Figure 12.1 Optical diagram of the fundus spectrophotometer. EA, confocal excitation; DA, detection apertures; S, shutters; IR, infrared.
A fundus camera with a bandpass filter from 535 to 580 nm for excitation and a bandpass filter from 615 to 715 nm as a barrier can be used to capture FAF. This camera uses a single flash to get an image of the entire retina at the same time (Fig. 12.2). The detected fluorescence signal derives from all tissue levels, in the light beam, with fluorescent properties. The filters allow long wavelengths to pass and filter short wavelengths. Filtering short wavelength reduces interference from fluorophores in the lens (which mainly emit in the range between 510 and 670 nm) especially in presence of nuclear sclerosis.
Figure 12.2 Fundus autofluorescence image with fundus camera and filters. A. Topcom camera. B. Zeiss camera.
Confocal scanning laser ophthalmoscopy, originally developed by Webb et al. (11), has a focused low-power laser beam that sweeps across the fundus in a raster pattern and then captures the reflected light through a small aperture (a confocal pinhole). The confocal pinhole suppresses light reflected or scattered from outside of the focal plane (Fig. 12.3). Light originating in the light beam, but out of the focal plane, is suppressed, reducing the autofluorescence from sources anterior to the retina (12). In contrast to the small field of the fundus spectrophotometer, cSLO allows imaging FAF over larger retinal areas and achieves high-contrast images of the posterior segment.
Figure 12.3 Confocal scanning laser ophthalmoscopy. The confocal pinhole suppresses light reflected or scattered from outside of the focal plane.
Three different cSLOs have been used for obtaining FAF images: the Heidelberg Retina Angiograph (HRA), the Rodenstock cSLO (RcSLO, Rodenstock, Weco, Düsseldorf, Germany), and the Zeiss prototype SM 30 4024 (ZcSLO, Zeiss, Oberkochen, Germany). The three cameras use an excitation wavelength of 488 nm. Emitted light is detected above 500 nm for the HRA, above 515 nm for the RcSLO, and above 521 nm for the ZcSLO (barrier filter). Bellmann et al. compared the three cameras taking in account image contrast, image brightness, and background noise. Their study showed that both image contrast and image brightness were significantly higher with the Zeiss prototype and the HRA than the RcSLO. Using a model eye to measure the background noise, they found that the highest background noise was measured with the ZcSLO and lowest with the HRA classic (13).
The Heidelberg Retinal Angiograph (the HRA classic, HRA 2, and Spectralis HRA; Heidelberg Engineering, Heidelberg, Germany HRA) is the currently commercially available cSLO equipped with excitation 488-nm solid-state laser and Heidelberg image analysis software (Fig. 12.4). The HRA can register and average multiple FAF images (typically from 9 to 15 single images) in order to amplify the FAF signal. With the signal amplification, the background noise is reduced and the image contrast is enhanced.
Figure 12.4 Fundus autofluorescence image with cSLO.
The differing wavelengths used by cSLO and fundus camera systems suggest that each system may record fluorescence from a different complement of fluorophores. To compare two FAF images from different cameras, it is useful to identify pathognomonic fundus changes undetectable by only either FAF equipment (14). An example of this situation is the difference in the hypoautofluorescence of macula with no pathology; normal macula with cSLO appears darker than with the fundus camera.
FUNDUS AUTOFLUORESCENCE IN RETINAL DISEASES
The FAF image shows the spatial distribution of the intensity of the signal for each pixel in gray values. These are arbitrary values from 0 to 255. Low pixel values (dark or hypoautofluorescence) shows low intensities. High pixel values (bright or hyperautofluorescence) shows high intensities.
Normal Fundus
The topographical distribution of an FAF in normal retina shows a consistent pattern (12). Retinal vessels are associated with a reduced FAF signal because of the absorption of the signal by blood. The optic nerve head appears hypoautofluorescent due to the absence of the RPE (no lipofuscin). The posterior pole shows a diffused autofluorescence signal. In the macula area, the FAF signal shows high degree of interindividual variability; in general, the signal is reduced at the fovea due to absorption of the signal by the luteal pigment. The signal in the parafoveal area is higher than in the fovea but still exhibits a relatively decreased intensity compared with the background signal. It has been speculated that this observation is caused by increased melanin deposition and lower density of lipofuscin granules in central RPE cells (5).
Abnormal autofluorescence signals can be derived either from a change in the amount or composition of lipofuscin in the RPE cell or from the presence of absorbing material (blood, lens opacity) anterior to the RPE. The identification of abnormalities in the FAF image is dependent on the quality of the recorded image (Fig. 12.5). Vitreous opacities, cataract or lens changes, and cornea abnormalities may affect the autofluorescence signal at the level of the RPE (Table 12.1).
Figure 12.5 FAF in a patient with cataract.
Table 12.1 CATEGORIES FOR INCREASED OR REDUCED FAF SIGNALS
aDiabetic macular edema can have either increased or decreased autofluorescence.
Data from Schmitz-Valckenberg S, Holz FG, Bird AC, et al. Fundus autofluorescence imaging: review and perspectives. Retina. 2008;28(3):385–409.
There are qualitative descriptions of localized FAF changes that are widely used. The FAF signal over a certain retinal location is categorized as decreased (hypoautofluorescence), normal, or increased intensities (hyperautofluorescence) in comparison to the background signal of the same image (Fig. 12.6).
FIGURE 12.6 FAF showing areas of hyperautofluorescence (yellow arrow) and areas of hypoautofluorescence (white arrow).
Fundus Autofluorescence Image for Dry Age-Related Macular Degeneration
Early Manifestation of Age-Related Macular Degeneration
Early manifestations of AMD include focal hypopigmentation and hyperpigmentation at the RPE level and drusen with extracellular material accumulating in the inner aspects of the Bruch’s membrane. Postmortem analyses demonstrate that some molecular species in drusen material possess autofluorescent properties.
The FAF image for early changes in AMD has been described by several investigators using both cSLO device and fundus camera–based systems. Drusen visible by fundus photography are not necessarily correlated with notable FAF changes; areas of hyperautofluorescence may or may not correspond with areas of hyperpigmentation or drusen (Fig. 12.7). In general, larger drusen are associated with more pronounced FAF abnormalities than smaller ones (15); there are two exceptions for this observation: the basal laminar drusen, which have a prominent appearance, and the crystalline drusen, which have a decreased FAF signal.
FIGURE 12.7 A. Color fundus photo showing drusen. B. FAF of the same eye showing areas of hypo- and hyperautofluorescence that do not correspond with drusen at the color fundus photo.
FAF (especially with fundus camera–based system) may show a pattern of ring distribution associated with drusen. This pattern consists of hypoautofluorescence in the center of the drusen surrounded by an annulus of hyperautofluorescence (16). It has been speculated that this appearance is caused by a peripheral displacement of the overlying RPE cytoplasm and/or lipofuscin granules. Confluent drusen and large foveal soft drusen topographically correspond with mildly increased FAF. Coalescence of several small, hard, or soft drusen shows irregular areas of FAF. Focal areas of hyperpigmentation (areas with increased melanolipofuscin) on fundus photographs may show as increased FAF intensity (Fig. 12.8). Areas of hypopigmentation (areas with absence of RPE cells) on fundus photographs tend to be associated with a corresponding decreased FAF signal (Fig. 12.8). Reticular drusen are identified as a peculiar pattern on FAF images (especially with cSLO). Reticular drusen present with multiple, relatively uniform, roundish, or elongated spots with decreased intensities that are surrounded by an interlacing network of normal-appearing FAF signals.
FIGURE 12.8 A. Color fundus photo shows hyperpigmented area secondary to RPE clumping and hypopigmented area secondary to RPE loss. B. FAF of the same eye shows hyperautofluorescence area corresponding with RPE clumping and hypoautofluorescence area corresponding with RPE loss.
To describe various abnormal FAF patterns in early AMD and to develop an FAF classification system, a workshop was organized by the Fundus Autofluorescence in Age-Related Macular Degeneration Group (FAM study group) in Germany on July 2003. The International FAM study group (17) has identified eight patterns: minimal change, focal increase, lacelike, reticular, speckled, patchy, linear, and plaque-like patterns (Fig. 12.9):
Figure 12.9 The International FAM study group has identified eight patterns: minimal change, focal increase, lace-like, reticular, speckled, patchy, linear, and plaque-like patterns. A,B. Normal. C,D.Minimal change: minimal variations from normal background FAF. E,F. Focal: several well-defined spots with markedly increased FAF. Fundus photograph of the same eye with multiple hard and soft drusen. G,H. Patchy: multiple large areas of increased FAF corresponding to large or soft drusen and/or hyperpigmentation on the fundus photograph. I,J. Linear: characterized by the presence of at least one linear area with markedly increased FAF. A corresponding hyperpigmented line is visible in the fundus photograph. K,L. Lace-like: multiple branching linear structures of increased FAF. This pattern may correspond to hyperpigmentation on the fundus photograph or to no visible abnormalities. M,N. Reticular: multiple, specific small areas of decreased FAF with brighter lines in between. The reticular pattern not only occurs in the macular area but also is found more typically in a superotemporal location. There may be visible reticular drusen in the corresponding fundus photograph. O,P. Speckled: variety of FAF abnormalities in a larger area of the FAF image. There seem to be fewer pathologic areas in the corresponding fundus. (Reprinted from Bindewald A, Bird AC, Dandekar SS, et al. Classification of fundus autofluorescence patterns in early age-related macular disease. Invest Ophthalmol Vis Sci. 2005;46(9):3309–3314, with permission.)
Minimal change: Eyes with minimal variations form the normal homogenous background; FAF without an obvious topographic pattern.
Focal increased pattern: Presence of at least one spot (less than 200 μm diameter) of markedly increased FAF much brighter than the surrounding backgrounds. The borders are well defined, with no gradual decrease of FAF observed between the background and the area with focal increased FAF. Some areas of focal increased FAF may be surrounded by a darker-appearing halo. These areas may or may not correspond to visible alterations (like drusen or focal hyperpigmentation).
Patchy pattern: Presence of at least one larger area (greater than 200 μm diameter) of markedly increased FAF. These areas are brighter than the surrounding background fluorescence. The borders of the areas are typically less well defined than the previous patterns. These areas may or may not correspond to visible alterations.
Linear pattern: Defined by the presence of at least one linear area of markedly increased FAF. The borders of the areas are typically well demarcated with no gradual decrease in FAF observed between the background and the linear structure. These linear structures of increased FAF correspond to hyperpigmented lines on color fundus photographs.
Lacelike pattern: Shows multiple-branching linear structures of increased FAF (lacelike pattern). This pattern may or may not correspond to hyperpigmentation on color fundus photos.
Reticular pattern: Multiple small areas of decreased FAF (less than 200 μm diameter). This pattern is found in the macula and in a superotemporal location. It may be associated with numerous small drusen or areas with pigmentary changes.
Speckled pattern: Characterized by simultaneous presence of a variety of FAF abnormalities in a large area and may cover the entire posterior fundus. The corresponding abnormalities on color fundus photographs include hyper- or hypopigmentation and multiple confluent drusen.
The FAM study group demonstrated with these observations that it is a poor correlation between the visible alterations on fundus photographs and the changes in the FAF. The findings of the FAF may indicate more widespread damage and may precede the visible lesions. This group also identified a few patients with very early dry AMD and a normal homogenous background at the FAF; the absence of abnormal alterations at the FAF, even in the presence of drusen at the fovea, was explained by the masking effects of the luteal pigment.
The progression in the fundus appearance, marked by changes in the drusen size, does not necessarily correlate with intensity changes in the FAF and vice versa (15). A longitudinal analysis within the FAM study included 125 eyes with soft drusen and no history of laser treatment (18). During the follow-up (mean 18 months), severe visual loss occurred in 11 patients, whereas two developed GA and nine exudative advanced AMD. The two GA eyes were classified as having the focal FAF pattern and the focal plaque-like pattern at baseline. The GA in these eyes developed following the collapse and flattening of large drusenoid RPE detachment. The patchy FAF pattern was found to be the most frequent in the eyes with progression to exudative AMD. A total of 35 eyes were classified to exhibit patchy pattern at baseline, and six of them developed exudative AMD over the review period. This finding strongly suggests that the patchy FAF pattern in early AMD may represent indeed a high-risk marker for progression to advanced AMD.
Geographic Atrophy
GA represents the late manifestation of dry AMD. It is characterized by the development of atrophic patches that initially occur in the parafoveal area. During the natural course of the disease, atrophy slowly enlarges over time, and the fovea itself is typically not involved until later. Histopathologic studies have shown that clinically visible areas of atrophy are confined to areas with absence of photoreceptor cells, RPE atrophy, and atrophy at the collateral tissue layers. With the disappearance of RPE, lipofuscin is also absent resulting in a corresponding marked decrease in FAF intensity over atrophic areas. The areas surrounding the atrophy (junctional zone) show high-intensity FAF levels. This junctional zone may appear to be slightly darker than the adjacent RPE by biomicroscopy and may appear to subtly block the background choroidal fluorescence during fluorescein angiography.
The photoreceptors and the RPE function in the junctional zone are impaired. The hyperautofluorescence of this area is indicative of exceptional levels of lipofuscin. The accumulation of lipofuscin occurs before cell loss and predisposes to atrophy expansion. Photoreceptor function is dependent on normal RPE function. Several compounds in the lipofuscin are toxic for the RPE and interfere with its normal work. An impaired RPE, loaded with lipofuscin, is not able to phagocytize the photoreceptor’s outer segments leading to photoreceptor damage. Furthermore, there is some evidence that the ongoing photoreceptor cell dysfunction and degeneration could also be responsible for the hyperautofluorescence in the junctional zone (19).
Natural history of advance atrophic AMD demonstrated a high variability in the progression of the atrophy. Using color fundus photos, the mean progression rate is 2.6 mm2/year; with FAF, the mean progression rate is 1.74 mm2/year (20,21). The variability in the progression rate between patients cannot be explained by the baseline atrophy or any demographic factor (e.g., smoking, lens status, family history) (22,23). Furthermore, there is a linear growth of atrophy over time, and the best predictor of future atrophy would be the growth rate in the previous year. The progression of GA is best studied in the junctional zone at the margin of intact and atrophic RPE, where increased FAF signal may be noted in varying levels. The diversity patterns of abnormal FAF in the junctional zone have a high degree of intraindividual symmetry between the eyes (24). FAF has shown that the extension of areas of hyperautofluorescence surrounding atrophy patches correlates with atrophy progression over time.
The FAM study introduced a classification of the FAF pattern for eyes with GA (25). This classification consists of five main patterns (none, focal, banded, diffuse, and patchy), and the “diffuse” pattern is further subdivided into five subtypes (Table 12.2) (21). A recent analysis of atrophy progression rates over time and FAF pattern at baseline revealed that variation in GA growth rates is dependent on the specific phenotype of abnormal FAF pattern at baseline (21). The progression rates in eyes with the “banded” (median 1.81 mm2/year) and the “diffuse” FAF patterns (1.77 mm2/year) were significantly higher compared with eyes without FAF abnormalities (0.38 mm2/year) and “focal” FAF patterns (0.81 mm2/year). Within the group of the “diffuse” pattern, eyes with a “diffuse trickling” pattern were identified that exhibited an even higher spread rate (median 3.02 mm2/year) compared to the other diffuse types (1.67 mm2/year) (Fig. 12.10).
Table 12.2 ABNORMAL PATTERN OF FAF IN THE JUNCTIONAL ZONE OF GA
Data from Holz FG, Bindewald-Wittich A, Fleckenstein M, et al. Progression of geographic atrophy and impact of fundus autofluorescence patterns in age-related macular degeneration. Am J Ophthalmol. 2007;143(3):463–472.
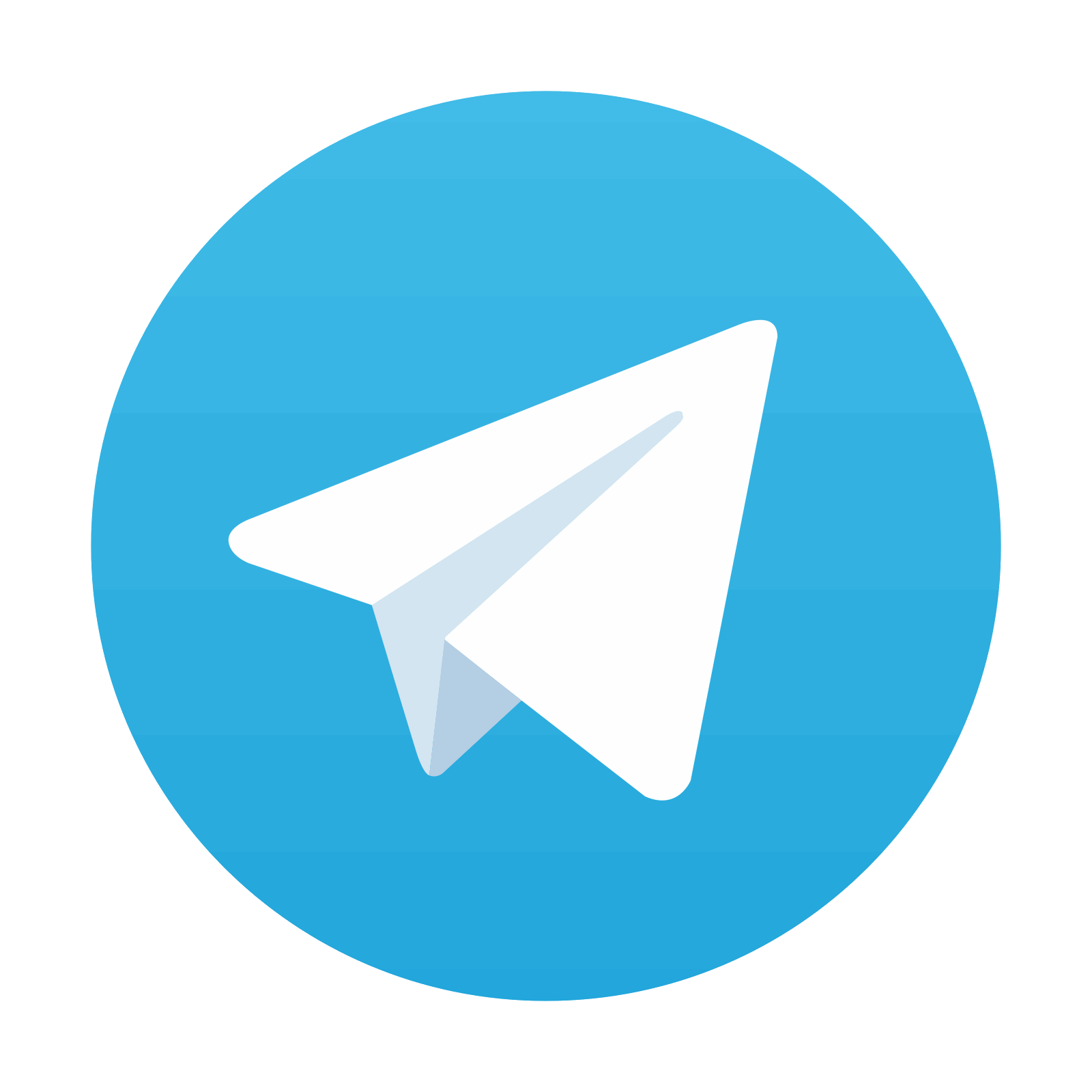
Stay updated, free articles. Join our Telegram channel

Full access? Get Clinical Tree
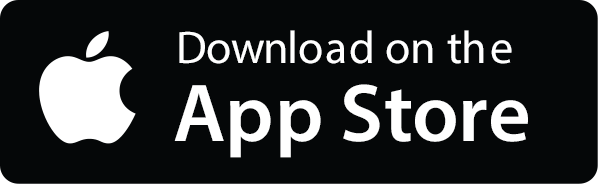
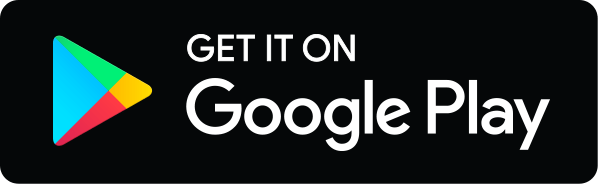