Purpose
To evaluate the effect of uncomplicated cataract phacoemulsification on the measurements of visual evoked potentials (VEP), pattern electroretinogram (PERG), and macular and retinal nerve fiber layer (RNFL) using 2 spectral-domain optical coherence tomography (OCT) instruments, the Cirrus OCT (Carl Zeiss Meditech) and Spectralis OCT (Heidelberg Engineering), in patients with retinitis pigmentosa (RP), and to assess the reliability of the OCT measurements before and after cataract surgery.
Design
Observational cross-sectional study.
Methods
Thirty-five eyes of 35 patients with RP (20 men and 15 women, 45-66 years) who underwent cataract phacoemulsification were studied. At 1 month before and 1 month after surgery, visual acuity, VEP, PERG, and 3 repetitions of scans using the RNFL and macular analysis protocols of the Cirrus and Spectralis OCT instruments were performed. The differences in measurements between the 2 visits were analyzed. Repeatability of OCT measurements was evaluated by calculating the coefficients of variation.
Results
VEP amplitude, RNFL thicknesses provided by Cirrus and Spectralis, and macular measurements provided by Cirrus OCT differed between the 2 visits. VEP latency, PERG measurements, and macular thicknesses provided by the Spectralis OCT before surgery did not differ significantly from those after surgery. The OCT repeatability was better after surgery, with lower coefficients of variation for scans performed after surgical removal of the cataract. The nuclear, cortical, and posterior subcapsular types of cataracts did not show different repeatability.
Conclusions
The presence of cataracts affects VEP amplitude, RNFL, and macular measurements performed with OCT in eyes with RP. Image repeatability significantly improves after cataract phacoemulsification.
Retinitis pigmentosa (RP) refers to a heterogeneous group of genetic retinal disorders primarily affecting the rod and cone photoreceptors and the retinal pigment epithelium cells. RP is a major cause of blindness and the most commonly inherited form of severe retinal degeneration, with a prevalence of about 1 in every 3500 births. Several genes are associated with RP, which can be inherited as an X-linked, autosomal dominant or autosomal recessive condition. Cases with no known family history, referred to as RP simplex, also occur.
Optical coherence tomography (OCT) is a well-recognized method of analyzing the in vivo retinal architecture. OCT is used for diagnosing and following retinal diseases, and is particularly useful and accurate for measuring retinal thickness. Several OCT studies of RP have been reported, most of which show the ability of OCT to recognize and follow retinal changes in RP patients. RP predisposes patients to cystoid macular edema, macular holes, epiretinal membranes, posterior subcapsular cataracts, pigmented flecks, and syneresis of the anterior vitreous. Noninvasive scanning using OCT yields valuable quantitative data related to the retinal nerve fiber layer (RNFL) and macular thickness. The clinical utility of OCT is well established.
Although good repeatability of OCT data has been described, OCT measurements are significantly affected by the OCT image quality. Factors such as the presence of cataracts, extent of pupillary dilation, and corneal dryness affect both image quality and the repeatability of subsequent measurements. Cataracts may affect diagnostic imaging quality in OCT and cause artifacts in images. OCT is an important ophthalmic diagnostic tool, particularly in retinal diseases such as RP. OCT imaging relies on near-infrared light. Similar to fundus photography and scanning laser ophthalmoscopy, cataracts are likely to increase light scattering in OCT, thereby degrading the image quality. Some authors have reported variations in OCT measurements before and after uncomplicated cataract surgery, but these studies included short series of healthy subjects and were focused on the slight increase in macular thickness after surgery and on an apparent increase in the RNFL thickness attributable to improved image quality. To the best of our knowledge, there are no reports comparing repeatability before and after cataract surgery using the Spectralis OCT (Heidelberg Engineering, Carlsbad, California, USA). Most of previous publications analyzing the effects of cataract surgery used the Stratus time-domain and Cirrus OCT (Carl Zeiss Meditech, Dublin, California, USA).
Repeatability and reproducibility are important for distinguishing normal subjects from patients with disease as well as for following retinal or RNFL modifications over time, aimed at measuring changes after treatment.
Neurophysiologic techniques are useful for evaluating the transmission characteristics of information within the visual system and provide the magnitude and latency of responses, which in turn reflect axonal and myelin integrity. The magnitude of the visual evoked potential (VEP) depends on the functional afferent fibers reaching the striate cortex. Afferent fibers from the optic nerve reach the lateral geniculate nucleus and indirectly affect synaptic activity in the striate cortex. The pattern electroretinogram (PERG) is a retinal biopotential evoked when a temporally modulated patterned stimulus of constant total luminance is viewed. The PERG may be altered by selective dysfunction of the macula or of the inner retina, which does not significantly affect the conventional full-field ERG.
In the present study, we assessed the influence of cataracts on macular and RNFL thicknesses provided by Spectralis and Cirrus OCT scans, VEP, and PERG, in patients with RP. We also evaluated the intra-operator reproducibility of OCT measurements in RP patients before and after cataract extraction. The main objectives of this study were to evaluate how cataracts affect OCT, VEP, and PERG repeatability and the changes observed in these measurements after surgery.
Methods
This was an observational, prospective, longitudinal study. The study and data accumulation were performed with approval from the Miguel Servet Hospital Institutional Review Board (IRB). The authors confirm that the study and data accumulation conformed to all country, federal, or state laws, and the study adhered to the tenets of the Declaration of Helsinki. Informed consent for the research was obtained from the patients or subjects.
Thirty-five patients with a diagnosis of RP and cataract were enrolled in the study. The diagnosis of RP was based on a history of night blindness, impairment in peripheral visual fields, reduction of electroretinogram rod and cone amplitudes, and the presence of characteristic fundus pigmentary changes. Only RP eyes with a best-corrected visual acuity (BCVA) of 0.1 or better on the Snellen scale were included because eye fixation is required to complete the ophthalmologic and neurophysiologic exploration.
Exclusion criteria were the presence of significant refractive errors (>5 diopters of spherical equivalent refraction or 3 diopters of astigmatism); intraocular pressure of 21 mm Hg or higher; other causes of media opacifications; systemic conditions that could affect the visual system; history of ocular trauma or concomitant ocular diseases, including a previous history of optic neuritis and glaucoma; and laser therapy or ocular pathologies affecting the cornea, lens, or optic nerve.
All patients underwent a complete ophthalmologic and neurophysiologic evaluation that included pupillary and anterior segment examination, with evaluation of nuclear color/opalescence, cortical or posterior subcapsular lens opacity according to the Lens Opacities Classification System III system, fundoscopic examinations; assessment of BCVA relative to the Snellen scale; VEP, PERG, and 3 repetitions of scans using RNFL and macular cube 512 × 128 analysis protocols of the Cirrus OCT and the RNFL protocol of the Glaucoma application and Retinal Fast protocol of the Retinal application of the Spectralis OCT. All measurements were performed 1 month before and 1 month after cataract surgery. All patients underwent cataract surgery by the same surgeon using an Alcon Infiniti Phaco System with OZil available (Alcon Laboratories, Inc, Fort Worth, Texas, USA) and an AcrySof intraocular lens with blue-light-filtering technology (Alcon Laboratories, Inc, Fort Worth, Texas). Each eye was considered separately and only 1 eye of each subject was included in the study. The indication for cataract surgery was visual acuity below 0.6 on the Snellen scale or low enough to interfere with the patient’s life and daily activities or when no satisfactory visual function could be obtained with glasses, contact lenses, and other optical aids.
The OCT tests were performed to obtain measurements of peripapillary RNFL using the Cirrus and Spectralis OCT devices, both of which were used in random order to prevent any effect of fatigue bias. All scans were performed by the same experienced operator. Between scan acquisitions, there was a time delay and subject position and focus were randomly disrupted, meaning that alignment parameters had to be newly adjusted at the start of each image acquisition. No manual correction was applied to the OCT output. An internal fixation target was used because it provides the highest reproducibility. The quality of the scans was assessed prior to the analysis and poor-quality scans were rejected. The Cirrus OCT determines the quality of images using a signal strength measurement that combines the signal-to-noise ratio with the uniformity of the signal within a scan and is measured on a scale of 1-10, where 1 is categorized as poor image quality and 10 as excellent image quality. The Spectralis OCT uses a blue quality bar in the image to indicate signal strength. The quality score range is 0 (poor quality) to 40 (excellent quality). Only 2 patients were excluded because a centered scan could not be acquired on account of poor fixation. Sixteen images with artifacts, with missing parts, or showing seemingly distorted anatomy were excluded. To obtain good-quality and centered images, 5 eyes required repeat scan acquisition using the Cirrus OCT and 3 eyes using the Spectralis OCT.
Following the recommended procedure for scan acquisition, the subject’s pupil was first centered and focused in an iris viewing camera on the system data acquisition screen, and then the system’s line-scanning ophthalmoscope was used to optimize the view of the retina. The OCT scan was aligned to the proper depth and patient fixation, and system polarization was optimized to maximize the OCT signal.
Three repetitions of optic disc cube 200 × 200 and macular cube 512 × 128 scans in each eye were performed using the Cirrus OCT. In each series of scans, mean RNFL thickness and quadrant RNFL thickness (superior, inferior, temporal, and nasal) were analyzed.
Three image acquisitions with the RNFL protocol of the classic Glaucoma application and Fast Retina protocol of the Retinal application using Spectralis OCT were performed for all subjects using TruTrack eye-tracking technology. The mean number of scans required to produce each circular B-scan was 9. The RNFL protocol of the Spectralis generates a thickness map with mean thickness, 4 quadrants (superior, nasal, inferior, and temporal), and 6 sector thicknesses (superonasal, nasal, inferonasal, inferotemporal, temporal, and superotemporal, in the clockwise direction for the right eye and counterclockwise for the left eye). The presence of defects in the RNFL was determined by comparing the measurements from each patient with the normative database of each instrument.
Retinal thickness values were calculated with Cirrus and Spectralis OCT for the 9 areas corresponding to the Early Treatment Diabetic Retinopathy Study (ETDRS). The ETDRS areas include a central 1-mm circle, representing the foveal area, and inner and outer rings of 3 and 6 mm diameter, respectively. The inner and outer rings are divided into 4 quadrants: superior, nasal, inferior, and temporal. Central foveal thickness was also calculated.
VEPs were recorded with a Neuronic Sense Witness 4.0 machine (Neuronic, Zaragoza, Spain), in a dark room and with full correction of refraction if necessary. Electrodes were fixed with collodion in the midoccipital and midfrontal locations, with Cz used as ground. Stimulation was monocular after occlusion of the other eye, and visual stimuli followed a checkerboard pattern (contrast, 80%; check size of 30 min of visual angle, 47′; mean luminance, 93 cd/m 2 .). Patterns were reversed in contrast with a frequency of 1 Hz. The transient VEP response is characterized by a number of waves with 3 successive peaks of negative, positive, and negative polarity, respectively. In normal subjects, these peaks have latencies of 75, 100, and 145 ms (N75, P100, N145), respectively. Latency and peak-to-peak amplitude were recorded for the P100 wave. At least 2 records of 100 events each were obtained and the mean was calculated.
PERGs were obtained using a Neuronic Sense Witness 4.0 instrument (Neuronic) in line with International Society for Clinical Electrophysiology of Vision standards. Stimuli were checkerboard patterns with a check size of 30 min of visual angle (min arc). The contrast used was 90% and the mean luminance 93 cd/m 2 . The bioelectric signal was recorded through fiber corneal electrodes described by Dawson, Trick, and Litzkow (DTL). The DTL was draped horizontally across the cornea at the level of the lower lid. The reference electrode was positioned at the outer canthus and the ground at Fpz. Pattern reversals were applied at a frequency of 2 Hz. Stimulation was binocular. The waveform is characterized by a small initial negative component, at approximately 35 ms (N35), which is followed by a larger positive component (45-60 ms), typically denoted as P50. This is followed by a large negative component at about 90-100 ms (N95). The evaluated parameters were N95 and P50 amplitudes and latencies and the N95/P50 ratio.
Statistical analyses were performed using the Statistical Package for the Social Sciences (SPSS 19.0; SPSS Inc, Chicago, Illinois, USA). The Kolmogorov-Smirnov test was used to assess sample distribution. VEP, PERG, RNFL, and macular parameters were compared before and after cataract surgery using Student t test for paired data. P values less than .05 were considered statistically significant.
For each OCT parameter, the coefficient of variation (COV) was calculated as the standard deviation divided by the mean of the measurement value and expressed as a percentage. Most investigators consider that devices with a COV less than 10% have high repeatability, while a COV less than 5% indicates very high repeatability. Bland-Altman plots were used to assess agreement. Comparison of COV between different types of cataracts (nuclear, cortical, or posterior subcapsular lens opacity according to the Lens Opacities Classification System III system) was performed using an analysis of variance (ANOVA).
Results
Thirty-five eyes from 35 subjects (20 men and 15 women) were examined. The age range was 45-66 years with a mean of 52.8 years. BCVA was 0.10 ± 0.23 before cataract surgery and 0.48 ± 0.21 after surgery ( Table 1 ). Twenty-two subjects presented with a nuclear cataract, 7 patients had a cortical cataract, and 6 subjects had a posterior subcapsular cataract, according to the Lens Opacities Classification System III system.
Before Surgery | After Surgery | P | |
---|---|---|---|
BCVA (Snellen scale) | 0.10 (0.23) | 0.48 (0.21) | <.001 |
Visual evoked potentials: amplitude P-100 (μv) | 10.55 (3.61) | 12.39 (2.69) | .036 a |
Visual evoked potentials: latency P-100 (ms) | 100.26 (5.77) | 100.12 (4.26) | .659 |
Pattern electroretinogram: amplitude P-50 (μv) | 2.31 (0.81) | 2.35 (0.74) | .315 |
Pattern electroretinogram: latency P-50 (ms) | 52.82 (4.40) | 52.76 (5.62) | .625 |
Pattern electroretinogram: amplitude N-95 (μv) | 5.15 (0.98) | 5.29 (0.71) | .404 |
Pattern electroretinogram: latency N-95 (ms) | 98.27 (7.82) | 98.11 (6.94) | .159 |
Pattern electroretinogram: ratio N95/P50 | 2.24 (0.77) | 2.18 (0.81) | .603 |
Intraocular pressure (mm Hg), mean (SD) | 15.14 (1.32) | 15.10 (1.44) | .365 |
Comparison of Parameters Before and After Cataract Surgery
We compared the parameters at 1 month before and 1 month after cataract surgery.
Neurophysiologic parameters provided by PERG were not significantly different, but the amplitude of P100 component of VEP increased after cataract surgery ( Table 1 ).
RNFL parameters obtained 1 month before and 1 month after cataract surgery using Cirrus and Spectralis OCTs were compared ( Table 2 ). Both devices detected significant differences ( P < .05). The greatest difference was in the superior quadrant (1.9 μm higher after surgery, P < .001) using the Cirrus OCT and in the inferotemporal sector (2.2 μm thicker after surgery, P = .025) using the Glaucoma application of the Spectralis OCT. Figure 1 shows the mean RNFL thickness differences before and after surgery and in the 4 quadrants measured by the Cirrus OCT and the Glaucoma application of the Spectralis OCT. A slight increase in RNFL thickness after surgery was observed for all measurements.
Before Surgery (μm) (Mean ± SD) | After Surgery (μm) (Mean ± SD) | P | |
---|---|---|---|
Cirrus optical coherence tomography | |||
Mean thickness | 76.6 ± 9.8 | 78.2 ± 13.7 | .003 c |
Superior thickness | 89.6 ± 10.2 | 91.5 ± 15.7 | <.001 c |
Nasal thickness | 64.7 ± 16.1 | 66.4 ± 17.0 | .011 c |
Inferior thickness | 112.4 ± 14.4 | 113.2 ± 13.7 | .123 |
Temporal thickness | 79.7 ± 10.1 | 80.8 ± 14.0 | .008 c |
Glaucoma application of Spectralis optical coherence tomography | |||
Mean thickness | 82.9 ± 10.2 | 83.5 ± 9.6 | .043 c |
Superior thickness | 96.3 ± 9.1 | 98.2 ± 8.6 | .009 c |
Inferior thickness | 104.5 ± 9.1 | 103.9 ± 9.4 | .302 |
Superonasal thickness | 86.3 ± 9.3 | 86.5 ± 12.2 | .225 |
Nasal thickness | 67.6 ± 11.2 | 67.1 ± 11.9 | .169 |
Inferonasal thickness | 83.9 ± 13.6 | 84.2 ± 14.3 | .569 |
Inferotemporal thickness | 136.5 ± 11.1 | 138.7 ± 10.5 | .025 c |
Temporal thickness | 83.5 ± 13.7 | 84.3 ± 14.8 | .019 c |
Superotemporal thickness | 103.0 ± 13.9 | 104.1 ± 12.8 | .004 c |
a Cirrus (Carl Zeiss Meditech, Dublin, California, USA).
b Spectralis (Heidelberg Engineering, Carlsbad, California, USA).
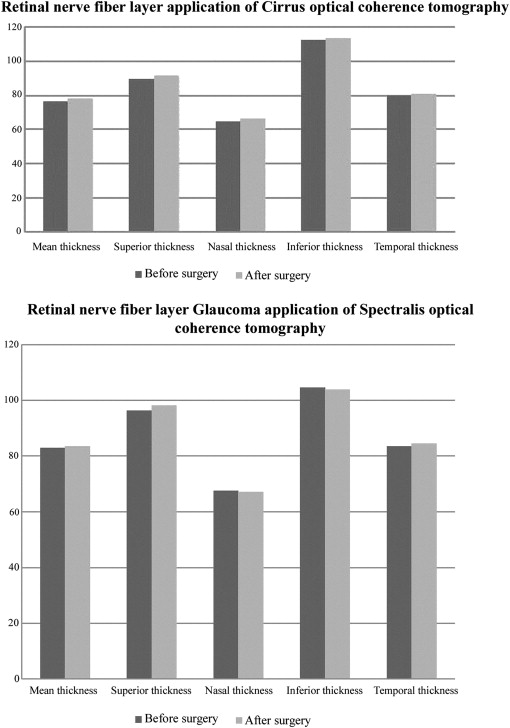
Macular parameters obtained by the Cirrus and Spectralis OCTs in the 2 visits were also compared ( Table 3 ). All macular parameters were significantly increased after surgery with Cirrus OCT ( Figure 2 ) and the largest difference was in the inferior inner thickness (11.8 μm higher after surgery, P = .004). Using the Spectralis OCT, however, there was no significant difference in the macular thickness measurements after surgery ( Figure 2 ). Mean thickness measurements, based on 3 individual scans, were used for the OCT analysis.
Before Surgery (μm) (Mean ± SD) | After Surgery (μm) (Mean ± SD) | P | |
---|---|---|---|
Cirrus optical coherence tomography: macular parameters | |||
Fovea | 227.3 ± 13.7 | 236.7 ± 15.8 | .002 c |
Superior inner macula | 263.1 ± 15.9 | 268.2 ± 12.1 | .011 c |
Nasal inner macula | 248.3 ± 16.8 | 258.3 ± 17.9 | .009 c |
Inferior inner macula | 258.1 ± 14.6 | 269.9 ± 13.6 | .004 c |
Temporal inner macula | 247.9 ± 16.8 | 256.2 ± 17.9 | .012 c |
Superior outer macula | 249.7 ± 14.1 | 260.1 ± 15.7 | .025 c |
Nasal outer macula | 255.1 ± 12.0 | 263.7 ± 13.3 | .035 c |
Inferior outer macula | 250.7 ± 13.7 | 258.3 ± 11.9 | .049 c |
Temporal outer macula | 228.4 ± 11.5 | 235.7 ± 13.8 | .028 c |
Spectralis optical coherence tomography: macular parameters | |||
Fovea | 232.4 ± 13.7 | 232.8 ± 13.9 | .177 |
Superior inner macula | 271.9 ± 12.7 | 272.2 ± 13.2 | .419 |
Nasal inner macula | 258.5 ± 14.8 | 259.1 ± 16.8 | .371 |
Inferior inner macula | 263.9 ± 17.4 | 264.0 ± 15.7 | .658 |
Temporal inner macula | 261.6 ± 18.9 | 260.8 ± 19.2 | .455 |
Superior outer macula | 263.5 ± 16.7 | 263.9 ± 13.9 | .771 |
Nasal outer macula | 270.4 ± 12.6 | 269.7 ± 13.6 | .489 |
Inferior outer macula | 268.9 ± 11.8 | 269.2 ± 11.9 | .121 |
Temporal outer macula | 249.7 ± 13.1 | 250.1 ± 14.6 | .466 |
a Cirrus (Carl Zeiss Meditech, Dublin, California, USA).
b Spectralis (Heidelberg Engineering, Carlsbad, California, USA).
