an extremely high percentage of the time (Levine et al., 1994). In the general MS population, ABR sensitivity hovers around 60%; however, this figure is probably somewhat artificially low as many individuals with MS do not have auditory involvement (Musiek, 1991). Compared to other central auditory tests, the ABR is one of the most sensitive measures, if not the most sensitive measure, for auditory dysfunction in patients with MS (Jerger et al., 1986, Musiek et al., 1989). When combined with other tests, however, this procedure can yield an even higher hit rate (Musiek et al., 1989). The present authors posit that the combination of an ABR and a dichotic listening procedure is the best test battery for MS as these tests assess the brainstem pathways as well as the corpus callosum, two regions of the auditory system often involved in MS.
Middle Latency Response. The MLR has been shown to be sensitive to auditory dysfunction related to MS. In fact, a 73% hit rate was reported for MS patients in a rather extensive study (Çelebisoy, Aydoğdu, Ekmekçi, & Akürekli, 1996). The MLR can be easily combined with the ABR and can yield many clinical advantages. By combining the ABR and the MLR, a hit rate of 80% was noted for a group of patients with MS (Japaridze, Shakarishvili, & Kavenishvili, 2002). Although more studies need to be completed, it appears that the MLR may be a valuable procedure to use in evaluating central auditory integrity in patients with MS, especially if the MLR is combined with the ABR.
Late Potentials (N1, P2, P300). The N1, P2 waveform complex has had limited use in the evaluation of patients with MS. The studies that were available for review showed a relatively modest hit rate, which would make one ponder the utility of these potentials with this population (Boose & Cranford, 1996; Japaridze et al., 2002). However, the P300 has shown significantly delayed latencies, reduced amplitudes, and even a moderate incidence of absent waveforms for MS patients compared to control groups (Boose & Cranford, 1996; Honig, Ramsay, & Sheremata, 1992; Polich, Romine, Sipe, Aung, & Dalessio, 1992). The key question surrounding these studies is whether the P300 abnormality is related to cognitive decline, delayed (auditory) neural conduction time, or both of these conditions. It may be efficient to record both the N1, P2 wave and P300 simultaneously using a standard oddball paradigm and to compare the results of these auditory evoked potentials as such a procedure would not significantly increase the testing time and could provide additional data for comparison and analysis.
Behavioral Test Procedures in AD
Dichotic Listening Tests. A number of studies have used the dichotic digits test to measure central auditory dysfunction in patients with AD (Gates et al., 2008; Grimes, Grady, Foster, Sunderland, & Petronas, 1985; Strouse, Hall, & Burger, 1995). Some of these studies have demonstrated significant differences between patients with AD and controls, with the AD population revealing poorer scores for both ears, whereas others have documented asymmetric performance with poorer left ear scores when compared to right ear scores in patients with AD. The dichotic sentence identification (DSI) and staggered spondaic word (SSW) tests also have shown deficits for the AD population compared to control groups (Cooper & Gates, 1991; Gates et al., 2008, Strouse et al., 1995). Given these types of findings, dichotic listening has been considered a useful procedure in evaluating auditory system integrity in patients with AD (Gates et al., 2008; Iliadou & Kaprinis, 2003).
Temporal Processing Tests. Strouse and colleagues (1995) examined the performance of patients with AD on pattern recognition tasks. The results of this study demonstrated that the duration pattern test clearly separated patients with AD from matched controls (i.e., there was a mean difference of more than 30% between the two groups when the test was presented binaurally), indicating possible auditory temporal processing deficits in the study’s participants with AD. Interestingly, the frequency pattern test did not yield significant differences between the patients with AD and controls in this same study. This finding points to basic differences between these two similar tests of temporal ordering, indicating that the two tests likely are assessing different underlying processes and therefore should not be used interchangeably. To date, there has been limited investigation of the application of temporal processing tests in the assessment of auditory processing in patients with AD and additional investigation in this area is warranted.
Monaural Low Redundancy Speech Tests. The Synthetic Sentence Identification with Ipsilateral Competing Message (SSI-ICM) was one of the early tests employed in measuring central auditory function in AD. This test revealed lower scores for patients with AD when compared to a control group (see Iliadou & Kaprinis, 2003). It also has been demonstrated that signal-to-competition ratios proved to be more diagnostically sensitive as greater group differences were noted when the test was administered at poorer signal-to-noise ratios than at more favorable ratios (Strouse et al., 1995).
Binaural Interaction Tests. There is some evidence that binaural interaction processes may be compromised in AD. One study clearly showed a deficit in sound localization for patients with AD compared to controls (Kurylo, Corkin, Allard, Zattore, & Growdon, 1993). This suggests that interaural timing and resolution tests may have applications in the assessment of patients with AD.
Electrophysiologic Test Prodedures in AD
Auditory Brainstem Response. As might be expected, AD seems to have little effect on the ABR. However, limited data are available in this area. In one study, Irimajiri, Golob, and Starr (2005) showed no shift in wave V latency of the ABR for patients with AD compared to normal individuals.
Middle Latency Response. As was the case with the ABR, few data are available on the results of MLR testing in patients with AD. Based on the limited data available, AD appears to have little influence on the Na, Pa waves, but possibly some abnormalities in the later wave (P1) of the MLR (Green, Elder, & Freed, 1995; Irimajiri et al., 2005).
Late Potentials (N1, P2, P300). For many years and in many studies, the P300 has been shown to be abnormal in many patients with AD (see the following discussion), but there are much less compelling data for the N1 and P2 waves. The N1 wave of the N1–P2 complex has been shown to be smaller in subjects with AD when compared to controls (Green et al., 1995; St. Clair, Blackwood, & Christie, 1985). However, there does not appear to be any influence on the latencies of these waves. The P2 also has been shown to be smaller in amplitude in patients with AD (St. Claire et al., 1985).
The P300 has demonstrated increased latencies and decreased amplitudes in patients diagnosed with AD (Polich & Corey-Bloom, 2005; St. Clair et al., 1995; and others). Although the P300 is clearly and systematically affected by age, it can still separate patients with AD from the normal aging population, and thus it can be a valuable tool in evaluating AD.
History
A 38-year-old male presented with a long-standing history of MS. He had general extremity weakness, blurred vision, ataxia, and slurred speech. He also had long-standing tinnitus on the right side and possible hearing difficulty. The clinical question posed by the patient was whether or not the auditory system was involved, and if it was, whether it was related to his diagnosed medical condition?
Audiology
This patient demonstrated normal puretone thresholds and excellent speech recognition ability bilaterally. His tympanograms showed normal pressure, volume, and compliance for both ears. Contralateral acoustic reflexes were present at normal levels for the left ear but were absent for the right ear for the frequencies tested (500 to 2000 Hz). Central auditory behavioral test results showed a left ear deficit on dichotic digits, bilateral deficits on frequency patterns, and normal performance for both ears on the filtered word test, which is a common set of findings for people with MS, likely due to corpus callosum involvement (Figure 7–3A). A bilateral MLD for a 500 Hz tonal stimulus was 4 dB, which was abnormal (see Figure 7–3A). The ABR results demonstrated the presence of the early waves (I and II), but essentially abnormal or absent later waves (III and V) bilaterally (Figure 7–3B). Low-repetition rate tracings (top tracings for each ear) as well as high-repetition rate tracings (bottom tracings for each ear) were of similar poor morphology. Interestingly, although the ABR was abnormal bilaterally, not all of the behavioral tests were, indicating a different underlying physiology for the various tests (see Musiek et al., 1994).
Impression
Central auditory involvement secondary to multiple sclerosis.
Medical Management
The patient was being followed medically by neurology for MS.
Audiologic Management
The patient was counseled about the findings, which indicated normal peripheral hearing and likely central auditory involvement. Listening strategies and environmental modifications (i.e., seeking seating with his right ear away from noise sources, a trial use of hearing aids to assist with his central auditory deficits) were recommended.
Figure 7–3. Central behavioral test results (A) and ABR tracings (B) for a young adult with a diagnosis of multiple sclerosis (Case 7–3).
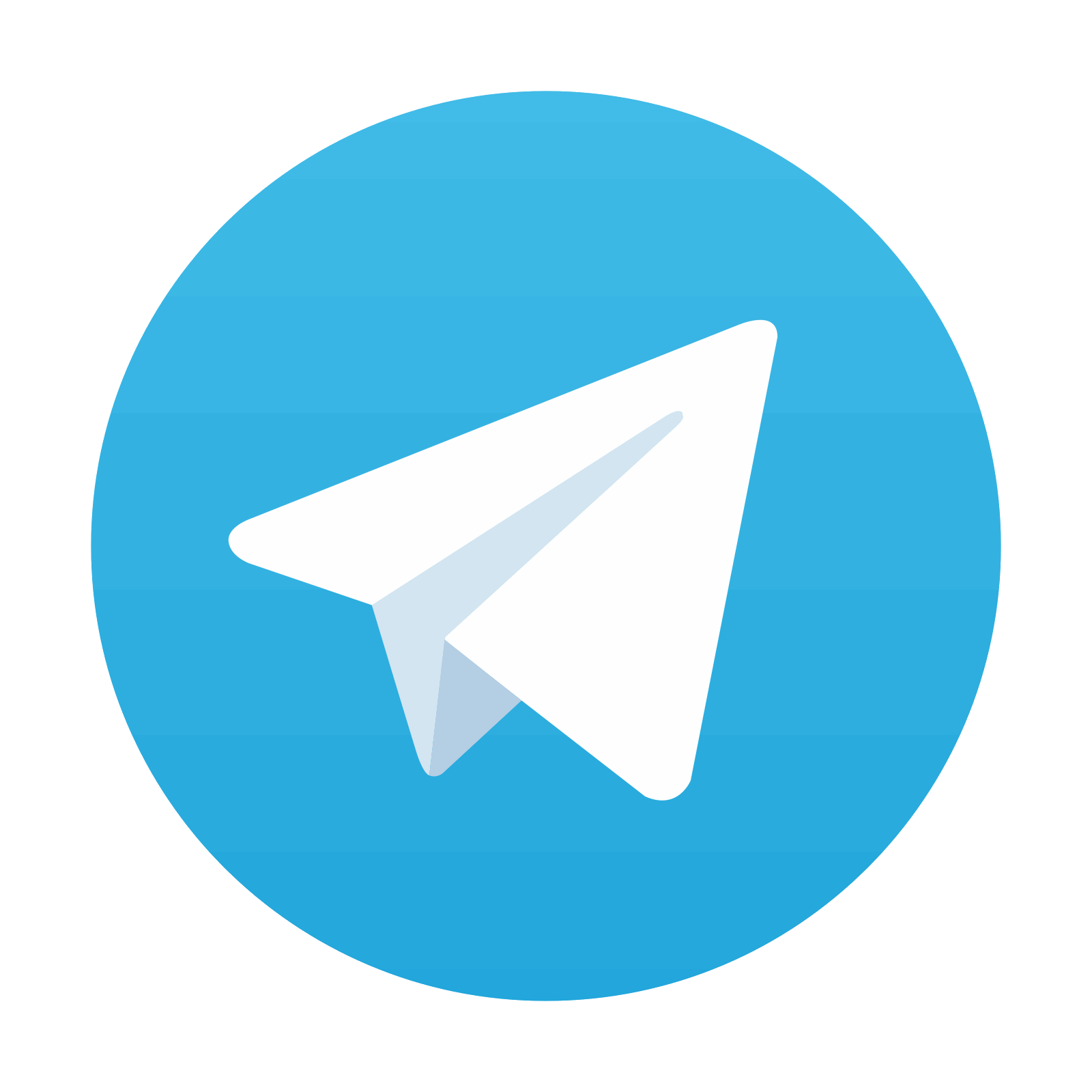
Stay updated, free articles. Join our Telegram channel

Full access? Get Clinical Tree
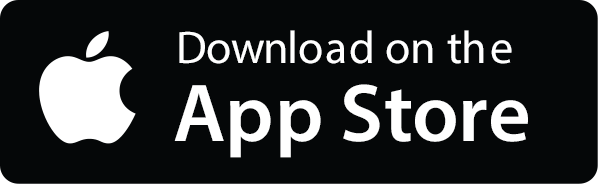
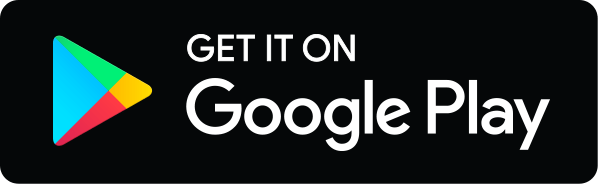