Implantable Hearing Devices
Charles C. Della Santina, Ryan M. Carpenter, Debara L. Tucci, and John K. Niparko
Advances in signal processing and implantable devices for the auditory system have led to a wide range of treatment opportunities for individuals with sensorineural hearing loss (SNHL). This chapter examines the neurobiology of SNHL, the development of technologies that enable a restored perception of sound, and the clinical application of implantable hearing technologies. We describe in detail practices related to cochlear implantation, including selection of candidates, techniques of device placement and activation, and the use of cochlear implants as a communication tool.
Cochlear Implants
Cochlear implants are neural prostheses that convey sound information to the auditory nervous system via electrical stimulation of the auditory nerve, bypassing hair cells that are dysfunctional in deaf individuals. In a typical device, sound signals detected by a microphone are decomposed into spectral components that each vary in intensity with time. These variations are encoded by the intensity and timing of electrical current pulses delivered via multiple electrodes inserted into the cochlea, with each spectral component represented by the one or more electrodes positioned near the subset of auditory nerve fibers that are normally sensitive to the corresponding range of frequencies (Fig. 31–1).
Electrical currents delivered by cochlear implants activate the auditory pathway in deaf individuals through stimulation of the robust population of surviving auditory neurons that subserve the principal speech frequencies. A significant proportion of auditory nerve axons survives even when deafness is profound and early in onset, and these neurons retain their responsiveness to electrical stimulation (Fig. 31–2). Auditory neurons activated by the electrical stimulation convey via spike trains the physiologic code that can support the detection and discrimination of complex stimuli.
Cochlear implants support language acquisition by providing auditory informational cues that complement the visual cues available to individuals with profound hearing loss. For most young recipients, developmental learning in the early, formative years enables processing of information from the implant to facilitate speech comprehension and oral language development. As the developmental effects of profound hearing loss are multiple, cochlear implants have been applied to ever younger children in an attempt to mitigate delays in developmental learning, particularly with respect to oral language development, the outcome of primary interest in most cases.
The outcomes associated with cochlear implantation span a range and depend heavily on patient factors. Many patients see dramatic benefit from the implant and demonstrate remarkable functional performance, whereas others may not reach the levels of speech recognition or sound perception to which they aspire. In either case, hearing via a cochlear implant is not the same as normal hearing. Optimal outcomes rarely occur without careful assessment of candidacy to guide surgical and postoperative care. Establishing appropriate patient (or parent) expectations prior to implantation is an important adjunctive goal of candidacy assessment because unrealistic expectations can negatively impact patient participation in essential postoperative rehabilitative care.
Assessing Candidates for a Cochlear Implant
Comprehensive assessment of candidacy is essential to minimize risks and realize benefits of cochlear implantation. To ensure complete assessment of candidacy, clinicians should consider the many factors likely to affect performance with a cochlear implant, including audiologic, medical, surgical, developmental, cognitive, and psychosocial factors. Candidates (or parents) should understand that the cochlear implant is a communication tool and is not curative because expectations largely shape postoperative satisfaction with any form of auditory rehabilitation.1
Hearing History and Audiologic Testing
Candidacy should be considered in the context of current functional status and likely outcome with and without cochlear implantation. Patient age, etiology of hearing loss, unaided and aided audition, duration of deafness, and the circumstances of social support surrounding the candidate carry predictive value. Environments that enrich and promote spoken language are likely to exert a favorable influence over use of the device and contribute to maximal benefit from it.
Figure 31–1 A cochlear implant is inserted into the scala tympani of the cochlea via the facial recess and a cochleostomy near the round window. The device’s multiple electrodes rest near endings of the cochlear (auditory) nerve, which are arranged from apex to base according to the frequency of sounds to which they are normally sensitive. (Courtesy of J. Buchman, 1993.)
Tyler and Summerfield2 observed evidence of the influence of auditory plasticity in adults with hearing loss of postlingual onset. Speech perception ability and the duration of profound/total deafness before implantation were negatively and significantly correlated. Performance improved over time after implantation. For adult patients, the level of performance measured shortly after implantation was, on average, about half the level measured eventually. Performance tended to reach an asymptote after about 3 years of implant use.
Such observations suggest that an established pathway for auditory processing is present even in profound SNHL and that refined processing develops over time. Though there are additional negative correlations between duration of deafness and performance,2–5 such correlations do not apply to every case. Even a prolonged period of deafness does not rule out prospects for open-set speech understanding with a cochlear implant, provided that basic foundations of communicating through audition (e.g., prior hearing aid use, use of lip-reading, and the production of speech) are in place. The greatest contrast in performance of open-set speech recognition exists between adults who acquired deafness postlingually versus those with prelingual deafness.6 Accordingly, the effect of prelingual deafness on the integrity of the central auditory pathway is an active area of investigation.7–9 In children, clinical evidence of improved performance with earlier implantation10 and the greater benefit with length of use in children11 suggests that the effects of experience can have large effects on speech comprehension with longer implant use.
Preliminary consideration of implant candidacy in deafness is based on an individual’s baseline hearing and experience with amplification. As a first approximation of SNHL severity, unaided, pure-tone thresholds are measured using age-appropriate behavioral testing or auditory-evoked potentials. Other objective testing may include measurement of otoacoustic emissions and the acoustic reflex. Although such tests are a useful adjunct in adults and a proxy for speech audiometry in young children, results should be viewed in light of the variability of speech discrimination that can occur with conventional amplification for a given level of SNHL on pure-tone audiometry.
Except in young children, criteria for implantation depend mainly on preoperative speech discrimination, rather than pure-tone audiometry. Currently, criteria vary somewhat across different manufacturers and health care payers but generally include an upper threshold of 40 to 50% words correct (with sentence presentation) for the poorer hearing ear, with up to 60% in the better ear and pure-tone average hearing loss (for 500, 1000, and 2000 Hz) of 70 dB or greater in both ears. As experience with cochlear implants has grown, outcomes have continued to improve, and candidacy criteria based on speech discrimination have continued to evolve toward higher levels of function over the past 25 years.12,13 Mean recognition scores for words in isolation after implantation now far exceed the 40% level, and individuals with some preserved speech-recognition ability preoperatively often score substantially higher postoperatively than prior to implantation.3,14 Residual hearing as reflected in aided speechrecognition levels is an important predictor of implant success.4,5 When combined with duration of deafness, preoperative scores on tests of sentence recognition provide a predictive composite that accounts for approximately 80% of the variance in postoperative word recognition.13
Figure 31–2 (A) Histologic section through the modiolus of a human cochlea (hematoxylin and eosin stain), and (B) corresponding artist’s rendition, showing the spiral staircase arrangement of cochlear nerve cell bodies and distal sensory dendrites. Although distal processes may recede in deafness due to hair cell loss, viable cell bodies in Rosenthal’s canal remain close to the stimulating electrodes of a cochlear implant in the scala tympani. St, scala tympani; cd, cochlear duct (scala media); sv, scala vestibule; Rc, Rosenthal’s canal; cn, cochlear nerve; h, helicotrema. [(A) From Niparko JK, ed. Cochlear Implants: Principles and Practices. Philadelphia: Lippincott-Williams & Wilkins, 2000, with permission. (B) Courtesy of Max Brödel, Max Brödel Archives, Johns Hopkins School of Medicine, Baltimore, MD. Reprinted with permission.]
An alternative approach to discrimination data in infants and toddlers employs criteria-referenced measures. The Meaningful Auditory Integration Scale (MAIS) consists of 10 questions directed to caregivers. The questions assess device (hearing aid or implant) bonding, spontaneous alerting to sound, and the ability to derive meaning from auditory events.15 Osberger16 found that MAIS scores of children with profoundly elevated baseline thresholds improved dramatically with cochlear implantation to levels that matched or exceeded those demonstrated by “gold” category hearing-aid users (i.e., those who achieved favorable outcomes through hearing aid use).
Selected pathologies present a unique challenge in the assessment of implant candidacy from an audiologic viewpoint. Starr and colleagues17 described auditory neuropathy (AN), a distinctive type of hearing deficit in which the level of speech recognition impairment relates poorly to pure-tone audiometry. Patients with presumed AN have normal outer hair cell function (as revealed by the presence of oto-acoustic emissions, OAEs). Inner hair cell or eighth nerve impairment is suggested by an absent or abnormal auditory brainstem response (ABR). Other findings include absent acoustic stapedial reflexes and word recognition abilities that are disproportionately poorer than predicted from thresholds. Experience with cochlear implantation in children with AN remains largely anecdotal, with published findings varying between observations of high18,19 to no20 implant benefit. Interestingly, in children with AN who benefited with implantation, ABRs were generated with implant-mediated stimulation. This suggests that electrical stimulation achieved greater neural synchrony than occurred with conventional amplification.
Auditory-driven behaviors should also be assessed. The auditory skills assessment evaluates the child’s ability to attend to and integrate sound with use of conventional amplification. A child may demonstrate residual hearing on audiologic evaluation, yet fail to show the skills needed to make use of that hearing in verbal communication. An auditory skills assessment determines a candidate’s ability to attend to sound, to integrate auditory perception with speech production, to make meaningful associations with sounds, and to integrate hearing in social interaction. A speech-language pathologist can determine how a child communicates with others, the candidate’s level of communicative intent, and the strategies used to support the intent to communicate.
The candidate’s skills in nonverbal problem-solving, attention, and memory affect the postimplant rehabilitation process and may provide predictive information regarding potential use and benefit. Baseline cognitive and psychological assessment of candidates includes measurement of verbal and nonverbal intelligence, visual-motor integration, attention, motor development, behavior, and candidate/family stress. The evaluator counsels candidates or parents about expectations and notes whether these expectations reflect a realistic view of what an implant can provide. Unrealistic expectations are addressed early, to reduce the risk of later patient frustration and consequent device nonuse.
Optimal performance with a cochlear implant requires continued long-term use of the device. Observations that speechrecognition performance asymptotes only after at least 3 years of use, on average, for young children21 and for adult users2 underscore the importance of patient engagement with the intervention.
Placement in an appropriate school environment is a key factor in optimizing benefit from the implant in children, and arrangements for this should be put in place before implantation. The school experience should provide opportunity for flexibility as the auditory skills of an implanted child develop and requirements for communication support evolve.22 An appropriate school environment provides stimulation of audition, maximum attention to language development, encouragement of spoken language, opportunities to interact verbally with adults and peers, and appropriate support services.
Otologic and Medical Assessment
Medical examination yields essential information about the health status of the candidate by identifying potential health concerns and determining suitability for general anesthesia. An otologic evaluation, including history and physical examination, is essential to identifying structural changes in the temporal bone that may affect surgical approach and feasibility. Clinical evaluation of the vestibular system may help to guide the choice of which ear is implanted, and may help predict whether implantation will produce vestibular sequelae.
Establishing the precise etiology of deafness may affect strategies for surgical implantation and postoperative rehabilitation. Meningitis may induce cochlear ossification, and cochlear dysplasia may require special consideration of the surgical plan.23,24 Cochlear otosclerosis and temporal bone fractures may be more likely to manifest adventitial facial nerve stimulation with activation of the implant,25 thereby necessitating a considered choice of implant system.
Eustachian tube dysfunction is commonly encountered in childhood candidates. Early management of eustachian tube dysfunction can facilitate accuracy in assessing the level of SNHL and response to amplification.
Tympanic membrane perforations may require treatment before implant surgery. Patients with chronic suppurative otitis media resistant to treatment or poor pneumatization may be treated with obliteration of the mastoid cavity and external canal closure, followed by cochlear implantation at a second operation 3 to 6 months later.26,27
Luntz et al28 found that 74% of children had one or more episodes of acute otitis media before implantation, whereas only 16% had the same diagnosis after implantation. Reduced infection rates were thought to be due to the natural tendency for otitis media to diminish with age, the use of intraoperative and perioperative antibiotics, and the effect of air cell removal as part of the mastoidectomy.
Vestibular Assessment
The main goal of vestibular function assessment prior to cochlear implantation is to identify significant asymmetry in labyrinthine function, so that cochlear implantation may be directed when possible to the ear with the weaker labyrinth. Although 50% of candidates tested with standard calorics29 and 40% of those tested using head impulse testing30 have bilateral vestibular hypofunction to some degree, significant asymmetry is common. When measured using caloric exams, 23% of 43 cochlear implant candidates exhibited ≥20% asymmetry of vestibular function.29 In another study, ice water caloric testing identified unilateral profound vestibular loss in 11% of 47 candidates.31 When measured using the quantitative head impulse test, 13% of 16 candidates had significant asymmetry.30
Cochlear implantation carries a 38% risk of some loss and approximately a 10% risk of severe or profound loss of vestibular function in the implanted ear as measured by caloric tests.31 When measured using quantitative head impulse testing, the risk of significant loss is approximately 10%.30 Because preexisting profound unilateral vestibular loss may be a marker for reduced tolerance of vestibular injury, the risk of implanting an “only balancing ear” may be even greater. Of five patients who received cochlear implants contralateral to an ear with profound vestibular loss, two developed bilateral vestibular hypofunction (BVH) and one of the two suffered complete failure of vestibular reflexes.31 Given this risk, cochlear implantation of an “only balancing ear” should only be performed after educating patients (or parents) about the possibility of inducing or exacerbating BVH, which can cause disabling postural instability, disequilibrium, and oscillopsia (degradation of visual acuity during head movement). At the same time, one should note that auditory input delivered by a cochlear implant can be essential to language development in young children, and that young patients generally compensate for the loss of vestibular function much more readily and completely than do adults.
At a minimum, vestibular assessment prior to cochlear implantation should include a focused history, a screening vestibular physical examination, and review of labyrinthine anatomy on computed tomography (CT) or magnetic resonance imaging (MRI) obtained for surgical planning. Inconclusive evidence of asymmetric vestibular function should prompt further investigation, including quantitative testing to clarify and document baseline function and to help guide choice of ear to implant.
Imaging Studies
High-resolution CT scans of the temporal bone define surgical anatomy and provide information about cochlear abnormalities that can aid the surgeon in surgical planning and patient counseling. Temporal bone CT scans should be obtained and reviewed for evaluation of temporal bone anatomy with attention to mastoid pneumatization, ossicular anatomy, position of great vessels, position of the facial nerve, caliber of the internal auditory canal (IAC), and labyrinthine anatomy.32 Scans are examined for evidence of cochlear malformation and ossification, enlarged vestibular aqueduct, and other inner ear and skull base anomalies that can affect implant surgery (Fig. 31–3). CT findings of cochlear patency generally correlate with surgical findings,33 but significant discrepancies can occur as a result of volume averaging.34,35
MRI may be a useful adjunct to CT for assessment of implant candidacy.36–38 Whereas CT is the procedure of choice for detailing bony anatomy, MRI is ideal for imaging soft tissues such as the membranous labyrinth, nerves in the internal auditory canal, and soft tissue within a cochlea en route to cochlear ossification after meningitis. High-resolution T2-weighted MRI is especially helpful for determining cochlear patency (by revealing the presence or absence of fluid within the scalae) and the presence of absence of nerves within the IAC in cases of otic capsule dysplasia (Fig. 31–4).
Fast spin-echo (FSE) imaging and fast imaging employing steady-state acquisition (FIESTA) have an advantage over both conventional spin-echo T1-weighted images and conventional T2-weighted images, which require longer scan time. The speed advantage of FSE allows the radiologist to obtain thinsection (2-mm) high-resolution T2-weighted images with excellent contrast in a fraction of the time needed for conventional spin-echo techniques.39 Arriaga and Carrier40 compared FSE T2-weighted MRI sequences with CT. In four of 13 patients evaluated with this protocol and CT, the MRI provided information not available from the CT.
Consideration should be given to conditions for which a patient may need future assessment with MRI. Implantation of a magnet in the internal device may be contraindicated in these patients. A nonmagnetic modification of one commercially available device can be made for patients whose medical or neurologic condition mandates future MR studies.41 Baumgartner et al,42 however, found that MRI applied to cochlear implant patients using different devices, imaged at 1 Tesla, did not cause implant malfunction or patient injury.
Figure 31–3 Axial (A) and coronal (B) CT scans of a normal right cochlea. (C-F) CT scans of the right ear in several cases of cochlear abnormality, including Mondini dysplasia (C), cochlear ossification after meningitis (D), enlarged vestibular aqueduct (E), and absence of the internal auditory canal (F).
Figure 31–4 (A) Axial T2-weighted MRI through modiolus of the cochlea of a normal right ear. The cochlea (single arrowhead) is clearly patent, and the scala tympani and scale vestibuli are just distinguishable. The cochlear nerve is visible as a dark linear void in the bright cerebrospinal fluid (CSF) signal of the internal auditory canal (*), as is one branch of the vestibular nerve en route to the vestibule (double arrowhead). (B) Axial FIESTA MRI image of a 4-year-old girl undergoing evaluation for cochlear implant candidacy. CT revealed cochlear agenesis on the right and absence of the internal auditory canal on the left. The MRI was obtained to ascertain whether stimulable tissue existed in the right side, and shows a facial nerve (double arrow) and single branch of the eighth cranial nerve going to the vestibule. (Courtesy of C. C. Della Santina.)
Cochlear Implant Surgery
The need for stable interactions between the implant and target neurons underscores the importance of careful, atraumatic device insertion. Traumatic array placement has been associated with poorer outcomes in clinical trials of adults43 and children.44 The trauma of inserting the device is minimized through appropriate design and careful surgical technique. With normal cochlear anatomy, the scala tympani offers an easily accessed, mechanically shielded site for electrode placement near the dendrites and cell bodies of auditory nerve afferent fibers. Anatomically based design of electrodes arrays with preformed curvature, stabilized silicone carriers, and anisotropic stiffness helps minimize the risk of injury to the basilar membrane as the device slides within the turns of the scala tympani.
Contemporary cochlear implantation procedures represent modifications of surgical procedures historically employed in managing chronic infections of the mastoid and middle ear. Prophylactic antibiotics are routinely administered to provide coverage for the placement of a prosthetic device; however, the necessity for this measure has not been established. General anesthesia is employed, paralytic agents are avoided, and a facial nerve monitor is often employed. An extended postauricular incision is made (Fig. 31–5), and the mastoid cortex and surrounding squamosa portion of the temporal bone are exposed by raising a “flap” of mastoid periosteum pedicled anteroinferiorly. Soft tissue flaps should accommodate stable placement of the implant at a safe margin from overlying incisions and enable internal device placement sufficiently away from the pinna to leave room for an ear-level processor.
A simple mastoidectomy is performed, preserving a slight overhang at the superior and posterior cortical margins (Fig. 31–6A,B). This provides protection for the connecting leads. The facial recess is opened to maximize visualization of the incudostapedial joint and cochlear promontory after adequate thinning of the bony canal wall, opening of the antrum, and removal of adequate air cells to enable systematic exposure of the horizontal semicircular canal, fossa incudus, and chorda-facial angle (Fig. 31–6C,D).
Figure 31–5 An extended postauricular incision (shown approximately 3 weeks postoperatively) used for transmastoid cochlear implantation. Many other incisions can be used. (Courtesy of John Niparko.)
Figure 31–6 Surgical procedures for transmastoid cochlear implantation. (A,B) A cortical mastoidectomy is performed, identifying the incus and horizontal semicircular canal eminence (hscc) and keeping the mastoid tegmen, sigmoid sinus, and posterior ear canal wall (peacw) intact. The facial recess is opened (C), the round window niche is identified (D), a well for later placement of the device case is created, a cochleostomy is made anteroinferior to the center of the round window niche, and the electrode array is inserted along a trajectory tangent to the center of the basal turn of the scala tympani (E-G), and then stabilized in place.
Before insertion of the electrode array, a well is created behind the mastoid to accommodate the receiver-stimulator portion of the internal device (Fig. 31–6E). The receiver-stimulator placement strategy should minimize protrusion, thereby reducing vulnerability to external trauma and restricting device movement, which can shear connecting leads and produce device exposure. If necessary, the overlying scalp is thinned to less than 7 mm in thickness to enable stable, magnetic retention of the external antenna. Hemostasis is confirmed prior to bringing the device into the surgical field, because use of monopolar electrocautery should be avoided once the implant is on the field.
Thinning of the bone anterior to the vertical segment of the facial nerve maximizes visualization of the round window niche via the facial recess (Fig. 31–6D; also see Fig. 31–1). As the dimensions of the tympanic cavity are established at birth and completed by 6 months of age, the risk associated with the facial recess approach is no greater in children than in adults.45 However, anecdotal experience suggests that facial recess aeration may be less in children younger than 12 months of age. The chorda tympani nerve may be sacrificed if the facial recess is small or if the array to be implanted requires a generous exposure.46 Permanent taste disturbance after chorda tympani sacrifice is rare. The round window niche should be exposed, and its tegmen and the round window membrane should be identified.47 Inspection of the posterior promontory allows for identification of the round window niche if it is bridged with bone; the niche is never more than 2 mm from the inferior margin of the oval window. A cochleostomy accesses the scala tympani, either directly through the round window membrane or indirectly through the promontory anteroinferior to the center of the round window niche. The preferred approach is debatable, but the priority should be to access the scala tympani without inducing direct drilling-injury to the basilar membrane, while providing adequate space for unimpeded insertion of the array carrier.48 The electrode array is advanced under direct visualization along a trajectory tangential to the basal turn of the scala tympani (Fig. 31–6F,G). Resistance to array insertion suggests the risk of buckling of the carrier. Because buckling of the implant can injure the spiral ligament, basilar membrane or cochlear nerve endings, aggressive insertion is avoided. Full insertion of the array within the basal turn of the cochlea requires an insertion depth of 25 to 30 mm, depending on array length. After insertion of the array the cochleostomy should be sealed gently with a small piece of fascia. The connecting lead should be stabilized within the facial recess to reduce the likelihood of the array extruding from the cochlea. Drilling a notch in the bridge of bone that defines the superior end of the facial recess provides a convenient slot in which the array lead can be stabilized.
Proximity of electrodes to targeted neuronal populations may influence channel selectivity. With closer approximation of electrodes to the modiolar wall, more focused stimulation of spiral ganglion cells may be achieved.49 Prior designs of electrode arrays that achieved closer position of electrodes to modiolar neurons using shim-like positioners were abandoned due to suspicions of predisposition to meningitis.50 Enhanced curvature of the carrier of the array is now favored as a strategy to achieve closer positioning. Fig. 31–7 shows examples of cochlear implant internal processors and electrodes that are currently available for clinical use in the United States.
Current electrode carriers are typically inserted over a distance of up to 25 or 30 mm (Fig. 31–8). Insertion along this length of the cochlea places electrodes of the array adjacent to fibers of the auditory nerve that normally subserve the entire range of speech frequencies.51 The aim is to simulate the frequency analysis normally provided by basilar membrane mechanics and the tonotopic pattern of fiber activation within the cochlea. Is deep insertion necessary to achieve optimal performance? Certainly insertion beyond the first portion of the basal (first) turn of the cochlea intuitively seems necessary to approach midfrequency neurons. There is some evidence that speech perception improves with deeper insertion.52 However, the need for insertion beyond 20 mm into the cochlea is as yet uncertain.
Figure 31–7 Internal processors and electrode arrays for devices currently available for clinical use in the United States. (A) Hi-Res 90K® by Advanced Bionics Corporation, a Boston Scientific Company. (B) Nucleus Freedom® by Cochlear Corporation. (C) Pulsar® by Med-El Corporation. (D) Close-up of distal portion of standard (top), split (middle) and compressed (bottom) arrays from Med-El. [(A) Courtesy of Advanced Bionics Corporation, a Boston Scientific Company. (B) Courtesy of Cochlear Corporation. (C,D) Courtesy of Med-El.]
Figure 31–8 Anteroposterior view x-ray of a cochlear implant in place in the right ear, with the curved portion revealing the depth of insertion into the cochlea (see offset dashed line tracing of intracochlear portion). Postoperative acoustic-only audiometry confirmed no change from preoperative pure-tone thresholds, confirming atraumatic placement. (Courtesy of C. C. Della Santina.)
Once the electrode array is in place, the receiver-stimulator is stabilized with sutures to the bony cortex or by virtue of a tight-fit subjacent to the pericranium and deep fascia of the temporalis muscle. As the incision is closed, the implanted device is covered with the skin of the scalp.
Revision Surgery
The feasibility of revision implant surgery, including single-channel to multiple-channel conversion to enhance perception of spectral cues, is now well recognized.53 However, patients facing reimplantation must be aware of the possibility of differences in sound quality and speech recognition after revision as occurs in roughly 10% of revision cases.54,55 Revision surgery often entails the need for revising the cochleostomy so as to avoid impediment of the replaced array by the existing scar within the scala tympani.
Implantation of Young Children
Implantation of infants and toddlers can be achieved with no greater risk of complication than that observed for adults.56–58 Experience with surgical implantation of young children has been reported by several centers, and results are encouraging.58–60 The most significant developmental changes take place in the size and configuration of the mastoid cavity, which expands in width, length, and depth from birth into the teenage years. Studies with nonhuman primates have shown that because cochlear implantation does not affect growth around bony suture lines, there is no adverse effect on skull growth.61 On the basis of measurements of skull expansion, Eby and Nadol62 recommended allowing 2.5 cm of electrode lead redundancy in the mastoid to accommodate head growth and to avoid electrode extrusion. Redundancy of the connecting lead actually does not appear to stabilize the electrode carrier unless it is protected from the tethering effects of connective tissue within the mastoid cavity.
Implantation in Children with Cochlear Ossification and Obstruction
Obstruction of each of the scalae may occur due to inflammatory changes (associated with meningitis, Cogan’s syndrome, syphilis, chronic otitis media, and malignant otitis externa), otosclerosis, and trauma.63 Labyrinthitis ossificans results in the formation of fibrous tissue, with the possibility of subsequent dystrophic calcifications deposited in the normally fluid-filled scalae. The scala tympani, especially in the basal turn, is the most common site of fibrous tissue and new bone growth, regardless of the etiology. However, near-total ossification of the cochlea as shown in Fig. 31–3D is unusual. Green et al64 reported only two cases of total ossification of 24 specimens with some degree of ossification. Balkany et al65 found that electrode insertion was possible in more than 90% of ossified cochleae. Fayad et al66 found ossification of the scala tympani that required drilling in 30% of 20 patients with cochlear otosclerosis, but the extent of ossification did not exceed 5 mm in any patient. Performance with the implant was found to be similar to that of patients without ossification.
Because many patients with cochlear ossification receive only partially inserted electrode arrays, performance may suffer either because of smaller numbers of available channels or spiral ganglion cell depletion. However, studies of implant performance with ossified cochleae have shown that general levels of auditory performance are similar to those of patients with patent cochleas.67,68 In a group of children with postmeningitic deafness (PMD), Francis and colleagues69 found no significant delays in cognitive performance or speech perception, with the exception of those children who exhibited evidence of meningitis-related hydrocephalus. Thirty-three percent of the children in the PMD group required drill-out of the scala tympani to at least the first turn of the cochlea, and 7% of the group had an incomplete electrode insertion. Data from this case series of 30 children suggest that central factors hold sway in predicting audiologic performance and that ossification does not preclude open-set speech recognition. At this time, however, no performance data are available to guide the surgeon in the choice between an extensive drill-out procedure70 that may allow complete electrode insertion or a more limited, less invasive procedure likely to allow only partial insertion of the electrode array.
Modified electrode designs may be used when ossification is suspected prior to operation. Compressed arrays include the same number of electrode contacts over a shorter length than conventional arrays (Fig. 31–7D). The split electrode offers the same number of electrode contacts on two carriers; one can be inserted through the conventional cochleostomy and another through an apical cochleostomy created distal to the obstruction.71
Implantation in Children with Cochlear Dysgenesis
A significant percentage of children with deafness of congenital onset exhibit cochlear malformation, typically manifested as cochlear hypoplasia (Fig. 31–3C) or a common cavity.72 Hypoplastic cochleas are associated with poor definition of cochlear turns and partitions between the modiolus and IAC and relatively low spiral ganglion cell populations.73 When associated with a narrow or absent IAC on preoperative CT scanning (Fig. 31–3F), innervation may be completely absent74 as supported by electrical evidence of lack of excitability with preoperative testing by means of a transtympanic electrode.75 Implantation of an ear with a severely narrowed IAC is thus contraindicated in the absence of evidence of peripheral auditory function. Preoperative electrical testing may help in determining this.
Mondini malformation (hypoplasia of the cochlea) is associated with shorter length and relatively low spiral ganglion cell populations, and often has a thin bony partition between the modiolus and a widened IAC.74 The latter anomaly accounts for the cerebrospinal fluid leak that can occur during a cochleostomy for electrode insertion. Other anomalies involving the round window niche and facial nerve have been observed in association with cochlear malformations.75,76 Despite associated anomalies, implantation of a sufficient number of electrodes within a malformed cochlea is feasible and can provide a surprising degree of speech perception in many subjects.75
Complications of Cochlear Implants
Cochlear implantation entails risks inherent in extended mastoid surgery and those associated with the implanted device. Cohen and colleagues77 characterized implant-related complications as major if they required revision surgery, and minor if they resolved with minimal or no treatment. A survey reported 55 major (12%) and 32 minor (7%) complications. Webb et al,78 reporting their experience with 153 patients, found 13.7% major complications and 13.7% minor complications. Hoffman and Cohen79 noted that in later follow-up 220 (8%) major and 119 (4.3%) minor complications occurred among 2751 implantations. Direct comparisons of complication rates between reports fail to include information on duration of device use, and studies vary in length and frequency of follow-up. Notwithstanding these limitations, longitudinal tracking indicates a substantial reduction in the incidence of major complications in the past 10 years.79
Major complications include facial nerve paralysis and implant exposure due to flap loss. Facial nerve injury is uncommon and, when recognized promptly, unlikely to produce permanent, complete paralysis. Loss of flap viability can lead to wound infection and device extrusion, necessitating scalp flap revision and when intractable infection is present, device removal with or without replacement.
Device Failure
Major complications that are strictly device-related involve partial or complete device failure. As the materials used to fabricate the internal device are expected to maintain a hermetic seal for beyond 100 years, use-related failure per se is not expected. Device failure is attributed to either flaws in manufacturing or trauma. Device failure as a result of loss of electrical function in the external processor commonly produces a sudden loss of function and, therefore, hearing. Intermittency and rarely “popping” sensations occur before processor failure. External processor function may be lost with direct trauma, exposure to water, and, most frequently, normal wear and tear of connecting lead-wires linking the processor unit with the magnetically retained antenna that relays information to the internal device.
Although much less prevalent, device failure as a result of loss of electrical function in the internal device is of considerably greater concern. An internal device failure typically presents as either an immediate cessation of function or intermittency associated with reduced quality of sound and a period of diminishing function over days to weeks. Reports of painful stimulation have been noted, but are rare.
Diagnosis of the mode of failure of an internal device is hampered when the implant lacks telemetry to enable electrical assessment of all contained circuits. When telemetry is available, removing select channels from the “map” of stimulated circuits may allow for continued function. In the absence of telemetry, revision surgery may be the only alternative.
Explanted devices are sent to the manufacturer for assessment of circuit integrity. Formal data on analyses of explanted devices have yet to be reported. However, for the purposes of this review the senior author interviewed manufacturers’ engineers and found general trends in patterns of failure across manufacturers. Of devices with a recognizable fault, the general pattern of failure mode occurs with a relative incidence of:
• Hermeticity or moisture: 75%
• Electronic/hybrid failure: 16%
• Electrode/connecting lead: 9%
Cochlear implants have maintained a historical reliability of 99% at 1 year. Reported trends suggest that device reliability has improved over the past 30 years.80 For example, the failure rate for the third-generation Cochlear Corporation (Lane Cove, New South Wales, Australia) device released in 2001 (0.3% per year) is approximately one third of that associated with their first-generation device (0.8%) used from 1985 to 1998. The United States Food and Drug Administration (FDA) has examined manufacturing processes at all three major manufacturers with public disclosure of concerns relating to two of the three companies.81,82
Device failure is the most common indication for revision surgery and cochlear reimplantation. “Upgrades” to more advanced models are rarely indicated, as the level of performance enhancement achievable is subject to individual case consideration. Infection and flap breakdown require reimplantation less frequently.83
Luetje and Jackson84 reported a 9% rate of device failure in a review of 55 children. Their findings matched results found by Parisier et al.85 The most common failures included fracture of the central pin feed-through for the antenna coil, damaged integrated circuits in the internal receiver from electrostatic discharges (occasionally associated with contact and friction with playground-grade polyethylene), damaged electrodes at the point of exit from the internal receiver, capacitor failure, and electrode-array damage.
Detection of device failure is imperative in the implanted child. The analysis of Parisier et al identified four major risk factors that point to failure: fluctuations in threshold and comfort levels of nine units for more than six electrodes, performance incompatible with age and duration of deafness, complaints of extraneous noises and intermittent shocks, and a high number of external equipment changes. Tests on the device showing an absence of recorded output, abnormal pulse configuration, or lack of amplitude increase in response to increased stimulation can verify failure. Weise et al86 found evidence of head trauma contributing to a greater risk of device failure in children relative to adults.
Prevention of device failure begins with proper securing of the implant, particularly the connecting lead between the receiver/stimulator and the electrode array, where the device is vulnerable to shearing. Surgeons should secure the device by embedding it in a well drilled in bone and fixing it in place with permanent suture material. Although the evolution of implant design has diminished the rate of device failure, these precautions may help to shield the device from traumatic events.
Implant Infection and Meningitis
The risk of bacterial infection of an implanted device producing labyrinthitis or meningitis and associated reactive fibrosis and destruction of neural elements appears to be low. Franz et al87 inoculated the bullae of implanted cats with streptococci to evaluate the risk of infection spreading from the middle ear into the implanted cochlea. Despite resulting inflammation of the round window niche, cochlear inflammation was absent. This finding was attributed to a seal that had formed around the electrode at the entrance to either the round window or the cochleostomy, thus forming a protective barrier against implant-surface colonization and bacterial biofilm.88
In 2002, an increase in the number of postimplantation meningitis cases was noted anecdotally. Initial reports suggested a higher risk of meningitis in patients implanted with a particular device design and particularly when placed with an intracochlear shim (electrode positioner) and the manufacturer ultimately recalled unimplanted devices utilizing the positioner. The level of risk did not suggest the need for positioner removal unless repeated infections occurred. Since then, continued studies have revealed a higher risk for the disease in patients with all cochlear implants compared with the general population. Children appear to be particularly affected: of the 52 cases originally reported by the FDA, 33 (63%) were under the age of 7.
Reefhuis et al50 conducted a study of 4264 children implanted between 1997 and 2002 and found 29 cases of bacterial meningitis in 26 children. This rate of meningitis (caused by Streptococcus pneumoniae) was 30 times the incidence in the general population. Although the use of a positioner increased the likelihood of contracting meningitis, even children implanted without a positioner had rates of meningitis 16 times higher than the general population. Casecontrol analysis found the following risk factors: a history of placement of a ventriculoperitoneal cerebrospinal fluid (CSF) shunt, a history of otitis media prior to implantation, the presence of CSF leaks alone or inner-ear malformations with CSF leak, the use of a positioner, incomplete insertion of the electrode, signs of middle-ear inflammation at the time of implantation, and exposure to smoking in the household. Although the incidence of meningitis decreased considerably after the perioperative period, cases still appeared even 2 years after implantation.
The design of the study conducted by Reefhuis et al, as noted by the authors, did not permit the comparison of meningitis risk between deaf children with and without a cochlear implant. That is, there is no comprehensive epidemiologic analysis of the risk of meningitis in deaf children in general. It is biologically plausible that deafness itself poses greater risk of meningitis given the strong association of deafness with skull base anomalies that predispose individuals to the disease. It is also possible that any surgical implant placed in or near the skull base of toddlers and young children (e.g., CSF shunts) does indeed carry a prolonged risk of meningitis.
Cochlear Implant Programming
Initial activation of the cochlear implant typically takes place 3 to 6 weeks after implantation to allow sufficient time for healing of the surgical incision and resolution of the accompanying edema. During routine use of the cochlear implant system the implanted device receives input from an external battery powered speech processor housed in a behind-the-ear unit similar to a hearing aid or in a “body” style encasement worn at the waist, in a pocket, or otherwise harnessed to the body. A microphone captures acoustic input and delivers it to the speech processor, which, in turn, encodes the signal. An external antenna magnetically retained behind the ear transmits the encoded signal across the scalp via radio frequency to the antenna of the internal device. Examples of external equipment are shown in Fig. 31–9.
The object of device programming is to establish a set of parameters that will result in optimized access to and perception of auditory input for a given patient. The input and output characteristics of the program or “map” depend on factors such as the microphone response pattern and sensitivity, compression, channel gain, frequency allocation, and feature extraction. The electrode array can stimulate the auditory nerve using pulsatile or analog currents with presentation options from fully simultaneous across electrodes to one or more pairs to sequential. Physiologic measures, behavioral methods, sound-field audiometry, standardized tests, feedback from rehabilitation and educational professionals, and patient report contribute to considerations made in the programming process. In most cases programming decisions revolve around maximizing speech recognition and achieving audibility of all critical components of the speech signal. However, in some cases—for example prelingual hearing loss in an older child without a history of spoken language experience or with multiple sensory deficits—the target outcome may be improved awareness of environmental sounds, speech-reading support, or better functional communication through a combination of manual and oral language.
Dynamic Range
Among the first and most important steps prior to activating the cochlear implant is to determine appropriate stimulation levels. After establishing a connection between a computer and the implant and measuring electrode impedances via back telemetry, the clinician can begin to demarcate the dynamic ranges of individual electrodes in the implanted array. Maximizing the dynamic range is important because it is typically much smaller for electrical stimulation via a cochlear implant than for acoustic stimulation of the normal ear. Normal behavioral responses to acoustic stimulation span approximately 120 dB with corresponding individual fiber dynamic ranges of 20 to 40 dB, whereas corresponding dynamic ranges in electrical stimulation span only 10 to 40 dB and 7 to 10 dB, respectively.89,90
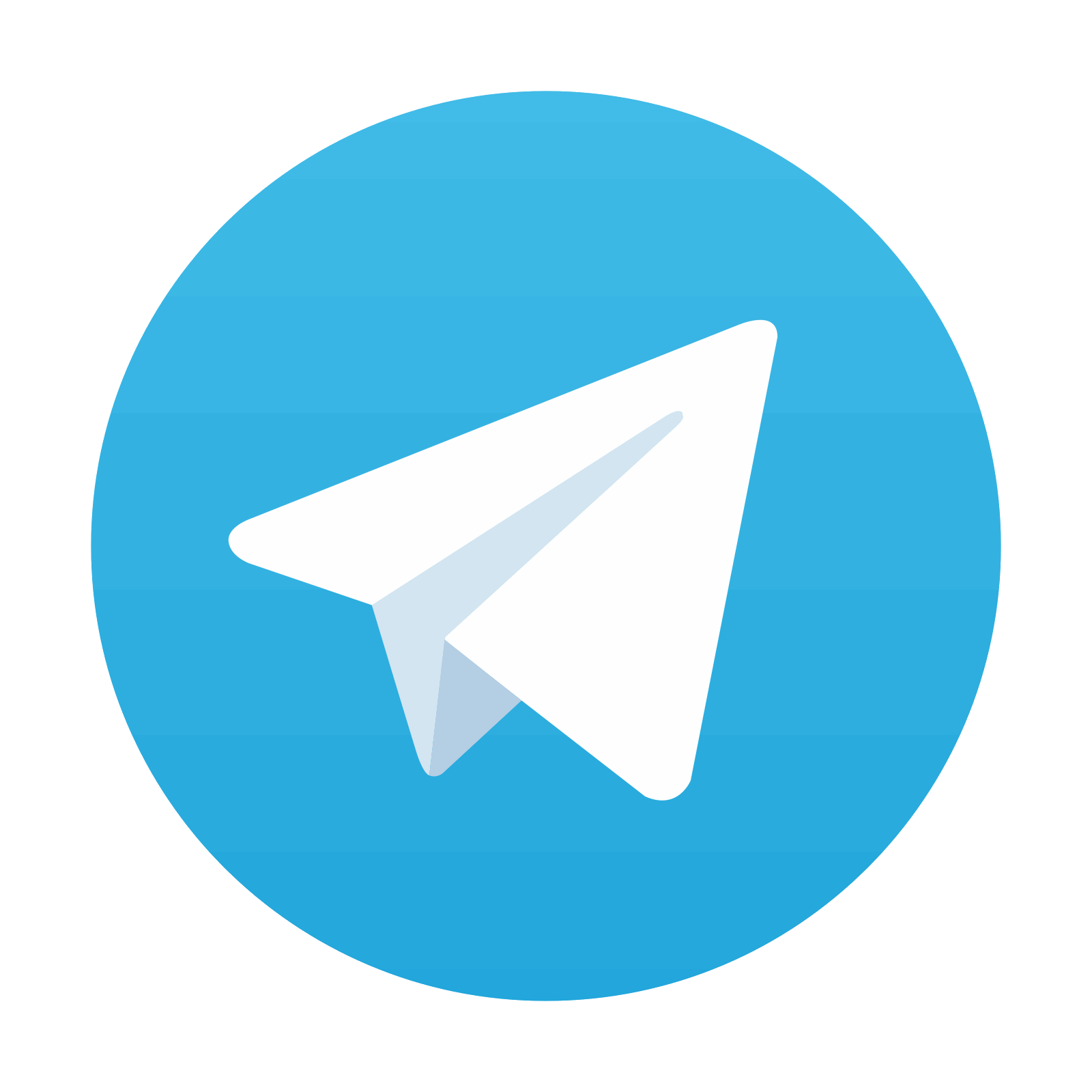
Stay updated, free articles. Join our Telegram channel

Full access? Get Clinical Tree
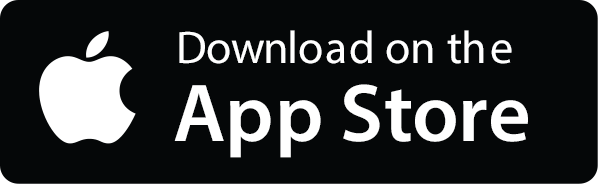
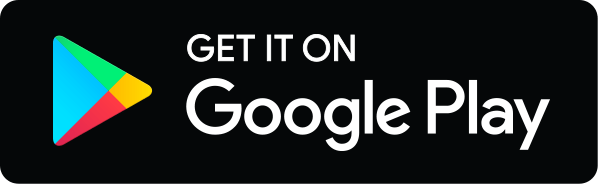