Fig. 21.1
(a–e) Swelling of inferior turbinate according to the nasal cycle in a healthy young man (parallel endoscopy using a 0° endoscope): (a) Decongested mucosa on the right and congested mucosa on the left side at the beginning (b) Change of swelling (c) Maximal congestion on the right and maximal decongested mucosa on the left side (d) Reciprocal change of swelling (e) Same situation at the end of the nasal cycle as at the beginning
With the endoscopic description in memomotion, the extent of the congesting process could be evaluated precisely. The processes of congestion and decongestion occur relatively rapidly so that the turbinates remain most of the time in a kind of plateau phase of submaximal to maximal congestion or decongestion. The size of the turbinates varies between very small and very large with complete obstruction of the nasal cavity in the visible area. During the course of the cycle, complete decongestion of both turbinates never occurred. For a short phase, a similar middle congestion status of both turbinates can be found.
The diagnosis of hyperplastic turbinates is often made in the clinical routine, but with the background of the mentioned results, it must be reconsidered. Hyperplasia means first the enlargement of tissue by cellular multiplication. During the nasal cycle, the healthy turbinate continuously changes from a low to a high degree of congestion. Internal and external factors additionally influence the extent of the swelling situation. As the physiology of a normal turbinate allows each size of minimal to maximal, there is no reference for the diagnosis of hyperplasia or hypertrophy or for definition of a standard normal size. Even if one side of the nose is completely obstructed because of the maximally congested inferior turbinate, the other free side allows a sufficient nasal air passage because it is decongested according to the physiologic nasal cycle. Only the bilateral massive swelling of the inferior turbinates seems to be unphysiologic (Keerl et al. 1995; Weber and Keerl 1996). It seems to be appropriate to use in the daily language exclusively the term of congested or enlarged turbinate and to use the term of hyperplasia only for enlarged turbinates with neoplastic changes of the surface (polypous, mulberry-like changes).
Besides the congesting and decongesting processes, regular changes of the secretory production could be observed endoscopically (Weber and Keerl 1996) as they had already been described by Melon (1968). The congesting turbinate seemed to become increasingly humid so that the maximally congested situation led to small droplets. This may be considered as the cause of the parasympathic tonus prevailing on this side. During the decongesting phase, the mucosa dries more and more, partly with resulting small dry layers. In this context, the dehydrating effect of nasal breathing accompanies the sympathomimetic and reduced parasympathic tonus.
21.2 Types of Nasal Cycles
Three types of nasal cycles are described (Grützenmacher et al. 2005): the classical reciprocal type, the in-concert type (simultaneous reduction and increasing of the nasal passage on both sides), and the irregular type.
In addition, Kern describes three types of non-cycle nose: there is no fluctuation of the nasal passage, the fluctuation is moderate only on one side, and the fluctuation does not change from one side to the other (Kern 1981).
The cyclic duration is very variable with an interval of about 1–6 h (Eccles 1982; Hasegawa and Kern 1977; Heetderks 1927; Keuning 1968; Keerl et al. 1995; Weber and Keerl 1996; Eccles 1978).
More recent quantitative studies using numerical parameters seem to show that true periodicity and reciprocity of nasal airflow exist only in 21–39 % of the population, reciprocity being the truly reciprocal changes in air flow between right and left passages and periodicity being the regularity of changes in airflow occurring with time in each nasal passage. Otherwise, these studies used measurement periods no longer than 8 h. So the time period of examination may be too short to discover the nasal cycle as was outlined by Grützenmacher.
Also in children, a nasal cycle can be revealed (Gallego et al. 2006; van Cauwenberge and Deleye 1984; van Cauwenberge 1980; van Cauwenberge et al. 1984). For children up to the age of 6, it amounts to a mean of 1 h and 20 min and is not influenced by physical activity. Even infections do not significantly influence the nasal cycle.
Even if the distribution of the nasal cycle types changes, a fluctuation of the nasal passage is found in laryngectomised patients (25 % classical type, 40 % irregular type, 35 % in-concert type) (Fisher et al. 1994).
According to Ingels et al. (1990), no correlation is found between the ciliary beating rate (CBR) of the nasal epithelia and the rhinomanometrically measured passage of the nasal cavity. The CBR of different cells in the same biopsies was clearly different.
In contrast to this, Doyle and van Cauwenberge (1987) could detect a higher mucociliary clearance rate with increased nasal passage by means of the saccharin test. Also Soane et al. (2001) found a significant acceleration of the mucociliary clearance on the free side in comparison to the obstructed nasal side with a factor of 2.5:1 using the radioisotope method.
Pearls
Spontaneous changes in nasal airway resistance due to congestion and decongestion of nasal venous sinuses are called nasal cycle.
Three types of nasal cycles are described with a duration of about 1–6 h.
The regulation of the nasal cycle is probably performed by the central sympathomimetic tonus.
Consider the nasal cycle and other physiologic changes in the congestion of the nasal mucosa when making a clinical assessment of a patient complaining of nasal obstruction.
Long-term rhinoflowmetry offers a new possibility for investigating nasal patency for up to 72 h.
21.3 Origin, Function, and Regulation of the Nasal Cycle
Finally, the origin and the function of the nasal cycle are unknown (Eccles 1982; Cole 1982; Maran and Lund 1990). According to Mlynski et al. (2000), the decongested side is in the working phase of the nose (Grützenmacher et al. 2005). The respirated air passes through the decongested nasal side; the air is conditioned, i.e. warmed up, moistured, and cleaned. The congested side is in its resting phase.
The regulation of the nasal cycle is probably performed by the central sympathomimetic tonus (Eccles 1978, 1982, 2000; Hanif et al. 2000). This tonus is controlled by two centres in the brain stem. They are connected with each other and dominate the tonus via the right and left cervical sympathomimetic nerval fibres finally asymmetrically and alternating over a period of several hours.
Changes of the nasal patency that are related to positional changes may be explained by two different mechanisms: an increased central venous pressure when changing from a standing to a lying position and a reflectory change of the nasal vasomotoric tonus when a lateral lying position is taken. The increase of the venous pressure causes an increased filling of the nasal venous sinusoids and an increase of the nasal resistance. The passive hydrostatic effect adds to each asymmetry in the context of the nasal cycle. The side with the highest degree of congestion generally has the highest increase of swelling and is completely obstructed (Cole and Haight 1986). Lying down leads to a temporary disturbance of the nasal cycle with congestion of the mucosa and increased flow resistance. The amplitudes of the changes of the resistance in the nasal cycle are higher in supine and lateral position (Cole et al. 1979; Cole and Haight 1986, 1984; Haight and Cole 1984).
The reciprocal changes of the nasal patency when taking a lateral lying position are induced by the pressure in the area of the shoulder girdle, the lateral thorax, and the hip that are the most sensitive regions. During local anaesthesia in the area of the skin, surface of the axilla cannot suppress this reflectory reaction; this is possible by an intercostal neural blockade (Haight and Cole 1986). This leads to the effect that the upper side of the nose is mainly open for nasal passage. An explanation may be that this avoids closure of the inferior side of the nose when lying on the ground so that nasal breathing is still possible.
The diagnosis of nasal obstruction and the nasal patency has to bear in mind the physiologic nasal cycle as well as changes of the nasal respiratory resistance in dependence of the surrounding conditions (temperature, probably humidity, irritating substances) (Battle1989; Schlegel and Gammert 1991), the physical activity, body position (Rao and Potdar 1970; Hasegawa and Saito 1979; Rundcrantz 1969; Jackson 1970), or pharmacological influences (Baumann and Masing 1970).
Physical activity leads to a reduced nasal resistance (Eccles 2000; Cole et al. 1983a; Dallimore and Eccles 1977; Hasegawa and Kern 1978; Richerson and Seebohm 1968). According to some studies, the nasal resistance mostly increases with cold temperatures (Battle 1989; Salman et al. 1971; Takagi et al. 1969). Schlegel reports about a main decrease of the resistance with cold temperatures and an increase with warmth. The change of the humidity of 20 % to more than 90 % has no influence on the nasal patency (Salman et al. 1971).
The nasal cycle may influence the nasal allergy provocation test (Pirilä et al. 1997; Sipila et al. 1990; Gilbert and Rosenwasser 1987; Gungor et al. 1999). Gotlib et al. (2005) found that the determination of the bilateral reduction of the cross-section surface in the area of the inferior turbinates is more sensitive than the determination of the one of the more reactive side. However, the risk of a spontaneous unilateral total increase of the nasal resistance must be considered.
There is some evidence to suggest that the nasal cycle is associated with ultradian rhythms of the cerebral hemispheres which affects cognitive function of the brain (Shannahoff-Khalsa et al. 1991; Block et al. 1989). Alternating dominance of cerebral hemispheric activity can be demonstrated in humans by EEG, and relative changes in electro-cortical activity seem to have a direct correlation with the nasal cycle (Werntz et al. 1983). These studies found a greater EEG activity on the side contralateral to the decongested side of the nose; a significant improvement in spatial and verbal cognitive function performed by the contralateral (in females) and ipsilateral (in males) cerebral hemisphere occurred in unilateral forced nasal breathing (Shannahoff-Khalsa et al. 1991). This may explain why some patients with nasal obstruction find this more than just a simple annoyance. The nasal obstruction may have effects on the ability to work during daytime (Hanif et al. 2000).
Patients with upper respiratory tract infections become far more apparent with a significant increase in the amplitude of the changes than healthy people (Eccles et al. 1996).
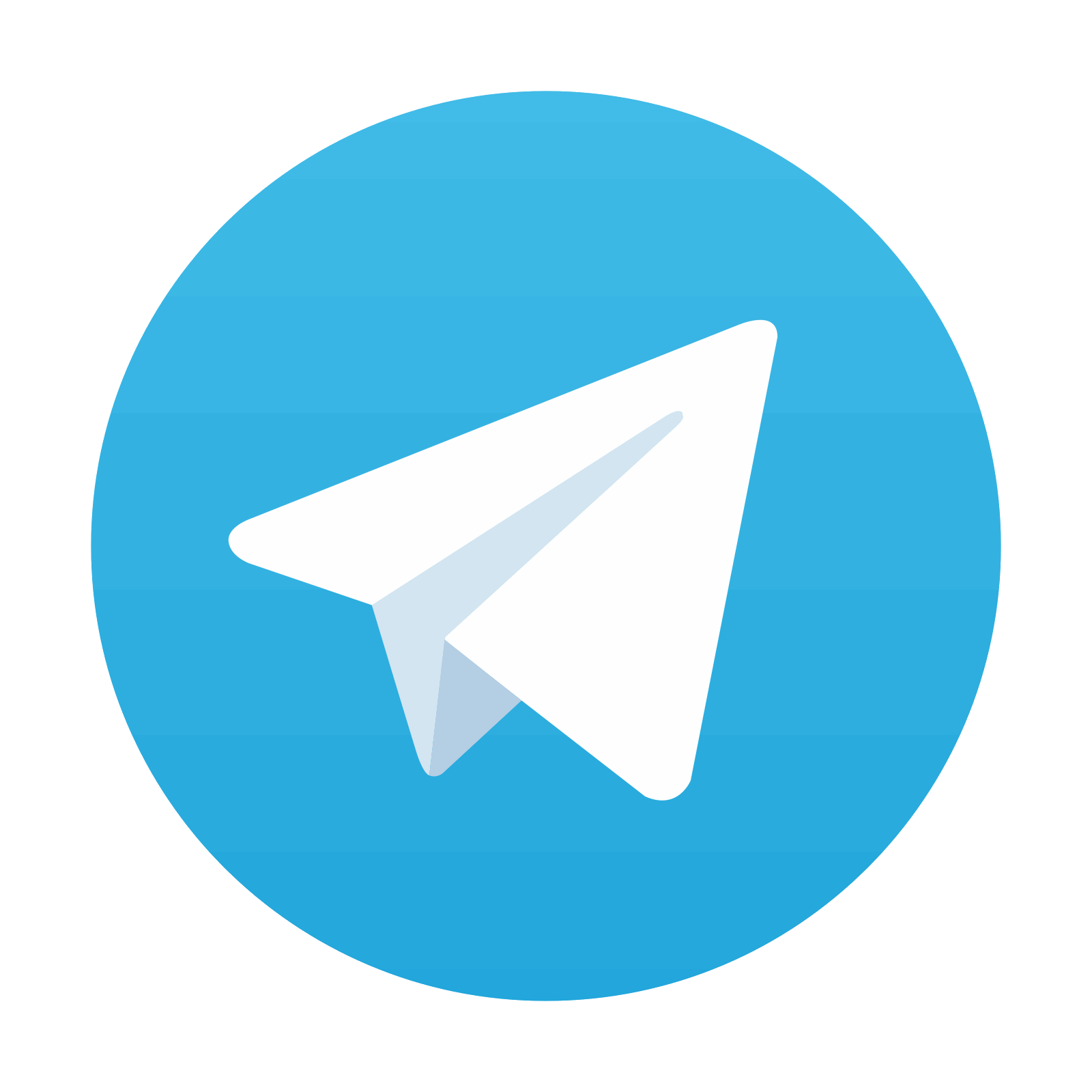
Stay updated, free articles. Join our Telegram channel

Full access? Get Clinical Tree
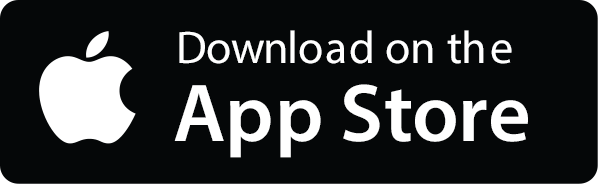
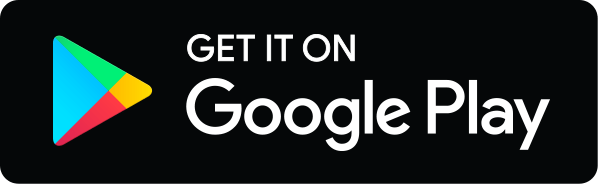