Hereditary and Congenital Hearing Loss
Genetics has emerged as an important topic in the field of audiology today. It is particularly valuable for the audiologist and health care professional to have a fundamental knowledge of genetics when discussing disorders of the auditory system due to the fact that many conditions have strong genetic associations. Although the purpose of this book is not to provide the reader with a comprehensive coverage of the genetic disorders that can result in hearing loss, we felt that we could not write a book on auditory disorders without dedicating some time to a review of basic genetic principles and disorders. It is beyond the scope of this book to discuss these topics in great depth; however, we provide the reader with some of the more critical concepts associated with genetics, along with a brief overview of the more common genetically based auditory disorders. For those interested in learning more about these topics, the following resources are recommended:
GeneReviews® (Adam et al., 1993–2019)
Genetics, Embryology, and Development of Auditory and Vestibular Systems (Jones & Jones, 2011)
Genetics Home Reference: Your Guide to Understanding Genetic Conditions (U.S. National Library of Medicine, 2019l)
Hereditary Hearing Loss and Deafness Overview (Shearer, Hildebrand, & Smith, 2017)
Hereditary Hearing Loss and Its Syndromes, Third Edition (Toriello & Smith, 2013)
Hereditary Hearing Loss Homepage (Van Camp & Smith, 2019)
Medical Genetics: Its Applications to Speech, Hearing, and Craniofacial Disorders (Robin, 2008)
The typical human being has 23 pairs of chromosomes, including 22 pairs of autosomes and a pair of sex chromosomes (i.e., XX in females and XY in males). These chromosomes contain genetic material composed of deoxyribonucleic acid (DNA) that is arranged on chromosomes in units called genes. DNA is inherited from one’s parents and is composed of four chemical bases or nucleotides. A nucleotide is a molecule that consists of a nitrogen-containing base (adenine, guanine, thymine, or cytosine in DNA and adenine, guanine, uracil, or cytosine in RNA), a phosphate group, and a sugar (deoxyribose in DNA and ribose in RNA). These nucleotides are strung together in sequences on strands. There are two strands of DNA nucleotides that bond together to form a double helix. The bond between these two strands is created by the pairing of adenine with thymine and guanine with cytosine.
Genes are composed of sequences of DNA that contain the “genetic instructions” for creating proteins. There are two copies of each gene, called alleles, with one allele located on each member of the chromosome pair. The genes carry the data (i.e., the DNA sequences) needed to make the various proteins that play important roles in the growth and development of the various structures and characteristics of the human body. This is accomplished via the processes of transcription and translation. Transcription is the process by which a complementary ribonucleic acid (RNA) copy of a DNA sequence is created (commonly referred to as messenger RNA or mRNA). This differs from translation, where short strands of transfer RNA (tRNA) nucleotides (carrying amino acids) pair with the corresponding mRNA bases that have traveled from the cell nucleus to the cell’s cytoplasm. The end result of these processes is the decoding of the genetic code and the assemblage of a protein with a specific amino acid sequence. There are several hundred of these proteins that result from these processes that are essential for the normal formation and functioning of the auditory system.
Changes may occur in the DNA material of the genes (called mutations) for a variety of reasons. If such changes occur, they may result in alterations in the end products of the genes (i.e., their proteins). In turn, abnormalities in the specific human growth or development processes in which these proteins are involved are likely to occur. Several types of gene mutations can occur, including nucleotide substitutions, deletions, and insertions. A comprehensive discussion of gene mutations is beyond the scope of this chapter. The reader interested in additional information on gene mutations is encouraged to access the resources listed previously as well as a basic text addressing the principles of genetics (e.g., Lewis, 2018; Read & Donnai, 2015).
The inheritance of a disorder can be caused by a single gene mutation or by more than one gene mutation, as in the case of digenic inheritance or modifier genes. There are two types of inheritance patterns: monogenic inheritance (includes Mendelian inheritance) and complex or multifactorial inheritance. When discussing monogenic inheritance patterns related to hereditary hearing loss, there are three patterns of inheritance that are commonly discussed: (1) autosomal recessive, (2) autosomal dominant, and (3) X-linked. The location of the gene (i.e., on either an autosome or the sex-linked X chromosome) and the number of mutated alleles (one or two) will determine the exact pattern of inheritance.
An important concept when one is discussing monogenic inheritance patterns is that of zygosity, which refers to the similarity of the gene’s two alleles. If the two alleles of a single gene are identical, they are said to be homozygous, whereas if one of the alleles is a wild-type (or normal) allele and the other is mutated, they are referred to as being heterozygous. If both alleles of a gene are affected but by different mutations, then the term compound heterozygous is used. The term hemizygous is used to refer to the condition where only one allele is present, as would be the case in sex-linked genes where only one X chromosome is inherited (e.g., XY).
An additional monogenic inheritance pattern sometimes seen in families with hearing loss is the mitochondrial inheritance pattern. This inheritance pattern is not as common as the other inheritance patterns. It is unique in that the inherited trait or characteristic can only be passed by a mother to her child through her mitochondrial DNA (mtDNA).
When an individual is seen for genetic evaluation, a geneticist or genetic counselor will draw a pictorial representation of the family called a pedigree, which includes the history of the family and the occurrence of a specific disorder or syndrome within the family. The individual who first brought the disorder or syndrome to medical attention is called the proband, and this individual is typically indicated in the pedigree by the presence of an arrow. The various symbols used in drawing a pedigree are shown in Figure 9–1, and these will be used in the pedigrees included in this chapter.
Autosomal Recessive Inheritance Pattern
In the autosomal recessive inheritance pattern, both autosomal gene alleles must have a mutation in order for the individual to have the disorder or syndrome. If both parents have an autosomal recessive disorder, then the offspring will inevitably inherit the disorder as the offspring will receive a mutated allele from each parent. However, if both parents are heterozygotes (i.e., they each have one mutated allele and one nonmutated or wild-type allele), the offspring may or may not inherit the disorder depending on the specific allele received from each parent as depicted in Figure 9–2. In the latter scenario, neither parent will manifest the disorder or condition, but both will be carriers of the trait that then can be passed down to their offspring. In such a situation, each parent has a 50% chance of passing a mutated allele to their offspring, and as such, there is a 25% chance of having an offspring with the disorder or syndrome, a 50% chance of having an offspring who does not have the disorder or condition but who would be a carrier (i.e., the offspring would have one mutated allele), and a 25% chance of having an offspring who would neither be a carrier nor have the disorder or syndrome. The same probability exists for every pregnancy and does not change as the number of pregnancies increases. Consanguinity (i.e., parents related by descent) significantly increases the chance that two parents will be carriers for the same mutated recessive gene that they inherited from their common ancestors. Figure 9–3 shows an example of a pedigree that might be observed when there is an autosomal recessive inheritance pattern.
Autosomal Dominant Inheritance Pattern
In the autosomal dominant inheritance pattern, the inheritance of one mutated allele is sufficient to cause a disorder or syndrome (Figure 9–4). With every pregnancy, there is a 50% chance for a parent with a specific disorder or condition to pass the mutated allele to his or her offspring. If the mutated allele is passed to the offspring, he or she will have the same condition or syndrome as the affected parent. Here, unlike in the case of autosomal recessive inheritance, many individuals in different generations of the family may have the disorder or syndrome; however, the severity and the age of onset of the condition may vary among these individuals. This phenomenon is called variable expression and refers to the range in phenotype that a particular genotype might cause (Welch, 2006). A phenotype is the clinical presentation or observable characteristics that result from the expression of a particular genotype (i.e., the constitution of a set of alleles present at one or more sites). For example, the same gene mutation may result in varying degrees of hearing loss within a family, with some individuals having profound hearing loss while others experience milder forms of hearing loss. Another phenomenon that can be associated with autosomal dominant inheritance patterns is reduced penetrance. This refers to the likelihood that a particular genotype will be expressed as a phenotype less than 100% of the time. For example, if 75% of individuals with a particular mutation are deaf and the remainder have normal hearing, this mutation is said to have reduced penetrance. Figure 9–5 provides an example of a pedigree that might be observed when there is an autosomal dominant inheritance pattern.
Figure 9–3. Pedigree showing autosomal recessive inheritance. Note: the shaded symbols represent individuals in the family tree with the condition and the arrow indicates the proband.
The X-linked inheritance pattern refers to the mode of transmission of genes on the X chromosome. This is a unique inheritance pattern as females have two X chromosomes (XX), whereas males have one X and one Y chromosome (XY), and most of the X chromosome genes do not have corresponding alleles on the Y chromosome. As a result, if a male has a mutation in a gene on the X chromosome, he will have the condition or disorder associated with that mutation because he only has the one mutated allele. Females, on the other hand, will not typically have the condition or disorder because they have one mutated allele and one wild-type allele. Figure 9–6 shows the inheritance pattern for a recessive X-linked disorder with a maternal carrier. In this inheritance pattern, there is a 25% chance that the couple will have a male offspring with the disorder or syndrome, a 25% chance that the offspring will be an unaffected male, a 25% chance that the offspring will be an unaffected female, and a 25% chance that the offspring will be an unaffected female who is a carrier for the condition. Figure 9–7 depicts a representative pedigree for a family with this type of X-linked recessive trait. The previous discussion addresses the mode of inheritance with a maternal carrier; however, an X-linked recessive disorder or condition can also be inherited paternally. If a male with the disorder or condition mates with a female with two wild-type (normal) alleles, then none of the male offspring will be affected, but all of the females will be carriers who may or may not show milder manifestations of the disorder.
Figure 9–5. Pedigree showing autosomal dominant inheritance. Note: the shaded symbols represent individuals in the family tree with the condition and the arrow indicates the proband.
Although most of the DNA in humans is located in the nucleus, a small amount is located in the energy producing organelles called mitochondria. Humans obtain their mitochondrial DNA from their mothers due to the fact that egg cells contain mitochondria and sperm cells do not. Hence, any mutations in the mtDNA are passed from the mother to all of her children, both males and females. Figure 9–8 provides an example of a pedigree that might be observed when there is a mitochondrial inheritance pattern.
For many disorders and conditions that result in hearing loss, the cause can be multifactorial (i.e., there can be an intricate interaction between genes and the environment). In these cases, individuals may inherit a predisposition to develop a disorder, but the disorder does not necessarily manifest itself if the individual is not exposed to the environmental conditions that will trigger the response. Genes and environmental factors may also cause a disorder in an additive fashion.
Figure 9–7. Pedigree showing X-linked recessive inheritance. Note: the shaded symbols represent individuals in the family tree with the condition and the arrow indicates the proband.
Figure 9–8. Pedigree showing mitochondrial trait inheritance. Note: the shaded symbols represent individuals in the family tree with the condition and the arrow indicates the proband.
An inheritance pattern can also be digenic. In these cases, mutations in two genes interact to cause deafness or hearing loss. In a few cases of inherited hearing loss, there are modifier genes that may determine if a genetic mutation will lead to a condition or disorder or not. Modifier genes interact with other genes in a variety of ways. As discussed by Welch (2006), one gene may determine if a person has hearing loss (or not), and a second gene may determine the degree of hearing loss experienced (mild versus profound, etc.). It is also possible that a gene may determine the presence or absence of a hearing loss, and an allele at a modifier locus may “save” the individual with the genotype from experiencing hearing loss. In this case, the individual presents phenotypically with normal hearing.
In humans, hearing loss is the most common sensory deficit. The prevalence of congenital hearing loss in newborns is about 2 to 3 per 1,000 (National Institute on Deafness and Other Communication Disorders, 2016). The overall prevalence of hearing loss increases dramatically with age due to the significant increase in the number of individuals with acquired hearing loss in older populations. It is estimated that 1 in 3 people between the ages of 65 and 74 years of age have hearing loss, and nearly half of those over the age of 75 years have hearing loss (National Institute on Deafness and Other Communication Disorders, 2018).
This increase in the prevalence of hearing loss in adults compared to children is due in part to the fact that genetic, medical/health, and environmental factors contribute to the development of hearing loss in many individuals, and in many cases, there are interactions among these factors (e.g., many individuals inherit a gene that increases their susceptibility to the damaging effects of noise exposure). Genetic etiologies account for the majority of hearing losses diagnosed in infancy (often detected by newborn hearing screening) or in very early childhood, with fewer hearing losses in this population attributed to environmental influences such as infections (i.e., cytomegalovirus), prematurity, ototoxicity, and so forth (Kochar, Hildebrand, & Smith, 2007). Genetic etiologies also contribute to a significant proportion of hearing losses of later onset (Kochhar et al., 2007). However, it is difficult to estimate the prevalence of genetic etiologies in age-related hearing loss due to the frequent presence of other comorbidities in older adults and the elderly, coupled with the fact that genetic testing is far less common among older individuals with hearing loss. In a recent publication, Shearer et al. (2017) provided estimates of the percentages of genetic and nongenetically based pediatric hearing losses. These authors estimated that 1 in 500 prelingual children in developed countries have hearing loss, with 80% of these losses being related to genetic causes and the remaining 20% being related to acquired conditions and/or environmental causes. Clearly, genetic etiologies play a major role in congenital hearing loss.
Genetic hearing losses (as well as hearing losses in general) are often classified by type and onset of the hearing loss. These classifications (described in detail in Chapter 3) include the following types of hearing loss: conductive, sensorineural, mixed, and central auditory dysfunction. In addition, the onset of hearing loss is taken into consideration with respect to if the hearing loss develops before the acquisition of speech (prelingual) or after the development of speech and language (postlingual). All congenital hearing losses are prelingual, but not all prelingual hearing losses are congenital. Perhaps the most common and useful classification of hereditary hearing loss is nonsyndromic versus syndromic hearing loss. These types of genetically based hearing losses are discussed in the next section.
Syndromic and Nonsyndromic Hearing Loss
Hearing loss is one of the most common of the sensory disorders, which affects individuals of all ages. As will become evident in the discussion that follows, genetic factors account for the vast majority of congenital hearing losses. Interestingly, even in hearing loss related to aging in the elderly population (i.e., age-related hearing loss), genetics (along with environmental causes in some cases) may play a role in the development of the hearing loss (Alford et al., 2014). In fact, approximately 30 gene mutations have already been associated with age-related hearing loss (Griffith & Friedman, 2017). Genetic hearing losses can be segmented into two main categories: syndromic and nonsyndromic. Syndromic hearing loss involves not only the auditory system but also other systems, making it a multifaceted disorder. Nonsyndromic hearing loss involves primarily the auditory system; however, in some situations, more than the auditory system can be involved but not nearly to the degree as is observed in syndromic hearing loss.
Nonsyndromic Hearing Loss
According to Shearer and colleagues (2017), nonsyndromic hearing losses are much more common in prelingual children than are syndromic hearing losses. They estimate that approximately 80% of the genetic hearing losses in this population is nonsyndromic, with syndromic hearing loss (which will be discussed later) accounting for about 20% of the genetically based hearing losses in prelingual children in developed countries around the world. Nonsyndromic hearing loss can be autosomal dominant or autosomal recessive, with the recessive form accounting for around 80% and the autosomal dominant form accounting for approximately 19% of all nonsyndromic hearing losses. Less than 1% of nonsyndromic hearing losses are reported to have X-linked, mitochondrial, or microRNA (miRNA) forms of inheritance (see Shearer et al., 2017). The miRNA pattern of inheritance, which was not discussed earlier, refers to a small noncoding RNA molecule that functions in RNA degradation or translation repression (Whaid, Shehzad, Khan, & Kim, 2010), and as can be seen in the statistics presented previously, it is an uncommon etiologic basis for genetic hearing loss.
Tables 9–1 and 9–2 provide examples of genes associated with autosomal recessive nonsyndromic hearing loss and autosomal dominant nonsyndromic hearing loss, respectively. Included in each of these tables are the functions associated with each of the genes mentioned. In addition, the typical onset of the hearing loss (i.e., either prelingual or postlingual) associated with these gene mutations is provided for each of the genes listed in both of these tables. These tables provide “examples” of gene mutations that have been associated with a hearing loss, but they are not intended to be exhaustive listings of all genetic mutations that can result in hearing loss. The reader is referred to Shearer et al. (2017) and Van Camp and Smith (2019) for additional information regarding nonsyndromic and syndromic gene mutations related to hearing loss.
Genes create proteins that play a critical role in the development, function, and dysfunction of structures throughout the various systems in the body. There are a variety of genes associated with the auditory system (Hood & Keats, 2011). According to Van Camp and Smith (2019), 75 autosomal recessive genes associated with nonsyndromic hearing loss have been identified. Identifying genes is a challenging process that includes defining the phenotype, finding families that have the genotype of interest, and then pursuing detailed family histories. A few of the better known genes (especially to the audiology and otology communities) are MYO7A, MYO15, and MYH9, which are motor molecules that encode the myosin protein. Also notable are the GJB2, GJB6, and GJB3 genes, which are all categorized as gap junction proteins that encode the proteins connexin 26, 30, and 31, respectively. The myosin genes are associated with movement of actin filaments and the maintenance of the tip-links of hair cells. These “movement” proteins make one think of the motile properties of the outer hair cells and the critical role they play in hearing. The GJB2 gene was one of the first genes identified that was associated with a high percentage of nonsyndromic hearing loss (autosomal recessive). Since the time of this discovery, there have been more than 100 mutations of the GJB2 gene categorized, with these mutations yielding varying degrees of hearing loss (Wingard & Zhao, 2015). Mutations in the connexin 26 gene are one of the major sources of hereditary hearing loss. It was once believed that a disruption in K+ recycling was the primary mechanism responsible for hearing loss associated with mutations of this gene; however, this has proven to in fact not be the case (see Wingard & Zhao, 2015). More recently, mouse models have demonstrated that hearing loss associated with connexin deficiencies is not directly related to hair cell degeneration but rather to cochlear developmental disorders (Chen, Chen, Zhu, Liang, & Zhao, 2014). This differs from late-onset hearing loss that is believed to result from a reduction of active cochlear amplification (Zhu et al., 2015). While advances have been made with respect to our knowledge of the genetic mechanisms underlying congenital and late-onset hearing loss, significantly more research in this area is needed.
Mutations of genes such as the ones that have been mentioned previously and/or referred to in the tables can result in hearing loss. Although in some cases certain mutations can result in severe to profound hearing loss, a similar mutation may also result in minimal hearing loss. This kind of variability seems common (Hood & Keats, 2011). Having said this, some trends in regard to hearing loss have been reported. First, a very high percentage of nonsyndromic hearing loss is sensorineural in nature, as the various gene mutations discussed previously affect proteins that influence the structures and functions of the cochlea. There is also some evidence that gene mutations often yield a certain configuration of hearing loss, although the degree of loss may vary. For example, mutations of the GJB2 gene are known to result in a sensorineural hearing loss. Although the degree of the sensorineural loss can vary from mild to profound, there is a trend toward the high frequencies being more involved than the low and middle frequencies in individuals with mutations of this particular gene (Shearer et al., 2017). The earlier discussion has focused on a few of the more common nonsyndromic genes associated with hereditary hearing loss; however, the reader should be aware that there are many other genes that also can result in nonsyndromic hearing loss when genetic mutations of these genes occur. For a more comprehensive listing of these genes, the reader is encouraged to access Shearer et al. (2017).
Syndromic Hearing Loss
More than 400 syndromes with associated hearing losses have been identified (see Toriello & Smith, 2013), most of which are rare. These syndromes can be the result of chromosomal anomalies, a single gene mutation, multiple gene mutations, or a combination of genetic and environmental factors. Most of these syndromes are inherited in the autosomal dominant fashion, but some are inherited in autosomal recessive and X-linked transmission patterns (Tables 9–3 and 9–4 provide information on some common syndromes along with their modes of transmission, gene symbols, and clinical findings). As mentioned previously, 20% of the genetically based hearing losses in prelingual children from developed countries around the world are syndromic in nature (see Shearer et al., 2017), and as such, they constitute a much smaller proportion of genetically based hearing losses when compared to nonsyndromic etiologies in this population of children.
Pendred Syndrome. Pendred syndrome is an autosomal recessive disorder with an unknown incidence. In addition to hearing loss, it is typically associated with a thyroid condition called a goiter. Exact prevalence data for Pendred syndrome are unknown; however, it is estimated that it accounts for 7% to 8% of all congenital hearing loss (U.S. National Library of Medicine, 2019e). The hearing loss is sensorineural in nature, and the high frequencies tend to be more involved than the low frequencies. The hearing loss is progressive, bilateral, and often ranging from moderate to profound in severity (Smith, 2017). A characteristic of patients with Pendred syndrome is enlarged vestibular aqueducts, which cause an enlargement of the endolymphatic duct and sac (see the following discussion on vestibular aqueducts). This genetic condition results in a defect in the ion transportation in endolymphatic fluid resorption, and patients also have euthyroid goiter. Pendred syndrome often results from a mutation of the SLC26A4 gene. In addition, the FOXI1 and KCNJ10 genes have also been linked to this syndrome (Smith, 2017).
Usher Syndrome. Individuals with Usher syndrome typically present with sensorineural hearing loss and retinitis pigmentosa. Usher syndrome has been reported to have a prevalence rate of 3 to 6 per 100,000 individuals (Saihan, Webster, Luxon, & Bitner-Glindzicz, 2009). It is believed to account for 50% of the individuals diagnosed with deaf-blindness (Lentz & Keats, 2016a). Several genes have been identified for this syndrome (see Table 9–3). These genes have a role in the structure and function of the cytoskeleton of stereocilia of the hair cells. Mutations in these genes are associated with retinitis pigmentosa (progressive visual loss and blindness), vestibular dysfunction, and ataxia in some cases. Usher syndrome is classified into 3 types, all of which are autosomal recessive.
Type I: Onset of retinitis pigmentosa by 10 years of age. Profound congenital hearing loss and absent vestibular responses are key characteristics. Pathogenic variants in one of six genes (MYO7A, USH1C, CDH23, PCDH15, USH1G, and CIB2) have been associated with this syndrome (Lentz & Keats, 2016a).
Type III: Onset of retinitis pigmentosa at puberty. Progressive hearing loss and variable vestibular function. This is typically a result of a pathogenic variant of CLRN1 gene (Smith & Jones, 2016).
This syndrome is associated with bilateral cochlear hearing loss that usually manifests after the presentation of visual symptoms (visual field cuts and night blindness). Cataracts can also develop in individuals with Usher syndrome. Types I and II account for the majority of Usher syndrome cases, whereas Type III is much less common and estimated to account for only 2% of Usher cases (see U.S. National Library of Medicine, 2019j).
Apert Syndrome. This syndrome has an autosomal dominant transmission pattern and has an incidence rate of about 1 in 100,000 cases that is linked to mutations in the FGFR2 gene (Robin, Falk, & Haldeman-Englert, 2011). Skull and skeletal malformations may include syndactyly (fusion of fingers and toes). A flat conductive loss is often noted bilaterally, although sensorineural loss may be present in some cases. A conductive hearing loss is estimated to occur in 80% of individuals with Apert syndrome. This is typically a result of external auditory canal stenosis or atresia, middle ear disease, and/or ossicular abnormalities. There is also a high rate of semicircular canal abnormalities (70%) found in affected individuals (Agochukwu, Solomon, & Muenke, 2014).
Waardenburg Syndrome. This syndrome is primarily an autosomal dominant inheritance with four subtypes reported in the literature. However, in some cases, an autosomal recessive pattern has been noted in Types III and IV (National Organization for Rare Disorders, 2015). It is estimated that Waardenburg syndrome occurs in about 1 in 40,000 individuals and accounts for 2% to 5% of congenital hearing loss (U.S. Library of Medicine, 2019k). Types I and II are the most common forms of Waardenburg syndrome, while types III and IV are rare. The following summarizes the key features commonly noted in each of the subtypes along with their associated gene mutations:
Type I: Sensorineural hearing loss; pigmentary abnormalities of the skin, hair, and eyes; as well as dystopia canthorum (lateral displacement of the inner canthi). Mutations in the PAX3 gene.
Type II: Similar features to Type I (sensorineural hearing loss and pigmentary abnormalities of the skin, hair, and eyes) with the absence of dystopia canthorum. Mutations in the MITF or SNAI2 genes.
Type III: Sensorineural hearing loss; pigmentary abnormalities of the skin, hair, and eyes; and upper limb abnormalities. Mutations in the PAX3 gene.
Type IV: Sensorineural hearing loss; pigmentary abnormalities of the skin, hair, and eyes; and Hirschprung disease (an intestinal disorder). Mutations in the EDNRB, EDN3, or SOX10 genes.
While some individuals with Waardenburg syndrome may have normal hearing, hearing loss can often occur. A hearing loss exists in 47% to 80% of individuals with Type I Waardenburg syndrome (Milunsky, 2017). In addition, vestibular dysfunction has been reported in 75% of individuals with this particular classification (Hageman, 1977). Type II has a high penetrance of hearing loss, perhaps as great as 77% to 80% (Milunsky, 2017). Although we were not able to ascertain the penetrance rates/percentages of hearing loss in Types III and IV, it is anticipated that these would also be quite high as these two types of Waardenburg syndromes are reported to represent more severe cases of Types I and II, respectively. The hearing loss in Waardenburg syndrome is often congenital, sensorineural, nonprogressive, and of a moderate to profound degree, and it presents with a variety of audiometric configurations. Asymmetric hearing loss as well as normal hearing can occur, and unilateral or bilateral involvement is possible. Histopathic findings include severely defective or even absent organs of Corti and reduced populations of auditory nerve fibers. Patients typically have different colored irises and a white forelock. Cleft lip and palate exist in 10% of the affected individuals, and the severity of hearing loss may be related to the number of skin and hair pigmentation differences (Reynolds et al., 1995).
Alport Syndrome. Alport syndrome has an incidence of about 1 per 50,000 live births (Kashtan, 2019). This syndrome has an autosomal dominant (5%), an autosomal recessive (15%), and also an X-linked inheritance pattern (80%) (Hood & Keats, 2011). It is caused by mutations in the COL4A3, COL4A4, and COL4A5 genes. There is progressive nephritis with hematuria and proteinuria in the first or second decade of life. Males are more severely affected than females in regard to the disease effects. Craniofacial dysostosis, brachiocephaly, bilateral proptosis, saddle nose, ankylosis, and spina bifida have all been observed in Alport syndrome. Progressive sensorineural hearing loss and vestibular hypofunction can exist, and the hearing loss when present typically begins as a high-frequency, bilateral sensorineural hearing loss (Kashtan, 2019). Due to the progressive nature of the hearing loss, it will often extend into other frequencies. The severity of the hearing loss tends to be greater in men than women. For autosomal recessive and dominant forms of Alport syndrome, the age of onset of the hearing loss is usually in late childhood or early adolescence with equal incidence between males and females. In cases of X-linked inherited mutations, the hearing loss develops in the majority of affected males by the age of 40 years (Jais et al., 2000).
Branchiootorenal Spectrum Disorder. Branchiootorenal spectrum disorder (BORSD) syndrome affects about 1 in 40,000 people (Fraser, Sproule, Halel, & Optiz, 1980; National Organization for Rare Disorders, 2018). The term “branchio” in the name of this syndrome is related to the fact that there is maldevelopment of the second branchial arch in patients with this syndrome. The second branchial arch contributes to tissue on the lateral and front of the neck. In order to be classified as having BORSD, an individual must present with one of the following profiles: three major criteria, two major and two minor criteria, or one major criterion and a first degree relative who has been diagnosed with BORSD (Chang et al., 2004). Major criteria include second branchial arch anomalies, deafness, preauricular pits, auricular malformations, and renal anomalies, while minor criteria include anomalies of the external auditory canals, middle ear, and inner ear; preauricular tags; and other features (facial asymmetry, palate abnormalities). In approximately 90% of the cases, the syndrome is passed on from one affected parent to the offspring in an autosomal dominant inheritance pattern. According to Stickens and colleagues (2001), more than 90% of patients with BORSD present with hearing impairment, including mixed, conductive, and sensorineural losses. Hearing loss in affected individuals can range from mild to profound and can be nonprogressive or progressive in nature (Kemperman et al., 2004). Specific abnormalities of the external ear include preauricular pits and tags, lop ear malformations, atresia, or stenosis. There can be middle ear abnormalities that include malformation, dislocations, or fixation of the ossicles, and malformation of the middle ear space. Inner ear anomalies can include cochlear hypoplasia and enlarged cochlear and vestibular aqueducts (Smith, 2018). There are three gene mutations associated with individuals with BORSD including EYA1, SIX1, and SIX5. EYA1 is believed to be the primary gene mutation and is reported to occur in approximately 40% of individuals with BORSD. While both SIX5 and SIX1 have been found to occur in BORSD, they are far less common. In some individuals, a co-existing EYA1 gene mutation has been identified, and it has been hypothesized to be the actual underlying cause of the condition. The proteins produced by these genes play a critical role in embryologic development and mutations that occur are believed to result in abnormal formation of organs and tissues. It should be noted, however, that there are individuals with BORSD who have none of these three gene mutations, which suggests that there is another unidentified gene (or genes) that can result in this particular syndrome (U.S. National Library of Medicine, 2019b).
Jervell and Lange-Nielsen Syndrome. Approximately 1% of infants with profound sensorineural hearing loss may have Jervell and Lange-Nielsen syndrome (JLNS), which is an autosomal recessive disorder (Hood & Keats, 2011; Tranebjærg, Samson, & Green, 2017). This is primarily a cardiovascular disorder caused by mutations in one of two genes (KVLQT1 and KCNE1) that are potassium channel genes, which lead to disturbances in endolymph homeostasis. This syndrome is characterized by hearing loss and cardiac involvement. The hearing loss typically presents as a profound, congenital sensorineural hearing loss that is bilateral in nature. There is elongation of QT intervals on EKGs. These abnormal QT intervals can result in syncopal attacks and raise the risk of sudden death related to functional heart disease. Carriers of this mutation (parents) may have no hearing difficulties but should be screened for long QT intervals. A family history of sudden death should encourage consideration of this syndrome. Life expectancy is severely shortened if the cardiac condition remains untreated.
Treacher Collins Syndrome. Treacher Collins syndrome has an incidence of about 1 in 50,000 births (Trainor, Dixon, & Dixon, 2009). The mode of inheritance can be either autosomal dominant or recessive. It is caused by a heterozygous mutation in the POLR1D or TCOF1
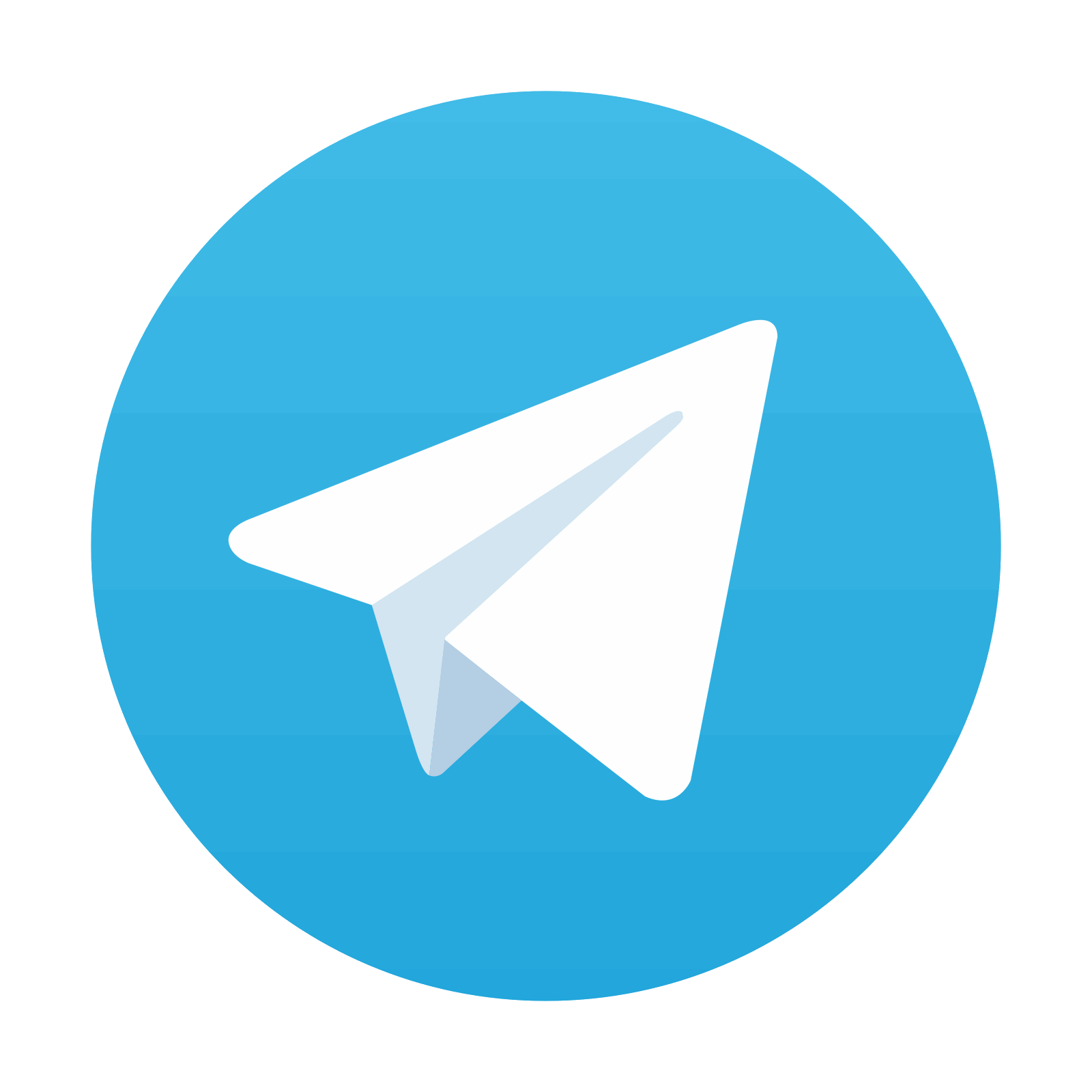
Stay updated, free articles. Join our Telegram channel

Full access? Get Clinical Tree
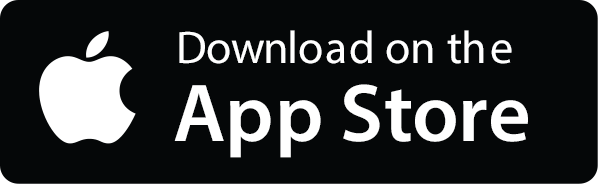
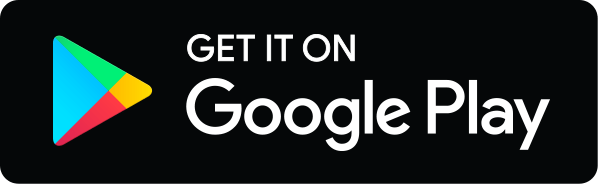