Hyperparathyroidism: Evaluation and Surgery
Maisie L. Shindo
ABSTRACT
Primary hyperparathyroidism is characterized by autonomous hypersecretion of one or more parathyroid glands. The incidence of primary hyperparathyroidism in the United States is approximately 100,000 patients per year. Causes of hyperparathyroidism can be classified as primary hyperthyroidism, secondary hyperparathyroidism, tertiary hyperparathyroidism, familial hypocalciuric hypercalcemia (FHH), and parathyroid carcinoma. Appropriate management of these patients with hyperparathyroidism requires a thorough understanding of the pathogenesis as well as typical and atypical presentations, knowledge of laboratory tests for proper workup, and interpretation of imaging studies for localization of parathyroid adenomas. The ability to rapidly measure parathyroid hormone (PTH) level has allowed parathyroid surgeons to assess biochemical cure intraoperatively. Thus, surgical treatment of primary hyperparathyroidism has evolved primarily into minimally invasive single-gland excision. However, bilateral exploration still plays an important role in treatment of secondary and tertiary hyperparathyroidism. This chapter discusses clinical, laboratory, and radiologic evaluation of the hyperparathyroid patient as well as surgical and postoperative management.
EMBRYOLOGY AND ANATOMICAL CONSIDERATIONS
The inferior parathyroid glands are derived from the third pharyngeal pouch and descend caudally with the thyroid and thymus. The superior parathyroid glands are derived from the fourth pharyngeal pouch, attach to the posterior surface of the superior or midportion thyroid and migrate caudally with it. This pattern of migration explains the variability in the final arrest of the parathyroid glands, resulting in many possible locations of ectopic or supernumerary parathyroid glands. The limited course of the superior glands leads to less variability in location compared to the inferior gland. The inferior parathyroid glands can typically be found inferior, lateral or posterior to the inferior pole of the thyroid, anterior to a plane drawn through the recurrent laryngeal nerve. They can be quite variable in location. The superior parathyroid glands are less variable in location, the vast majority of them typically located near the cricothyroid joint, just superior to where the recurrent laryngeal nerve courses medially to enter the larynx. The blood supply to the parathyroid glands is primarily from the inferior thyroid artery, and in some there is contribution from the superior thyroid artery.
Humans typically have four parathyroid glands, two superior and two inferior. Approximately 3% to 13% of adults have supernumerary glands, and 5% have fewer than four (1). Normal parathyroid glands vary considerably in shape, size, and color. They are usually 2 to 3 mm in width, 1 to 2 mm in thickness, and 4 to 6 mm in length. Each gland weighs an average of 30 mg.
CALCIUM HOMEOSTASIS
Maintenance of extracellular calcium homeostasis involves the interaction of calcium, PTH, calcitonin, and vitamin D upon several target organs (kidney, bone, intestines, and parathyroid). Parathyroid cells respond to minute changes in the extracellular ionized calcium concentration, such that a small change in calcium concentration results in a change in the release rate of PTH in the opposite direction. This calcium-sensing property of parathyroid cells allows for the precise regulation of the extracellular ionized calcium concentration within a narrow range, normally 1.2 to 1.3 mmol/L. The calcium-sensing ability of parathyroid cells is believed to be mediated by a G-protein-coupled receptor on the parathyroid cell surface, known as calcium-sensing receptor (CaSR) (2). Hypocalcemia
induces PTH secretion, which raises the plasma calcium level toward normal by effecting the release of calcium from bone, increasing renal calcium reabsorption, and stimulating the production of 1,25-dihydroxyvitamin D. Vitamin D in turn increases intestinal absorption of calcium. Osteoclasts and certain cells of the renal tubule also exhibit calcium-sensing properties (Fig. 134.1).
induces PTH secretion, which raises the plasma calcium level toward normal by effecting the release of calcium from bone, increasing renal calcium reabsorption, and stimulating the production of 1,25-dihydroxyvitamin D. Vitamin D in turn increases intestinal absorption of calcium. Osteoclasts and certain cells of the renal tubule also exhibit calcium-sensing properties (Fig. 134.1).
PARATHYROID GLAND HISTOLOGY
The parenchyma of the parathyroid gland comprises primarily chief cells that are small, round, and light in color because of very weakly staining acidophilic cytoplasm. The nucleus is centrally placed. Chief cells synthesize PTH. Oxyphilic cells are less frequent, entirely lacking in small children under 6 years, and increase in number with age. Their cytoplasm is strongly acidophilic; the nucleus is small and uniformly intensely basophilic. They contain large amounts of mitochondria. These parenchymal cells are arranged in anastomosing chords surrounded by delicate connective tissue septa with abundant capillaries. A considerable number of fat cells infiltrate the gland beginning around puberty and may account for about half the weight of the parathyroid glands in adults (Fig. 134.2).
HYPERPARATHYROIDISM
Hyperparathyroidism is classified into primary, secondary, and tertiary. Other rare causes of hyperparathyroidism include parathyroid carcinoma and FHH. It is important to understand the different causes of elevated PTH in surgical treatment considerations.
Primary Hyperparathyroidism
Primary hyperparathyroidism (1°HPT) is characterized by autonomous hypersecretion of one or more parathyroid glands. The incidence of 1°HPT in the United States is approximately 100,000 patients per year, and its prevalence is estimated to be between 0.2% and 1% (3). It predominantly affects those between the ages of 55 and 70 with a female-to-male ratio of 2:1. Recent reports from large series indicate that the etiology is a single parathyroid adenoma in 89% of cases, and hyperplasia or multiple adenomas in the remainder (4). The incidence of double adenomas ranges from 4% to 16% (5, 6, 7).
Secondary and Tertiary Hyperparathyroidism
Secondary hyperparathyroidism (2°HPT) is characterized by elevation of PTH level as a result of chronic hypocalcemia, most commonly from chronic renal failure, but it can also be caused by chronic vitamin D deficiency. In chronic renal failure, renal tubular excretion of phosphate is impaired, leading to hyperphosphatemia and binding with free calcium. In addition, renal hydroxylation of vitamin D is impaired leading to hypovitaminosis D. Vitamin D deficiency leads to decreased calcium absorption from both the gut and reabsorption of calcium in the renal tubules. The end effect is lower extracellular calcium, which in turn, stimulates the parathyroid glands to secrete PTH, resulting in hyperplasia of the parathyroid glands and elevation of PTH level. Other possible causes of elevated PTH associated with normal or low calcium are low calcium intake, inefficient calcium absorption from gastrointestinal disorders, and deficiency in intake of vitamin D; all these conditions result in low calcium and a compensatory rise in PTH level. In tertiary hyperparathyroidism, the chronically hyperplastic glands autonomously secrete PTH, which usually occurs after successful renal transplantation but can also occur after long-standing hypocalcemia of any other cause is corrected.
Parathyroid Carcinoma
Malignancy of the parathyroid gland is a very rare cause of hyperparathyroidism, representing less than 0.2% of patients with 1°HPT (7). It can rarely occur in association with hyperthyroid-jaw bone syndrome. Patients with this syndrome are carriers of the HRPT2 gene (8). This condition typically manifests with extremely high plasma calcium levels, usually greater than 13 mg/dL. Rarely a mass may be palpable (9).
Familial Hypocalciuric Hypercalcemia
FHH is an autosomal dominant genetic disorder characterized by mutations in the intracellular part of the CaSR (10, 11). The result is a defect in the CaSR of the parathyroid cell resulting in a lack of PTH suppression in the setting of hypercalcemia. Genetic linkage studies have shown that chromosome 3q is the major, but not the sole, locus for FHH-causing gene (11, 12). There are at least two other genetic loci, one linked to chromosome 19p and another family described in which linkage to 3q and 19q loci has been excluded but the FHH-causing gene has not yet been identified (13, 14). While this condition is usually diagnosed at a younger age, it may manifest for the first time in older patients who have not sought medical attention throughout life. It typically has a benign course without the hypercalcemia-induced morbidities and is therefore generally not a surgical disease (15, 16).
CLINICAL MANIFESTATIONS
The clinical presentation and complications of hyperparathyroidism depends on the degree of hypercalcemia and the rapidity in which the condition develops. The classic symptoms and manifestations of the disease are
Musculoskeletal: bone and joint pain, muscle pain, muscle weakness, osteopenia, osteoporosis, pseudogout, renal osteodystrophy
Genitourinary: nephrolithiasis, renal insufficiency, nocturia, polyuria
Gastrointestinal: constipation, peptic ulcers, heartburn, pancreatitis, abdominal pain, nausea
Neuropsychiatric: depression, anxiety, confusion, memory loss, impaired thinking or “brain fog” A popular mnemonic for remembering these symptoms is “bones, stones, groans, and psychiatric overtones.” Other manifestations that can also be seen are fatigue, calciphylaxis (soft tissue calcification), cardiocalcinosis, and band keratopathy.
EVALUATION
Evaluation of the hypercalcemic or hyperparathyroid patient starts with a good history and determination of what manifestations of the disease the patient has. Information on use of medications that can cause hypercalcemia should be elicited, specifically thiazide diuretics, lithium, dietary supplemental calcium, and vitamin D. Family history of hypercalcemia or hyperparathyroidism should be attained, as the patient may have familial hyperparathyroidism or FHH.
Laboratory Studies
The diagnosis of 1°HPT is made based on plasma calcium and PTH levels. Plasma calcium and PTH levels should be drawn on the same day. Normal range for plasma calcium in most laboratories is 8.5 to 10.2 mg/dL (or 2.2 to 2.5 mmol/L). Since most of the body’s plasma calcium is bound to albumin, a decrease in plasma albumin will lower the plasma calcium level even though the free calcium remains the same. Therefore if the albumin level is low, the plasma calcium should be corrected such that 0.8 mg/dL should be added to the total calcium for every drop in albumin of 1.0 g/dL. Alternatively, one can measure ionized calcium, which represents free calcium. Normal range for ionized calcium in most labs is 1.14 to 1.28 mmol/L. PTH is an 84 amino acid peptide chain with a C-terminal and an N-terminal. Laboratories in the past measured PTH levels by measuring the N-terminal or C-terminal. Today, the most accurate assessment of PTH level is measurement of the entire chain, known as intact PTH. Normal range for PTH in most laboratories is 15 to 72 pg/mL. The classic laboratory findings of 1°HPT are elevated plasma calcium level and high intact PTH level. Some patients may present with elevated plasma calcium levels and PTH levels only in the midrange to upper limits of normal. In the absence of 1°HPT, elevated plasma calcium should suppress the PTH level. Therefore, unsuppressed PTH in the setting of elevated plasma calcium is “inappropriate” and indicates 1°HPT. In addition to plasma calcium and intact PTH levels, it is also helpful to look at 24-hour urine calcium and serum phosphate levels. Typically in 1°HPT, the serum phosphate is low, and the 24-hour urine calcium is normal or elevated. Some patients may present with only periodic elevations of plasma calcium. In patients with mildly elevated or normal calcium, if the PTH levels are persistently elevated, the serum phosphate is low and 24-hour urine calcium is high (greater than 350 mg), the diagnosis of 1°HPT is likely. In a patient with normal or occasional mildly elevated calcium levels, intermittent mild elevation of PTH, normal phosphate and low to normal 24-hour urine calcium, the diagnosis of 1°HPT is somewhat questionable. If the diagnosis is uncertain, it would be prudent to repeat calcium and intact PTH levels and follow the laboratory values. Other than 1°HPT, the differential diagnosis of hypercalcemia includes thiazide diuretics, immobilization, and hypercalcemia of malignancy and granulomatous diseases. Contrary to 1°HTP, the PTH level is generally low in these conditions.
Elevated plasma calcium and PTH with a low 24-hour urine calcium is suggestive of FHH. The diagnosis of FHH should be considered if there is a family history of hypercalcemia, PTH is not markedly elevated, or the 24-hour urinary calcium is low. In that setting 24-hour urine for calcium, plasma calcium, serum creatinine and 24-hour urine creatinine levels should be obtained to calculate calcium-creatinine clearance ratio. These tests should all be performed at the same time. FHH manifests with hypercalcemia and mildly elevated or normal PTH levels (17, 18). In FHH, the 24-hour urine calcium is below normal range. The calcium-creatinine clearance ratio, calculated using
the following formula, is helpful in differentiating FHH from 1°HPT.
the following formula, is helpful in differentiating FHH from 1°HPT.

A ratio that is greater than 0.01 is consistent with 1°HPT, though does not absolutely exclude FHH, and less than 0.01 is suggestive of FHH.
Some patients may also present with plasma calcium values in the mid to upper normal range but exhibit elevated ionized calcium. In recent years, a new entity known as normocalcemic hyperparathyroidism has been recognized where calcium level, including ionized calcium, is normal and PTH is elevated (19, 20). It has been proposed that there is a generalized targeted tissue resistance to PTH in patients with this entity. A study by Maruani et al. (21) showed PTH-dependent functions of the kidney to be attenuated in the normocalcemic hyperparathyroid patients despite an identical primary hypersecretion of PTH. They concluded that: (a) PTH induces milder biologic bone effects than in hypercalcemic patients; (b) Calcium absorption in the renal tubular system is lower in patients with normocalcemic 1°HPT compared to that of patients with hypercalcemic form of the disease. (c) The ability of PTH to decrease tubular phosphate reabsorption and stimulate synthesis of 1,25-dihydroxyvitamin D is also blunted in the patients who remain normocalcemic, compared with those who are hypercalcemic. Normocalcemic 1°HPT can be difficult to diagnose, and 2°PTH from other conditions such as chronic vitamin D deficiency, renal insufficiency, and renal calcium leak need to be excluded first. In 2°HPT, the typical laboratory finding is elevated PTH but unlike 1°HPT, the plasma calcium level is low or normal. Those with 2°HPT from chronic renal failure also exhibit elevated blood urea nitrogen, serum creatinine, and phosphate.
Vitamin D levels should also be measured. In 1°HPT, the 25-hydroxy form will typically be low, and 1,25-hydroxy form is often elevated. Vitamin D levels are generally not used to make a diagnosis of 1°HPT but may be helpful in differentiating 2°HPT due to chronic vitamin D deficiency from “normocalcemic” 1°HPT. Since both conditions will present with normal calcium and elevated PTH, if the 25-hydroxyvitamin D level is low, one can correct the vitamin D deficiency and follow the calcium level. If the calcium level becomes elevated or the PTH level does not correct back down to normal range with vitamin D replacement, the diagnosis is likely to be 1°HPT.
Imaging Studies
While imaging studies are used primarily to localize parathyroid adenomas for surgical planning, they may also be helpful in confirming the diagnosis of 1°HPT. If the lab values are intermittently or only mildly elevated, the diagnosis of 1°HPT can be confirmed if the imaging study unequivocally detects a parathyroid adenoma. The two most commonly used imaging studies are parathyroid ultrasound and technetium-99m-sestamibi parathyroid (MIBI) scan. The sensitivity of each test varies considerably, depending on the equipment and more importantly how experienced is the individual performing or interpreting the study. In the hands of a highly experienced ultrasonographer, which can be radiologist, surgeon, or endocrinologist, the sensitivity is 80% to 85%. On ultrasound, parathyroid adenomas appear hypoechoic and hypervascular (Fig. 134.3). Generally ultrasound should be able to detect adenomas located dorsal to the esophagus. The limitation of ultrasound is detecting adenomas located retroesophageally or in the mediastinum. The principle of sestamibi parathyroid scanning is that 99Tc MIBI tracer is taken up by both the thyroid and parathyroid adenoma but washes out of the thyroid faster than the parathyroid. Therefore early images at 20 minutes after injection are obtained, followed by delayed images typically at 2 hours (Fig. 134.4A). It is quite specific; however, its sensitivity
can be low in detecting small glands. One of its limitations is that concurrent thyroid disease can result in a falsepositive study. Another limitation is that the images are two-dimensional (2D) planer views and therefore do not provide information on the depth of the adenoma. This information is important for glands that are located inferior to the thyroid. On a 2D planer anterior-posterior view, without the information on the depth of the gland, a retroesophageal or paraesophageal gland can look virtually identical to an anteriorly located inferior gland. Oblique views or single photon emission computerized tomography (SPECT) obtained in conjunction with the 2D planer sestamibi scans can be helpful in providing information on the anterior-posterior location of the adenoma (Fig. 134.4B). Knowing this information obviates unnecessary extensive or missed exploration. If localization with sestamibi scan and ultrasound performed in experienced hands fail, thin-cut CT with intravenous contrast may be helpful. It is especially useful in detecting ectopic glands such as paraesophageal, retroesophageal, or mediastinal glands (Fig. 134.5).
can be low in detecting small glands. One of its limitations is that concurrent thyroid disease can result in a falsepositive study. Another limitation is that the images are two-dimensional (2D) planer views and therefore do not provide information on the depth of the adenoma. This information is important for glands that are located inferior to the thyroid. On a 2D planer anterior-posterior view, without the information on the depth of the gland, a retroesophageal or paraesophageal gland can look virtually identical to an anteriorly located inferior gland. Oblique views or single photon emission computerized tomography (SPECT) obtained in conjunction with the 2D planer sestamibi scans can be helpful in providing information on the anterior-posterior location of the adenoma (Fig. 134.4B). Knowing this information obviates unnecessary extensive or missed exploration. If localization with sestamibi scan and ultrasound performed in experienced hands fail, thin-cut CT with intravenous contrast may be helpful. It is especially useful in detecting ectopic glands such as paraesophageal, retroesophageal, or mediastinal glands (Fig. 134.5).
![]() Figure 134.3 Ultrasound of left inferior parathyroid adenoma. Top: adenoma (P) between the trachea and carotid artery; Bottom: adenoma is at the inferior tip of the thyroid. |
INDICATIONS FOR SURGERY
Parathyroidectomy is indicated in patients with 1°HPT who have complications of the disease, such as nephrolithiasis, hypercalcemic crisis (calcium greater than 12.5 mg/dL), or are symptomatic with musculoskeletal pain, muscle weakness, or severe neuropsychiatric dysfunction (irritability, fatigue, confusion, or insomnia). However, since the gamut of muscular and neuropsychological symptoms is broad, it is often difficult to determine if the symptoms are truly due to 1°HPT. The indications for surgery in the asymptomatic patient were first established in 1990 by a NIH sponsored consensus panel and modified in 2002 (Table 134.1) (22). In 2009, the guidelines were rereviewed at the Third International Workshop on Asymptomatic Primary Hyperparathyroidism, and the recommendations basically remained the same except for three changes: (a) hypercalciuria (24-hour urine calcium greater than 400 mg) was eliminated, (b) creatinine clearance was changed from less than 30% to less than 60 mL/min, and (c) bone density T-score at any site less than −2.5 and/or previous fracture or
bone fragility (Table 134.1) (23). Since parathyroidectomy traditionally has been performed under general anesthesia and required bilateral exploration, which has potential significant risks, many asymptomatic patients had generally been observed rather than treated surgically. However, in recent years, parathyroid surgery has evolved into minimally invasive focused exploration often under local anesthesia, with significantly lower surgical risks. Therefore, surgical consideration should be given to asymptomatic patients, even in healthy elderly patients with a reasonably long life expectancy, if they are appropriate candidates for minimally invasive parathyroidectomy (MIP).
bone fragility (Table 134.1) (23). Since parathyroidectomy traditionally has been performed under general anesthesia and required bilateral exploration, which has potential significant risks, many asymptomatic patients had generally been observed rather than treated surgically. However, in recent years, parathyroid surgery has evolved into minimally invasive focused exploration often under local anesthesia, with significantly lower surgical risks. Therefore, surgical consideration should be given to asymptomatic patients, even in healthy elderly patients with a reasonably long life expectancy, if they are appropriate candidates for minimally invasive parathyroidectomy (MIP).
Multiple studies in the last decade have proven the benefits of parathyroidectomy for 1°HPT. Studies on quality of life postparathyroidectomy have been performed using the SF-36 survey, a self-ranked 36-item survey that assesses general health, as well as the Parathyroidectomy Assessment of Symptoms survey. Both have been shown to improve quickly after surgery (24, 25, 26, 27). Furthermore, these improvements are sustained long term (24, 27, 28). Bone density and hyperlipidemia have also been shown to improve with parathyroidectomy (29, 30).
TABLE 134.1 INDICATIONS FOR PARATHYROIDECTOMY IN ASYMPTOMATIC PATIENTS | ||||||||||||||
---|---|---|---|---|---|---|---|---|---|---|---|---|---|---|
|
Indications for parathyroidectomy in 2°HPT have been based mostly on presence of musculoskeletal symptoms, such as bone and joint pain, muscle weakness, or radiologic evidence of renal osteodystrophy. In recent years treatment with vitamin D and its analogs have allowed the majority of these patients to be managed by medical treatment for many years without severe symptoms of high bone turnover. Nevertheless, chronic high values of phosphate, calcium, and PTH in renal failure patients have been shown to be associated with increased mortality, mainly due to cardiovascular complications induced by ectopic calcifications (31, 32). Therefore, the main indication currently for parathyroidectomy in these patients is when serum phosphate, calcium, and PTH levels cannot be maintained within target ranges with vitamin D or D-analog therapy (32, 33). The introduction of cinacalcet, a new allosteric modulator of the CaSR on parathyroid cells that reduces PTH secretion, has also reduced the need for parathyroidectomy by 90% (34). Surgery is indicated when cinacalcet cannot be continued due to adverse side effects or poor compliance, or if the condition is refractory to cinacalcet therapy. Several studies have shown that parathyroidectomy in dialysis patients with advanced 2°HPT is associated with reduced incidence of major cardiovascular events and overall lower mortality (35
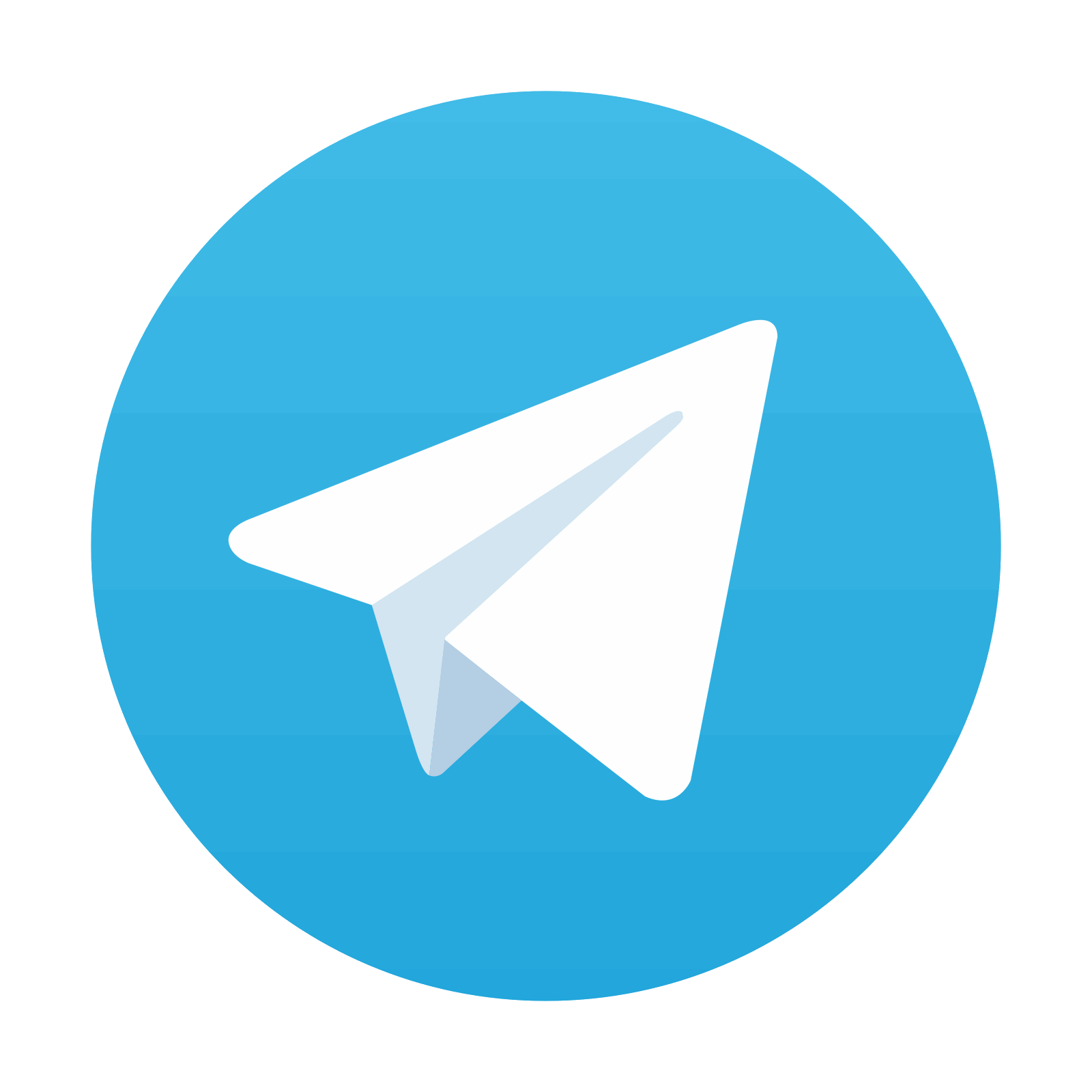
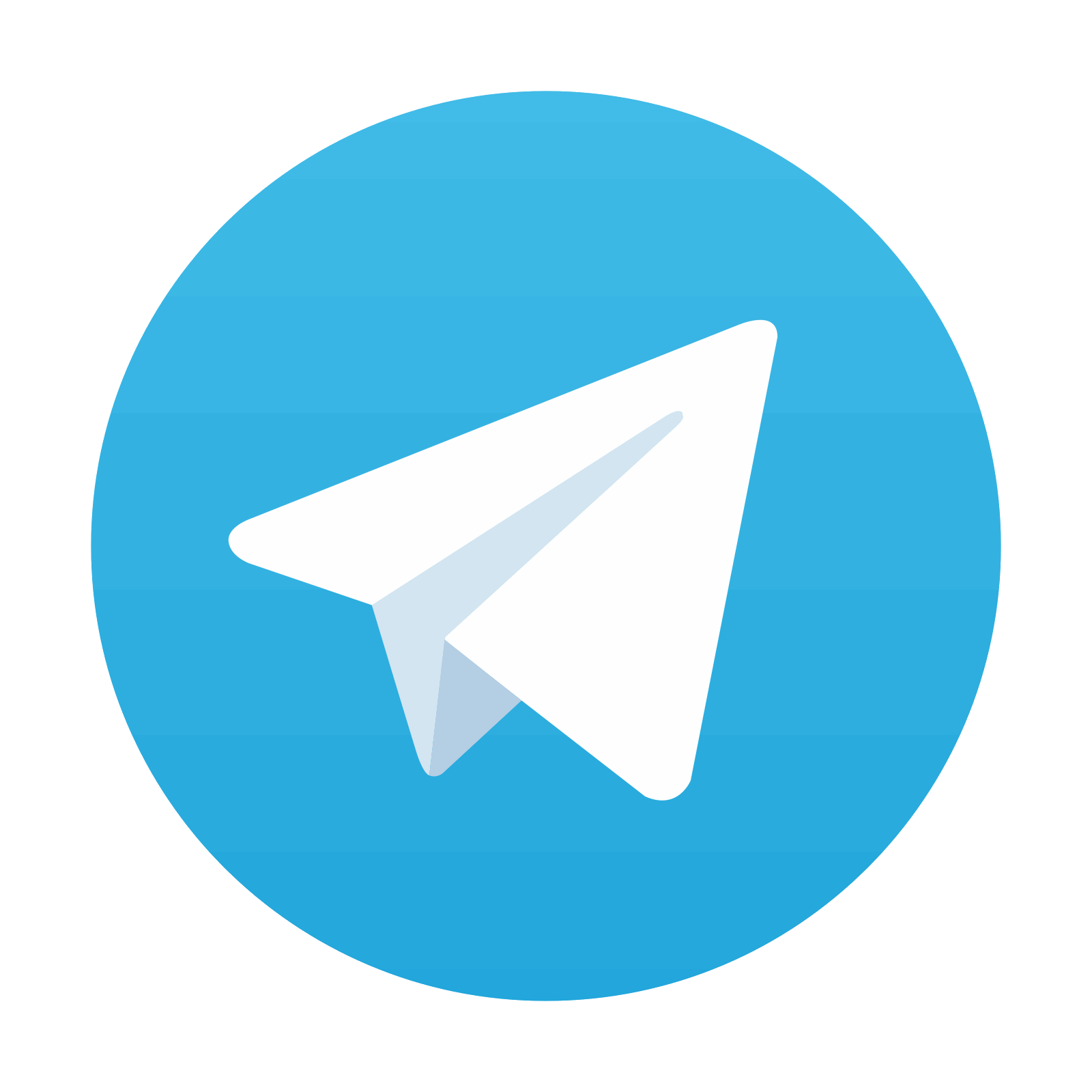
Stay updated, free articles. Join our Telegram channel

Full access? Get Clinical Tree
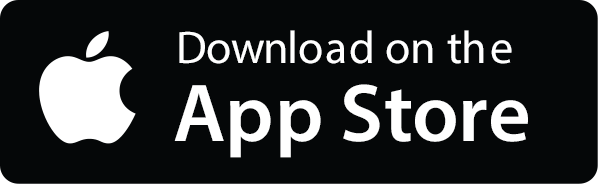
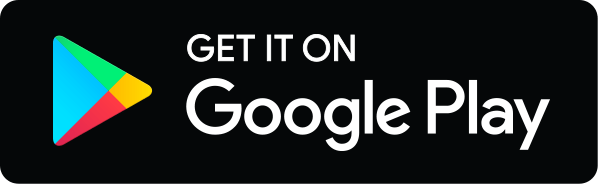