Fig. 11.1
Angioid streaks. Note the “star like” hypopigmented areas surrounding the optic nerve, courtesy of Edward Chaum, M.D. Ph.D.
The pathogenesis of angioid streaks is poorly understood, especially in the setting of sickle cell disease . Clarkson [48] reports that along with sickle cell disease , the most common relationships are with pseudoxanthoma elasticum and Paget’s disease. While they are associated with the hemoglobinopathies, they appear to be a rare feature, occurring in 1–2 % of patients with these diseases. They seem to increase with age and are not in and of themselves associated with vision loss. They have been noted to rarely cause visually significant complications, usually in the form of choroidal neovascular membranes [49]. Since there are breaks in Bruch’s membrane, the vessels from the choriocapillaris are allowed, under certain circumstances, to grow unchecked under the retina leading to hemorrhage and serous retinal detachment. Angioid streaks also cause the eye to be more fragile leading to these eyes being called “egg-shell eyes”. As a result, contact sports should be avoided by patients with angioid streaks [50].
Choroidal Infarctions and Ischemia
Choroidal infarctions and ischemia have been reported. Some believe that they cause the “black sunbursts” seen in the retina. Dizon documented a patient with SS disease who developed amaurosis and had angiographic and clinical evidence of choroidal infarction on two different occasions. She further noted that the incidence of this is not known as it can be asymptomatic leaving only non-specific retinal pigment epithelium (RPE) changes [51].
Retina
Macular complications of sickle cell disease can be acute macular occlusions and chronic macular changes. The macula is the area of the retina responsible for central vision. Acute changes include cotton wool spots (which represent vaso-occlusions of arterioles), “microaneurysm-like dots, dark and enlarged” arteriolar segments, and “hairpin-shaped venular loops”. Many have reported macular infarctions in adult and pediatric patients with sickle cell disease [52–54]. Merritt reported a case of a 10-year-old girl with HbSS that developed bilateral infarctions of the macula with severe vision loss in the right eye (light perception) and mild loss (20/30) in the left. Hydration and transfusion improved left eye vision to 20/15, but her right eye only returned to counting fingers at 3 in. [55].
Chronic changes result from multiple vaso-occlusions of macular vessels leading to an enlarged foveal avascular zone (FAZ) [56]. Since most of these patients retain good vision, Lee studied this phenomenon with fluorescein angiography and Octopus visual field testing (of the central 30°). She found that for these patients the FAZ was indeed abnormal (enlarged) as were their Octopus visual field results [57]. Asdourin did not find corresponding visual field defects with the Goldman or Amsler grid despite angiographic evidence of severe macular changes [56]. Cusick reports a case of bilateral sickle cell macular disease in a patient with SC disease (he notes this is more common in SS disease). The presentation was unusual in that the patient’s chief compliant was “dark spots” in his nasal field. Indeed he did have nasal visual field defects, which are usually considered to be optic nerve related. However, the use of ocular coherence tomography (OCT ) and multifocal electroretinography (mfERG) allowed diagnosis of the retinal vascular pathology [58].
Epiretinal membranes (ERMs) are another rare complication of sickle cell disease . Moriarty [59] found a rate of 4 % in SS and SC patients that were under 60 and had not had previous laser surgery. He found the risks for ERM formation to be proliferative sickle cell retinopathy (PSR), the more extensive, the higher the risk; and vitreous hemorrhage. The risk for ERM formation was lowered by successful treatment of PSR [59]. Clarkson studied 85 patients with sickle cell disease (SS, SC, HbSβthal) ranging from 28 to 123 months and found a prevalence of 1.3 % for macular hole, 2 % for ERM, and 1.3 % for macular pucker [35]. Carney found a rate of ERM formation of 3.7 %, but that included patients with previous laser surgery, a known risk factor for ERM formation. Carney also reports a patient that developed a macular hole after ERM progression. Close observation of patients with ERMs from sickle cell disease is recommended [60].
New technology such as OCT can be especially useful in diagnosing macular pathology from sickle cell disease . Witkin reported a case of bilateral infarction of the branch retinal artery as documented on OCT [61]. Robert reports a 13-year-old female with HbSS disease that developed a central retinal artery occlusion in the left eye and thinning of the nerve fiber layer in the temporal median raphe of the right eye. The girl was asymptomatic and her vision was 20/20. She had stenosis of the internal carotid artery on the right side. The authors postulate that this retinal pathology was due to ocular ischemia [62]. Chow found retinal thinning with corresponding diminished sensitivities on microperimetry testing (measuring the patient’s response to light stimuli at various retinal points followed by superimposing the data on a scanning laser ophthalmoscope (SLO) image). These patients all had good vision. While peripheral retinal pathology is more common, it is important to note that macular disease occurs in patients with abnormal hemoglobin [63].
Non-proliferative Retinal Disease
Venous Tortuosity
Clarkson studied 85 patients with sickle cell disease (SS, SC, HbSβthal) ranging from 28 to 123 months and found an incidence of 11 % for venuous tortuosity. The incidence was 14 % percent in patients with SS disease, 3.4 % in patients with SC, and 0 % in those with HbSβthal [35]. There does not appear to be a correlation with severity of tortuosity and the severity of the anemia . Others have found the rates of vascular tortuosity to vary between 10 and 50 % [64], with rates of 29 % in SS patients and 19 % in SC patients [36] (see Fig. 11.2).


Fig. 11.2
Vascular tortuosity, courtesy of Edward Chaum, M.D. Ph.D.
Salmon Patches and Schisis Cavities
Occlusions of arteries within the retina will rupture the vessel’s walls creating an intraretinal hemorrhage. Within several days, the red blood develops a “salmon” color, hence the name, “salmon patches ”. With continued resolution these may result in a schisis cavity with iridescent spots that represent macrophages containing hemosiderin. Welch and Goldberg, who first used the term, noted that the salmon patch could also become a “black sunburst” which represents migration of retinal pigment epithelial (RPE) cells into the retina after such an event. These misplaced RPE cells have undergone hypertrophy and hyperplasia to create the black retinal scar [12]. Gagliano later confirmed this photographically [65]. Others think the “black sunburst” is actually due to choroidal ischemia or choridal neovascular membranes that occur after choroidal infarction [66, 67]. Perhaps both can lead to these depending on the level of RPE disruption by vaso-occlusive events. In Clarkson’s study of 85 patients (SS, SC, HbSβthal) ranging in age from 28 to 123 months he found a prevalence of 17 % for retinal hemorrhage (RH) and 28 % for schisis cavity/iridescent spots in patients with SC disease. The rates were 2.8 % for RH and 3.7 % for schisis cavity in patients with SS disease and 8.3 % for RH and 0 % for schisis cavity in patients with HbSβthal [35]. Friberg found black sunbursts in 46 % of 92 patients with SS disease, 63 % of eight patients with SC disease and 37 % of ten patients with HbSβthal [36].
Proliferative Retinal Disease
Goldberg was the first to devise a classification system for proliferative sickle cell retinopathy (PSR) based on clinical findings. The following is a brief description of each stage of PSR [68, 69].
- Stage I:
Peripheral arteriolar occlusions
Vaso-occlusive events in the small arterioles of the peripheral retina cause the normal terminus of the retinal vessels to end progressively closer to the macula and thus progressively farther from the ora serrata (the normal end point of retinal vessels).
- Stage II:
Peripheral arteriolar-venular anastomoses
The occluded arterioles fuse with pre-existing capillaries to form a connection with a venule. This forms loops at the terminus of the retinal vessels, which leaves avascular retina between this new endpoint of vascularization and the ora serrata [68]. McLeod found that the arterio-venous anastomoses formed “hair-pin loops” that were the result of new vessel formation between an occluded vessel and a patent one [66] (see Fig. 11.3).
Fig. 11.3
Angiography of a patient with sickle cell retinopathy showing non-perfused retina with early neovascularization, courtesy of Edward Chaum, M.D. Ph.D.
- Stage III:
Neovascular proliferation
New vessels grow toward the area of ischemic retina and look like “sea fans” (Gorgonia flabellum). Fed by one or more arteriole and having one or more draining venule, these can coalesce and become larger (see Fig. 11.4). They often remain flat along the surface of the retina, but may grow into the vitreous causing traction, vitreous hemorrhage (see Fig. 11.5), or tractional retinal detachment. If the traction becomes sufficient, a rent may be created in the retina, causing a rhegmatogenous retinal detachment.
Fig. 11.4
Angiography of a sea fan , with avascular retina peripheral to the area of neovascularization. Courtesy of Edward Chaum, M.D. Ph.D.
Fig. 11.5
Sea fan with hemorrhage , courtesy of Edward Chaum, M.D. Ph.D.
- Stage IV:
Vitreous hemorrhage
- Stage V:
Retinal detachment
Clinical and Angiographic Identification of Stages of PSR
While the above system is based on clinical examination alone, new technology such as non-contact wide-field angiography allows visualization and photography of the retina all the way to the ora serrata in one image. Cho described the use of non-contact wide-field angiography in the diagnosis of six patients (12 eyes) with significant peripheral retinal changes. These were not noticed clinically in 25 % of the eyes, they were only detected on wide-field angiography [70].
Management/Treatment
Untreated sickle cell retinopathy has been reported to cause vision loss (20/60 or worse) in 10 % of patients. Half of these were legally blind at 20/200 or worse. Almost all vision loss was attributable to proliferative disease. This was almost always caused by epiretinal membrane, traction retinal detachments (although one patient developed a rhegmatogenous detachment) or vitreous hemorrhage. Angioid streaks [leading to choroidal neovascular membrane (CNV)], sickling maculopathy and branch retinal artery occlusion (BRAO) were less frequent causes of vision loss in non-proliferative disease [71]. Proliferative retinopathy (and retinopathy in general) is more common in SC disease and HbSβthal than SS disease [72].
Clarkson found the prevalence of proliferative retinopathy to be 18 % when all three groups (SS, SC, HbSβthal) were combined. If each group was examined independently, SS patients had a prevalence of 11 % and HbSβthal patients had a prevalence of 17 % [35]. He found a prevalence of vitreous hemorrhage of 21 % in patients with SC disease and 1.8 % in patients with SS disease. Retinal detachment was seen in 10 % of patient with SC disease. The incidence of PSR increased in patients over 40 years. While PSR is less common in children than in adults, it can occur and be vision threatening. Clarkson also reports an 11 year old that had Stage III disease in one eye and non-proliferative retinopathy in the other on initial exam. He eventually lost all vision in the more severely affected eye due to unsuccessful surgery to repair a retinal detachment.
Friberg found in patients with SS disease from 8 to 20 years old (34 patients) that the incidence of peripheral vascular occlusions was 9 %, arteriovenous anastomoses was 2 % and vitreous hemorrhage was 3 % [36]. However, Hayes did not find any proliferative retinopathy in patients with SS disease who were under 19 years old [73].
Penman looked at peripheral retinal changes angiographically in children with sickle cell disease in the hopes of stratifying risk for development of PSR. He classified type I as “fairly” normal peripheral retinal vasculature, with continuous arteriovenous loops. Type II did not have continuous loops and gave the appearance of “moth-eaten” vasculature. While few patients in his study developed PSR, all that did had Stage II disease prior to its development. He therefore concluded that this method of assessing angiography could be used for screening for the development of PSR [74]. Interestingly, all but one of the patients that developed PSR had SC disease [74].
Eruchalu looked at 37 patients under 13 years old who had severe systemic SS disease. “Severe” was defined as three or more vaso-occlusive events in 1 year. Thirty-two percent had retinopathy and three eyes of two patients had proliferative disease. All of these patients were 8 years old or older. After one year, one had received laser treatment and the other was stable. They recommend yearly screenings after age 8 in this high-risk group [75].
Goldberg found a tendency toward progression of PSR in 25 eyes of 16 patients with SC disease, although only one lost vision [76]. In Clarkson’s study, only three patients had 20/30 or less vision on presentation and only five developed vision loss over the 6 years of follow-up [35]. Condon found that 61 % of PSR lesions autoinfarcted in his study of sickle cell patients followed over 8 years. He noted that the fastest progression of PSR lesions occurred in patients 15–29. Only, 12 % of eyes lost vision due to PSR. While many lesions autoinfarct, others go on to cause vitreous hemorrhage, retinal detachments, and vision loss [77].
Since a significant percentage of cases of PSR autoinfarct (20 % according to Nagpal) [77, 78], the treatment of PSR is somewhat controversial. Usually treatment is not indicated until active neovascular proliferation (Stage III) has occurred in both eyes, or rapid progression of PSR, or proliferative disease in patients with only one remaining eye [79]. Historically, laser occlusion of the feeder vessel to the proliferation was employed. However, this was fraught with complications, including breaks in Bruch’s membrane, choroidal neovascular membrane development, choroidal ischemia, retinal detachments and vitreous hemorrhage [80, 81]. More recently panretinal photocoagulation, either sector or 360° treatments, has been utilized (see Fig. 11.6). Farber reported on 174 eyes in 116 patients randomized to either scatter photocoagulation (99 eyes) or observation (75 eyes). In the treatment group, 30 % achieved total resolution and 51 % partial resolution. In the observation group 22 % and 24 % achieved total and partial resolution, respectively. The treated eyes had slightly less visual loss (3 %) than did the control group (8 %) [82]. Fox reported a series of 88 patients with SC disease that were randomized to observation or sector laser photocoagulation. There was a higher rate of regression of neovascularization (NV) in the treatment group, although only a few in both groups had complete resolution of NV. If the patient was younger than 25 years old and NV was flat and small, there was a better chance that the lesion would infarct [81]. Sayag noted that Stage III was more common in SC disease and in the 20–31 year olds. Lesions in patients in their 40s tended to remain stable [83]. Condon reports that progression of PSR is more common in younger patients (15–29 years) and autoinfarction more common in older ones (29 and older) [77]. Fox also found progressive disease only in patients under 40. He also noted higher rates of autoinfarction in patients over 40 and in patients with SS disease. This would suggest the need for more aggressive management of younger patients and a tendency to observe in patients 40 and over, especially in the setting of SS disease [84].


Fig. 11.6
Regressed sea fan after laser treatment (panretinal photocoagulation), courtesy of Edward Chaum, M.D. Ph.D.
Sayag classified sea fans (Stage III PSR) in order to attempt to stratify risk of progression. He defined the following: Grade A: “sea fan with leakage less than 1 Macular Photocoagulation Study (MPS) disc area” on fluroscein angiography; Grade B: “elevated sea fan with hemorrhage”; Grade C: “elevated sea fan with partial fibrosis”; Grade D: “complete sea fan fibrosis without well demarcated vessels”; Grade E: “Complete sea fan fibrosis with well demarcated vessels”. He identified 73 eyes of 101 patients with PSR and randomized them to scatter laser photocoagulations with argon green laser (38 eyes) or observation (35 eyes). He found that Grades A and C did not do statistically better with treatment while Grade B did. He further found that the only complications that occurred were in patients that were untreated and belonged to Grades B and E. Four developed vitreous hemorrhage and three developed rhegmatogenous retinal detachment [83].
While laser photocoagulation is the usual modality for the treatment of Stage III PSR, Seiberth reported a case of successful trans-scleral diode laser photocoagulation for the treatment of PSR. In this particular case, there was a vitreous hemorrhage, obscuring the view of the retina, so traditional laser treatment was not an option [85].
Vitreous hemorrhages usually remain localized over the site of neovascularization. They can, at times, spread over the macula and interfere with vision [69]. If vision is affected, the patient is usually followed for 6 months to see if the vitreous hemorrhage will clear. If it does not or a retinal detachment occurs, surgery is performed. However, both systemic and ocular complication rates are higher in patients with sickle cell disease . Therefore, careful consideration of the risks and benefits as well as proper pre-operative care of the patient are required [86].
The need for surgical treatment of Stage V sickle cell retinopathy (retinal detachment) is rare in children, however it is indicated at times. The techniques have improved over the years. Anterior segment ischemia was a dreaded complication of retinal buckling procedures. Ryan and Goldberg [87] reported six of ten eyes in patients with SC disease that developed anterior segment ischemia after scleral buckling for retinal detachments. Four eyes became phthisical or prephthiscial, while the other two regained 20/20 or 20/25 vision [87]. Some advocated transfusion prior to such surgeries and even then had significant post-operative complications. Newer vitrectomy and buckling techniques have been employed successfully , without anterior segment ischemia. Pre-operative transfusion is no longer recommended routinely. Intraoperative attention to adequate hydration and oxygenation by the anesthesiologist and good control of intraocular pressure by the surgeon seem to limit the rates of this complication [88]. Chen looked at 15 eyes of 14 patients that underwent retinal surgery for sickle cell eye disease: six for vitreous hemorrhage, one for ERM, and eight for retinal detachment. All had local anesthesia. All had vitrectomy, and two additionally had retinal bands placed. While four patients in the retinal detachment group developed another detachment and required further surgery, none developed anterior segment ischemia. Visual results were good in the vitreous hemorrhage and ERM group (20/60 or better), and as expected in the retinal detachment group (20/400 or better) [88].
Williamson reported the results of 27 patients with PSR, ERM, or vitreous hemorrhage. For reasons not fully explained, 10 of them were observed. One had an inoperable retinal detachment. Some (no number given) were vitreous hemorrhages that cleared and some had traction retinal detachments that did not progress. Two resolved, one with a rhegmatogenous retinal detachment that spontaneously flattened and one with an ERM that resolved with posterior vitreous detachment development. None of the patients had pre-op laser surgery because the author believed it would increase the risk of anterior segment ischemia. There were no reports of this in any of the patients studied. Of the 18 eyes of 17 patients who had surgery, iatrogenic retinal tears developed in seven, vitreous hemorrhages in two (one requiring more surgery), and cataracts in three. Eighty-three percent had improved vision after surgery or surgeries [89].
Leen reported a case of anterior segment ischemia after vitrectomy. The patient had hypotension during surgery and intraoperative laser was employed, possibly contributing to the complication [90].
The role of bevacizumab in the treatment is still being elucidated. Several case reports have noted regression of neovascularization in patients with PSR [91, 92], but one noted a hyphema after injection [93].
Central retinal artery occlusion is a rare complication of sickle cell disease . Liem reports three cases in patients with SS disease. In his review of the literature, no other types of hemoglobinopathy have been reported to have CRAO. His patients, as well as most in his review, were treated with exchange transfusion resulting in 71 % recovering some or all of their vision [94]. Fine reported a case of spontaneous CRAO in a patient with sickle cell disease . This case was also remarkable because vision improved, without treatment, to 20/60 [95]. Brown reports one case of a 14-year-old patient with SS disease who developed a central retinal artery occlusion during an acute pain crisis. No further details are given [96].
Optic Disc
The phrase “disc sign of sickling hemoglobinopathies” refers to dark red spots or “curlicue” vessels on the optic nerve head. They are transient occlusions of the small vessels of the disc, analogous to those seen in the conjunctiva. Like the conjunctival sign, the disc sign is more frequent in patients with SS disease. Goldbaum found a rate of 11 % in 80 patients studied. The group consisted mostly of patients with SS disease [97]. Clarkson found a prevalence of 12 % in patients with SS disease. No one in the SC or HbSβthal group was noted to have this [35]. Neither author reported visual sequela from these vascular changes. Neovascularization of the optic disc has been rarely reported in hemoglobinopathies [64].
Henry described three cases of idiopathic intracranial hypertension in three patients with sickle cell disease . One patient had SC disease and two had SS disease. They were all successfully treated with acetazolamide without any complications, either systemic or ocular [98].
Ophthalmic Complications of Sickle Cell Trait
While patients with sickle cell trait have been reported to develop ocular complications, it is usually in the setting of concomitant systemic disease or ocular trauma [69, 99–102]. Taban reported a case of frosted branch angiitis in a 4-year-old girl with sickle cell trait [103]. Lim reported an adult patient (58 years old) with sickle cell trait and hypertension who developed a superior ophthalmic vein thrombosis. The patient had orbital pain, diplopia, 4 mm of proptosis and 20/25 vision. Oral warfarin was given and the patient’s condition was fully resolved with 20/20 vision 2 weeks later [104]. Wax and coworkers reported a case of a 14-year-old male with traumatic hyphema that developed IOPs from 28 to 43 mmHg. Nine days after injury his vision decreased to 6/60 and his IOP was 36 mmHg. There was acute cupping of the optic nerve and retinal whiting with a “cherry-red spot ”. Angiography and electroretinogram (ERG) confirmed an impending CRAO. An emergent paracentesis was performed and the patient’s vision improved to 6/15 9 days afterward. The cupping resolved as well. The authors used acetazolamide and isosorbide in the initial management of the glaucoma. However, they advocate not using these modalities as they may have contributed to further sickling, increased IOP , and retinal occlusion [105]. Brown reported two patients with sickle cell trait who developed CRAO: one was a 9 year old who developed a CRAO after ocular trauma (no further details are discussed) and the other was a 24-year-old male with rheumatic heart disease in addition to sickle cell trait [96].
Hedreville reported a case of a 26-year-old cyclist with sickle cell trait who developed a central retinal vein occlusion (CRVO ) shortly after a cycling race in a very hot environment. The patient had noted a sudden loss of vision a few months prior that resolved within 2 days. At the time of CRVO development, he also had four blood clots in the left atrium of his heart. This lead the authors to believe that the intense exercise, dehydration, and hot environment caused hemodynamic changes significant enough to cause clotting in this patient with “just sickle cell” trait. The patient developed neovascular glaucoma and despite aggressive treatment developed total retinal detachment and bare light perception vision [106].
Barry reported a case of a 3.5 year-old boy with sickle cell trait who presented with leukocoria and esotropia in the left eye due to vitreous hemorrhage. Ischemic changes were noted in the right eye. Vitrectomy and photocoagulation were performed on the left eye and vision returned to 20/32 [107]. Jackson reported three cases of retinopathy in patients with sickle cell trait: one was a 14-year-old boy who developed salmon patches and peripheral vascular occlusions after traumatic hyphema with increased IOP ; and the other two patients were adults with glaucoma [108].
As mentioned in the anterior chamber/glaucoma section, patients with sickle cell trait are at increased risk of complications after traumatic hyphema . Please see that section for further details and treatment recommendations .
Diagnosis
A child with sickle cell disease will be asymptomatic at birth due the presence of fetal hemoglobin, which is protective; however, anemia and reticulocytosis develop during the first months of life due to the physiologic switch to adult hemoglobin. The classic blood smear occurs after 3 years when the majority of hemoglobin is the adult form [1]. In the United States, screening is performed in the “newborn screen” on all children born in a hospital. The majority of screening programs use isoelectric focusing (IEP) of an eluate from dried blood spots and some utilize high-performance liquid chromatography (HPLC) or cellulose acetate electrophoresis as the initial screening method. Confirmatory testing with complementary electrophoretic methods should be performed in abnormal initial tests. Solubility tests such as the “sickle prep” or “sickle dex ” are inappropriate screening or confirmatory assays, in part because high levels of fetal hemoglobin (thus low levels of HbS) give false negative results in infants with sickle cell anemia.
Diagnosis of sickle cell retinopathy, before it becomes visually devastating, requires screening. Screening for sickle cell retinopathy in adults is yearly, sooner if complications arise. In children, the recommendations are a bit less clear. Gill recommends starting screening for sickle cell retinopathy at age 9 for patients with SC disease and 13 years for patients with SS or S β Thalassemia [109]. Others suggest beginning screening at age 10 for all these patients [110], while Eruchalu recommends screening yearly after 8 years old [75]. There is also disagreement about follow up exams. Gill recommends yearly exams [109], while others recommend yearly for SC disease and every 2 years for SS and S β Thalassemia.
Management
Hydroxyurea is a commonly used medication in sickle cell disease . It increases the proportion of fetal hemoglobin and decreases dense cells. It also increases mean corpuscular value and mean corpuscular hemoglobin [1]. These changes reduce complications normally associated with hemoglobinopathies. While guidelines exist, initiation and management of this medication should occur in consultation with a hematologist. Of note, ocular complications of hydroxyurea have rarely been reported. Limbal stem cell deficiency with eye pain, photophobia, corneal ulceration, opacification and loss of vision have been documented in patients receiving this medication [111, 112].
Anemia is a common complication of sickle cell disease . This and other indications create the need for chronic transfusions. In such patients, iron overload can lead to other complications, namely liver dysfunction. To avoid these complications, iron chelation therapy is often employed with deferoxamine (Desferral) and Deferasirox (Exjade). Desferoxamine has been reported to cause a myriad of ocular complications including optic neuropathy and retinopathy [113, 114]. Deferasirox is also thought to cause similar complications although there is scant evidence of such in the literature [115, 116].
Care of Pre-operative Patient
Patients with hemoglobinopathies are at higher risk of systemic and ophthalmic complications. Adequate pre-operative hydration, consideration of transfusion, and adequate oxygenation during the procedure are needed. An 11-year-old girl with SC disease died following evisceration after orbital trauma, proving adequate pre-operative and intra-operative care must be achieved even in the emergency setting [117]. Pre-operative consultation with hematology and anesthesiology is needed.
Prevention of Ophthalmic Disease
Hydroxyurea holds promise for decreasing the incidence of retinopathy in childhood. Estepp observed 123 children (under 19 years) with SS disease. Lower hemoglobin F levels were associated with an increased incidence of retinopathy. Additionally, 72 of the 123 children started hydrocarbamide (hydroxyurea) during the study. Of those, 8 % developed retinopathy while 14 % did so in the untreated group. While not a statistically significant difference, the treatment holds promise for preventing eye disease in children with sickle cell disease [15].
Leukemia
Definition
Ocular involvement by leukemia has long been recognized [118]. Leukemia is a neoplasm of the leukocytes (white blood cells). The prognosis for childhood leukemia has improved enormously since the 1970s [119] due to improved chemotherapy, new understanding of the natural history of the disease, bone marrow transplantation and better supportive care of the patient [120].
The leukemias are classified into acute and chronic forms as well as lymphoid and myeloid cell types. The classification is based on the cell type and maturation. In general, acute leukemias have more primitive appearing cells. The clinical course of the acute and chronic leukemias is also quite different, with acute leukemia having a relatively rapid and relentless deterioration unless treated aggressively.
The cells of acute lymphoblastic leukemia (ALL) are derived from the lymphocyte cell line. Acute and chronic myleoblastic leukemias (AML and CML, respectively) are derived from primitive myeloid cells [119]. There is increasing use of genetic evaluations to determine prognosis and subtypes of ALL and AML [121].
History
Leukemia was initially thought to be a suppurative disease of the blood [122]. With the advent of formal histopathology in the nineteenth century, leukemia was recognized to be a malignancy of leukocytes. Prior to the advent of bone marrow biopsy, Liebreich in the 1860s [123] recognized that the fundus examination was helpful in making the diagnosis of leukemia [118]. The study of Ridgway and coworkers was the first to review leukemic ophthalmopathy in children [124].
Epidemiology
The leukemias are the most common malignancies of childhood. It is estimated that approximately 3250 new cases are diagnosed each year in the United States in children less than 20 years old [119]. Of childhood leukemias, ALL makes up 75 %, AML accounts for 15–20 %, chronic myeloid leukemia makes up less than 5 %, and chronic lymphocytic leukemia is virtually never seen in children [120]. ALL occurs at a rate of 29.2/1 million while AML occurs at a rate of 7.6/1 million in children less than 20 years old [119].
Systemic Manifestations
Common presenting signs of ALL are fever, hemorrhage, bone pain, lymphadenopathy, hepatosplenomegaly, splenomegaly, mediastinal mass, and central nervous system (CNS) disease [120, 125]. Clinical features of childhood ALL at the time of presentation depend on the extent of disease. An elevated white blood cell count may cause cerebrovascular accident, renal failure, retinal cotton wool spots, or mild respiratory distress. Central nervous system involvement, while occurring in both AML and ALL is more common in ALL. Distinctive presentations of AML are granulocytic sarcomas or chloroma (tissue infiltration of leukemic cells) seen principally in the orbit, spinal cord or skin, which may precede the development of frank leukemia . Gum hyperplasia and disseminated intravascular coagulation may also be seen in AML [126]. Loss of weight is a common non-specific feature at the time of presentation [127].
Many of the systemic features of established leukemia are the consequences of therapy [121]. Infections (viral, bacterial, and fungal) are common and reflect the compromised state of the body’s immune mechanisms. Doxorubicin (Adriamycin ) is known to cause cardiac toxicity. High doses of intrathecal chemotherapy in association with cranial irradiation (as used for early CNS prophylaxis) may cause a late leukoencephalopathy. Graft-versus-host disease is a serious complication of bone marrow transplantation and has significant ocular, dermatological and gastrointestinal effects.
The prognosis for both ALL and AML has improved dramatically since the 1970s [119]. Five-year disease-free survival is reported to be approximately 80 % for ALL and 41 % for AML [119], although this is affected by age and other risk factors. Infection and the complications of hemorrhage are the usual cause of death.
Diagnosis
While blood counts are often abnormal, bone marrow aspiration is necessary for diagnosis of any leukemia . Blast cells must make up more than 20 % of the bone marrow for the diagnosis to be made. Cytogenetics and other diagnostic testing such as FISH, PCR, microarray, and spectral karyotyping are often used to further define the disease and tailor treatment [120].
Management
A detailed discussion of the treatment of leukemia is beyond the scope of this book. For such a discussion, the reader should consult a standard text such as the chapter by Margolin and coworkers in Cancer: Principles and Practices of Oncology [120].
The principles of treatment are the induction of disease remission and the maintenance of this disease free state. This involves multi-agent (combination) chemotherapy and CNS prophylaxis (both intrathecal chemotherapy and craniospinal irradiation) [121]. Supportive care involves the use of blood products to treat anemia and clotting disorders, the detection and treatment of infection, metabolic and nutritional support and psychosocial care of the patient and family [121]. Bone marrow transplantation (BMT ) is an important treatment modality especially in the management of AML and high risk or refractory ALL [128].
With increasing overall cure rates for most children with ALL and AML, considerable research efforts have been expended to devise the most effective therapies with the least associated morbidity. Attention has been directed towards identifying high-risk subgroups and designing more aggressive treatment protocols for these groups in an attempt to improve overall survival.
Ocular Side Effects of Medications Used in the Treatment of Leukemia
Steroids are a mainstay in the treatment of leukemia . Oral, intravenous, and topical steroids have all been shown to cause increased intracranial [125] and intraocular [129] pressures in susceptible individuals. Blurry vision, headaches, nausea, and vomiting are common features of both conditions, but can also be side effects of many medications. A thorough ophthalmic exam is indicated if any of these complaints arise in a leukemic patient in active treatment in order to rule out these conditions. A more thorough discussion of increased intracranial pressure from steroid use is found in the optic nerve portion of this section. Ocular hypertension from steroid use can usually be controlled with anti-glaucoma drops while the patient is on high-dose steroids [129]. It is possible that surgical management may be needed .
Other medications commonly used in the treatment of leukemia are known to cause ocular complications. Vincristine is known to cause neuropathies, including those of CN II, III, IV, V, VI, and VII [130]. CN II would cause an optic neuropathy. CN III, IV, and VI would cause motility disorders and misalignments of the eye. CN V would cause decreased sensation of the cornea that can lead to break down and ulceration of the cornea. CN VII would cause inability to full close the eye as well as decreased facial tone. This could lead to compromise of the cornea depending on severity. These almost always resolve with cessation of the medication. Cyclosporine has been associated with idiopathic intracranial hypertension [131] and retinal toxicity [132]. Busulfan is implicated in the development of cataracts, especially posterior subcapsular cataracts [130]. Radiation and steroids are also known causes of cataracts [132]. Cytosine arabinoside (Ara-C) causes a toxic conjunctivitis and keratitis [133] and warrants pre-treatment with topical artificial tears and steroid eye drops.
Bone Marrow Transplant
Bone marrow transplantation (BMT ) has become a more common treatment for leukemia , especially for AML. Bone marrow transplantation leaves the patient severely immunocompromised causing them to be at increased risk of the infections described in the following retina section [134]. Bone marrow transplantation can cause mild to severe dry eye [135] and cataracts [136]. Graft-versus-host disease (GVHD ) can develop after BMT and increases the chances of patients developing ocular GVHD . Ocular GVHD can cause very severe dry eye, conjunctival scarring, and possibly keratopathy and corneal scarring. Aggressive lubrication, topical and systemic steroids, topical and systemic immunosuppressants (cyclosporine and tacrolimus), autologous serum or albumin, punctual plugs, bandage or even scleral contact lenses have been employed in the treatment of this very difficult ocular disease process [136].
Central Nervous System Leukemia
Clinical Features
The symptoms and signs of CNS leukemia are the result of direct leukemic infiltration into the CNS leading to meningeal irritation and raised intracranial pressure [137]. The major symptoms of CNS leukemia in descending order of frequency are nausea and anorexia, lethargy, vomiting, headache, seizures, coma, visual blur or diplopia. The major signs (also in descending frequency) are papilledema, tendon reflex changes, facial nerve palsy, positive Babinski sign, nuchal rigidity, pupillary changes, and sixth nerve palsy [137].
Patients with CNS leukemia will rarely present directly to the ophthalmologist. However, ophthalmic opinion should be sought to elucidate the cause of visual symptoms and to confirm findings of papilledema, pupil abnormalities and CN VI palsy. There have been several reports of children presenting with CNS leukemia as their initial manifestation of leukemia [138–141]. Two presented with sudden development of strabismus. One also had ataxia and papilledema [138] the other had bilateral ptosis, sluggish pupils, and upgaze paresis [139].
Diagnosis
In any child with CNS abnormalities and a history of leukemia , CNS involvement should be considered. The diagnosis is confirmed by the finding of blast cells in the cerebrospinal fluid (CSF) [120].
Differential Diagnosis
Meningitis (bacterial or viral) may present with similar ocular symptoms and signs as those of CNS leukemia . Various antileukemic medications have side effects that may be confused with CNS leukemia . Chief amongst these are corticosteroids, methotrexate and vincristine. Pseudotumor cerebri or idiopathic intracranial hypertension syndrome secondary to corticosteroid use is well recognized [142]. Intrathecal methotrexate has been noted to cause optic neuropathy and internuclear ophthalmoloplegia [142]. This effect is potentiated by concurrent cranial irradiation. Vincristine has been noted to cause cranial nerve palsies, optic neuropathy (and subsequent optic atrophy), night blindness, and transient choroidal blindness [142].
A temporal relationship of the onset of symptoms and signs to the use of a specific therapy may implicate medication as a cause. CSF studies are very helpful in differentiating the various causes of newly acquired neurologic dysfunction in leukemic patients.
Incidence
Prior to the development of antileukemic chemotherapy in 1948, meningeal or CNS leukemia was rarely diagnosed. Shaw and coworkers reviewed the literature in 1960 and found that only 31 cases were reported prior to 1948 while over 100 cases were reported in the 10-year period from 1949 to 1959 [137]. Later CNS prophylaxis resulted in a reduction of the number of cases of CNS leukemia . Currently less than 10 % of children with leukemia develop CNS leukemia [143].
Natural History
Untreated, the natural history of CNS leukemia is one of continued neurologic dysfunction, coma, and death. Early reports of treatment reported little success and the prognosis for survival was considered poor [137]. Recently the rate of CNS relapse has been reported to be between 2 and 10 % [144]. The long-term prognosis is still guarded; though in more than 90 % of patients, remission for 1–2 years is obtained [143]. Long-term event-free survival has been reported to be 70–80 % with the more recent strategy of delaying radiation for 6–12 months while intensive chemotherapy is administered [144].
Treatment
There have been numerous randomized trials undertaken in an attempt to determine the optimal treatment obtained for overt CNS leukemia [143]. In most cases remission can be obtained with either intrathecal chemotherapy and/or craniospinal irradiation. Without some form of maintenance therapy, however, this remission is generally short-lived.
Ophthalmic Manifestations of Leukemia
The effects of leukemia on any tissue may be primary or secondary. Leukemic infiltration is an example of a primary effect while hemorrhage secondary to thrombocytopenia is a secondary effect. This distinction becomes readily apparent when considering the ophthalmic manifestations of leukemia .
The incidence of various sites of leukemic ocular involvement depends upon the method of ascertainment and the stage of the disease in which the examination is made. Pathological studies consistently show higher incidence of eye involvement than clinical series. This is almost certainly because these are autopsies with a large number of patients dying of disseminated disease. Pathological examination often detects leukemic involvement that is clinically silent. Choroidal leukemic involvement, which is generally cited as the most common site of ocular involvement in autopsy series, is a good example of this phenomenon [145, 146].
Ocular involvement is detected in approximately 30–80 % of autopsy eyes [145–148]. In a prospective study of 120 patients examined at the time of initial diagnosis, only 3 % were found to have primary ocular involvement (retinal infiltrates). Thirty-nine percent had secondary manifestations (hemorrhage, cotton wool spots and central retinal vein occulsions secondary to hyperviscosity). The authors of this study acknowledge that choroidal infiltration may easily have been missed [149]. Reddy and Menon examined 82 children with acute leukemia within two days of diagnosis and found that three had ocular symptoms, while 14 (17 %) had ocular signs related to leukemia [150].
There have been several excellent reviews of the ocular manifestations of leukemia [124, 145, 146, 148, 151]. The most recent series of ophthalmic manifestations of leukemia have focused on ocular involvement of leukemia within two days of diagnosis. Reddy and co-workers found that 17 % of newly diagnosed children with acute leukemia had ocular changes related to their disease [150]. Ohkoshi reported that of 28 patients found to have ophthalmic manifestations of acute childhood leukemia , 27 died within 28 months of the diagnosis of eye abnormalities [152]. All 28 patients had either CNS or bone marrow relapse at the time of diagnosis of ocular involvement. They further reported that the 5-year survival was reduced by half (from 45.7 to 21.4 %) if there was ocular pathology [152]. However, Somervaille and colleagues reported 17 cases of ocular relapse (as a first relapse) in ALL. Eight of them had a relapse in the CNS or bone marrow at the time or ocular relapse. All were treated with chemotherapy and radiation. Eleven of those were still alive and in remission over 4 years after diagnosis of relapse. These authors note that more aggressive treatment at the time or relapse seems to improve survival. All of the 11 survivors were treated with intense relapse protocol chemotherapy with radiation to the involved eye(s). If treated less intensely, all but one expired and the one survivor was in chronic relapse at the time of publication of the study. This suggests that aggressive treatment is warranted in the setting of ocular involvement at the time of relapse [153].
Orbit
Clinical Features
Clinically significant orbital infiltration with leukemia usually presents with proptosis, lid edema, chemosis and pain [146]. ALL and AML may infiltrate the orbit although AML does so more often [154–158]. The orbital mass seen in myeloid leukemia (AML) is known as a granulocytic sarcoma (GS or chloroma). These were so named because of a greenish color due to myeloperoxidase seen in two-thirds of specimens [159]. AML may present with orbital or spinal cord “chloromas”.
The proptosis caused by orbital infiltration may cause displacement of the eyeball in any direction. Retinal striae may be observed if there is compression of the globe. Specific orbital structures, such as the lacrimal gland or extraocular muscles, may be infrequently infiltrated by leukemic cells [130, 160]. Compression of the optic nerve at the orbital apex may result in sudden loss of vision.
Diagnosis
A clinical diagnosis may be made readily with MRI imaging in a child know to have leukemia . The homogenous mass molds to bony walls of the orbit without destruction. It is isointense to brain and muscle on computed tomography (CT, unenhanced), isointense to gray matter and muscle on T1-weighted MR and isointense to muscle and white matter on T2-weighted MR [158]. If it is not possible to reach a diagnosis on the basis of clinical findings, imaging studies and systemic assessment followed by orbital biopsy is required [130].
Differential Diagnosis
The differential diagnosis includes the causes of rapidly expanding orbital masses. Entities that should be considered are orbital inflammatory conditions [such as orbital cellulitis (bacterial or fungal) and pseudotumors] and tumors [such as lymphoma (including Burkitt’s lymphoma), rhabdomyosarcoma, and neuroblastoma] [163].
Orbital and preseptal cellulitides are the most common cause of orbital inflammation in childhood. Leukemic patients are more prone to such infection because of the effects of their disease and systemic chemotherapy on their immunocompetence. Sinus disease either due to direct leukemic infiltration [130] or infection may also give rise to signs and symptoms of orbital inflammation. Infection with unusual organisms, such as Aspergillus and Phycomycetes (including Mucormycosis), needs to be considered in immunocompromised patients [164]. McCarty et al., reported seven cases of fungal cellulitis in pediatric cancer patients, all of whom presented with fever and neutropenia. Four patients had upper eyelid edema, one complained of headache, and one noted facial pain. One additional patient was discovered to have fungal orbital cellulitis incidentally on CT imaging. Despite aggressive medical and surgical management, five of the seven patients died. The authors advocate that even subtle signs of preseptal cellulitis in the immunocompromised patient should be treated with antifungal therapy in addition to antibiotics. This should be done while work up is initiated. Since the immunocompromised patient cannot mount a normal inflammatory response, even subtle signs of infection should be fully investigated and treated empirically [165].
Orbital lymphoma is rare in children. Before this diagnosis is made granulocytic sarcoma should be excluded using the appropriate histochemical stains [163]. Neuroblastoma may present with rapidly growing orbital metastases, with or without the characteristic “raccoon-eye” periorbital hemorrhage and involvement of the lateral orbital wall.
Incidence
Kincaid and coworkers reported a 7.3 % incidence of orbital involvement in acute leukemia in an autopsy series of 233 cases [130]. The majority of these cases were diffuse infiltrations of orbital tissue and not clinically significant.
Granulocytic sarcomas of the orbit were thought to be rare in Caucasian children. In a group of 257 children with proptosis reported by Crawford, 2 % were due to granulocytic sarcoma [166]. Porterfield reported a series of 214 children with orbital tumors in which 3 % had GS [167]. In contrast Cavdar reported a 27 % incidence of granulocytic sarcoma in a series of 121 with AML from Turkey [157]. However, more recently, Bidar reported 17 of 27 children with GS who were white [158]. Most series document a higher incidence in males than females [157, 158, 163, 168].
Natural History
Granulocytic sarcoma may present at the same time as the hematological malignancy though some studies show that it precedes the onset of the leukemia [169]. Zimmerman and Font reported 33 patients with GS [163]. Four of these patients developed GS late in the course of established AML, while 29 presented with GS and went on to develop leukemia . Most develop AML less than 1 year after diagnosis of GS [163], although longer intervals have been reported [170]. Bidar reports on 23 of 27 children that were diagnosed with orbital disease after their leukemia diagnosis [158].
Cavdar and Zimmerman both reported orbital leukemic involvement as a poor prognostic indicator. However, Bidar’s survival rates were considerably higher and were approximately that of the underlying disease. The majority of their patients were already diagnosed with leukemia so the diagnosis of orbital leukemic involvement was made earlier. They also state that since their report was on more recently treated patients, advances in leukemic treatments likely played a strong role in the higher survival rates in their patients [158].
Treatment
Orbital leukemic infiltrates respond well to systemic chemotherapy. If there is massive infiltration or compression of the optic nerve secondary to mass effect, local irradiation is indicated [157, 158, 171]. Radiation may also be indicated if systemic chemotherapy is ineffective in treating the orbital disease [158].
Lacrimal Apparatus and Lids
Leukemic infiltration of the lacrimal gland is occasionally noted [130, 172]. Leukemia rarely may present with lacrimal gland enlargement [130]. Reports of such have been limited to adults, most with the chronic forms of leukemia .
Leukemic dacryocystitis has been reported in adults with chronic lymphocytic leukemia [130, 172–174]. Dacryocystectomy [174] and dacryocystorhinostomy [130] have been undertaken to confirm the diagnosis and treat the symptoms.
Reddy reported a 15-year-old male whose presenting sign was an abscess of the left upper eyelid. Dilated retinal examination revealed dilated and tortous venules in both eyes as well as cotton-wool spots of the left retina [175].
The lids may be swollen and occasionally infiltrated by leukemic cells in association with orbital infiltration. Rarely, isolated leukemic involvement of the skin (leukemia cutis) is noted and occurs on the lids [130].
Conjunctiva
Clinical Features
Subconjunctival hemorrhage is seen in acute leukemia due to hematologic abnormalities [175, 176]. Conjunctival infiltration by leukemia is more commonly identified post-mortem than ante-mortem. Allen and Straatsma noted conjunctival involvement in 18 % of their series [145] and Kincaid and Green reported a 4 % incidence [130]. Ante-mortem recognition of leukemic infiltration of the conjunctiva is a rarity and most cases have been in adults with either AML or CML [177–180], although Lo Curto reports one child who presented with conjunctival infiltration that on biopsy proved to be leukemia [181].
Diagnosis
Biopsy is required to make a definitive diagnosis, as there are no distinctive clinical features of leukemic infiltration of the conjunctiva [179]. The differential diagnosis includes: allergic/vernal conjunctivitis, sarcoidosis, and other causes of papillary conjunctivitis.
Treatment
The reported literature gives little guide as to the most appropriate treatment for conjunctival infiltration by leukemia . In one report the conjunctival changes responded to systemic chemotherapy alone [177]. While in another, radiotherapy was also required. Local measures may be required to prevent corneal and conjunctival drying and ulceration in cases of massive involvement with conjunctival prolapse [179].
Corneal and Sclera
Clinical Features
Corneal and scleral involvement in leukemia is rare. There have been reports of limbal infiltration [145], corneal infiltration [182–184] and ring ulceration [182, 183]. However, these have been mostly in adults. Russo noted five pediatric patients that developed keratitis, but no further details were given [176]. These cases may have been due to infection secondary to immunosuppression, epithelial changes from chemotherapy, or dry eye secondary to graft-versus-host disease [185]. Scleral involvement is rarely noted clinically. Allen and Stratsma noted scleral involvement in 22 of 76 autopsy cases [145]. There have been no ante-mortem reports of leukemic infiltration of the cornea or sclera in children.
Diagnosis
The diagnosis of corneal or scleral infiltration can be confirmed by biopsy.
Differential Diagnosis
The corneal ulceration induced by leukemic infiltration may mimic an infectious keratitis or chronic ulceration. Episcleral involvement, if recognized clinically, may simulate episcleritis.
Treatment
In cases of corneal ulceration, infective causes have to be excluded with scrapings for microscopy and culture. In the single reported case in which resolution of corneal involvement occurred, treatment consisted of topical antibiotic and steroid in conjunction with systemic chemotherapy [183].
Anterior Segment and Glaucoma
Clinical Features
Leukemic iritis generally presents with minimal symptoms and is more common with relapse of disease. It is also more common with ALL, but may be seen in AML [186, 187]. Patients may notice photophobia, tearing, ocular pain, and headache; but these symptoms are usually mild. Pain may be a more significant symptom if secondary glaucoma develops [188]. Older children may complain of blurring of vision. Anisocoroia has been described as a feature prompting ophthalmic evaluation [189, 190].
Ciliary injection of a variable degree is commonly apparent at presentation. Leukemic involvement of the anterior segment has been mis-diagnosed as conjunctivitis [124, 190–192]. Hypopyon is commonly present [186, 187, 190, 193–202]. On occasion the leukemic infiltrate in the anterior chamber may present as a solid mass, rather than a fluid level [190]. Heterochromia iridis and hyphema are less frequently seen [124, 189, 203]. Involvement is generally unilateral; bilateral cases make up less than 20 % of those reported [187, 189–191, 194, 201, 202, 204–206].
Examination reveals a variable uveitis. Keratic precipitates, if present, are usually fine and white [124]. The iris frequently appears diffusely thickened with a smooth, velvety surface. This is secondary to infiltration of the iris stroma with resultant loss of definition of the iris stromal trabeculae and crypts [124, 207]. This infiltration is also responsible for the alteration of iris color. The iris involvement is less commonly nodular [186, 190, 204, 206, 208].
Iris infiltration may be associated with stromal hemorrhages [209]. The involved eye may appear lighter [202, 210] or darker [204, 206] than the normal eye. The hypopyon may vary from white to grey and on occasion may be admixed with hyphema [203].
Posterior synechiae have rarely been described in patients with leukemic iritis [211]. Ectropion uveae has been noted in one case of anterior segment relapse [188]. Anisocoria has been reported [189, 190], but rubeosis iridis has not been noted in the literature. Anterior segment involvement may mask posterior segment disease [195].
Elevated intraocular pressures are found in about 20 % of children with leukemic infiltration of the anterior segment [186, 187, 189–192, 194, 195, 201, 202, 204, 205, 211]. All these cases had marked involvement of the anterior chamber with hypopyon, hyphema , and/or nodular infiltration of the iris. Pain was noted in some, but not all. Secondary glaucoma is, in most cases, believed to be due to leukemic infiltration of the trabecular meshwork [124, 188]. Corneal haze is often noted in children with secondary glaucoma [188, 190]. Rarely, cases of open angle glaucoma with minimal or no anterior chamber involvement have been reported [100, 212, 213]. Acute angle closure glaucoma has also been reported in the setting of spontaneous choroidal hemorrhage in leukemic patients [214, 215].
Diagnosis
The diagnosis is generally made clinically on the basis of a combination of clinical findings described above in a patient already known to have leukemia . Iritis refractory to treatment is very common in leukemic iritis and should be high on the differential in such a patient. Also any mass or thickening of the iris should alert the clinician to such a possibility. Clinical misdiagnosis most often occurs in the rare setting of an ophthalmic presentation of leukemia [191, 204].
Most authorities recommend that the clinical diagnosis be confirmed by biopsy as accurate diagnosis is vital in planning further therapy for such patients [124, 130, 190]. This can be by needle aspiration of aqueous from the anterior chamber or by open biopsy of the iris. It is recommended that a pathologist be on hand for the immediate delivery of the specimen as the leukemic cells are extremely fragile and prompt examination is needed for accurate diagnosis .
Glaucoma should be suspected in all patients with leukemic infiltration of the anterior segment. Measurements of intraocular pressure should be part of the standard assessment of such patients.
Differential Diagnosis
Leukemic involvement of the anterior segment has been misdiagnosed as idiopathic iritis [187], endophthalmitis [191, 199] and juvenile xanthogranuloma [204]. Leukemic patients are also immunocompromised as a result of their primary disease and secondarily by their treatment. Endogenous endophthalmitis is generally seen in the setting of a very ill patient and presents with a chorioretinitis and vitritis more commonly than with an anterior uvitis [216].
There are only two reports of infectious or inflammatory anterior segment uveitis in the literature, one due to mumps [217] and the other idiopathic [190].
Incidence
Leukemic iritis is not common [124, 181, 188–197, 199, 202, 204–207, 209, 211, 219, 220]. Two large series of anterior chamber relapses have been reported [124, 194]. Bunin showed 0.5 % anterior segment relapse while Ridgway reported 1.7 % [124]. A more recent study by Somerville reports a rate of 1.43 % of relapse in the anterior chamber [153].
Natural History
The natural history of untreated leukemic iritis has not been described. Glaucoma , if not already present at the time of diagnosis, appears to supervene in a matter of days to weeks if treatment is not commenced immediately [204]. In general the raised intraocular pressure responds rapidly to treatment of the leukemic infiltrate. The leukemic infiltration appears to cause no gross permanent structural damage to the iris or angle. When the patient survives long enough to respond to treatment, the eye clinically appears to return to normal [124, 190, 194]. Lens opacities are frequently noted after ocular irradiation for anterior segment leukemia . Only one case of visually significant cataract requiring lensectomy has been reported. The patient’s vision improved markedly afterwards [201].
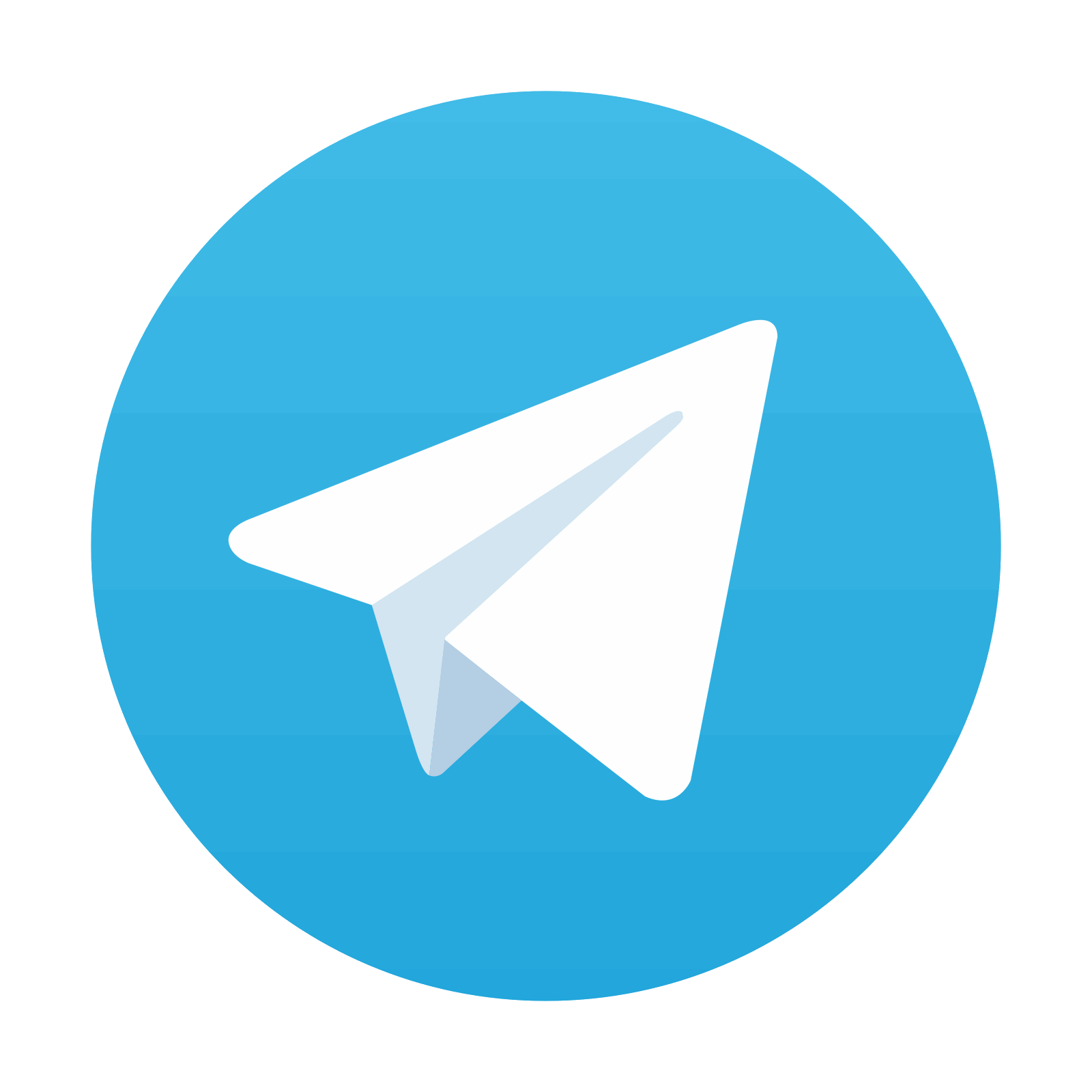
Stay updated, free articles. Join our Telegram channel

Full access? Get Clinical Tree
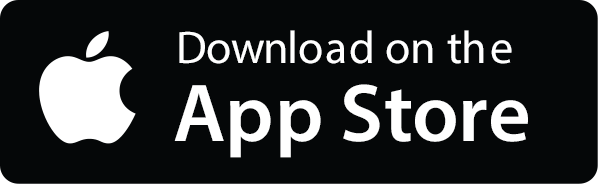
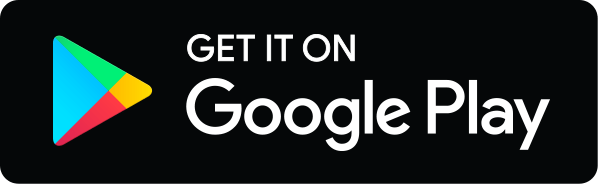
