It was with great interest that we read the paper by Alsulaiman and associates. Recently, we published a case of bilateral macular hole from a handheld laser pointer in a young patient, and we would like to further the discussion on specific aspects of macular hole formation from exposure to blue laser beams.
In our case the device was a nominally 474 nm laser, which in fact refers to a 473 nm frequency doubling of 946 nm laser from a diode-pumped neodymium-doped yttrium-aluminum garnet (Nd:YAG) or neodymium-doped yttrium orthovanadate crystal, using barium borate as nonlinear frequency doubler. In the cases presented by Alsulaiman and associates, it seems to be an indium gallium nitride semiconductor laser, capable to produce blue light without frequency doubling at 445 nm (447 nm ± 5 nm).
Regarding the mechanism for the retinal damage, both photodisruption and photocoagulation have been proposed. Photodisruption mandates the formation of plasma, achieved by focusing energy in a small spot, typically <50 pm in diameter. Short-pulsed Nd:YAG lasers can produce enough irradiance, usually 10 10 –10 11 W/cm 2 (for the visible spectrum, owing to the focusing of the crystalline lens, the equivalent at the cornea level is 10 5 –10 6 W/cm 2 ), to induce optical breakdown, dissociating electrons from their atoms and creating a plasma. Nd:YAG lasers achieve this either by Q-switching or by mode-locking techniques. The laser pointers under investigation are neither of these, and most likely are continuous wave (CW) laser sources.
Therefore, we should rather focus on photocoagulation effects. It is important to mention that the peak absorbance of blue light waves occurs at the level of high concentration of xanthophylls corresponding to certain topographic locations in the retina—that is, retinal nerve fiber layer (RNFL), inner plexiform layer (IPL), and outer plexiform layer (OPL)—hence these are the most probable target tissues. Possibly, the initiation of the damage occurs at these anatomic areas and absorption of large amounts of energy may lead to dehiscence of these layers from neighboring layers, allowing for a cascade of events (hydration theory may apply here) that leads to macular hole formation.
The authors mention a hyperreflectivity of the retinal pigment epithelium (RPE) in optical coherence tomography. This could be attributed to the absence of the overlying tissue, and could be regarded as an artifact. Regarding the hyperreflective excrescences along the surface of the RPE in the hole bed, the authors believe them to be either nodular proliferations of the RPE or eosinophilic material–laden or lipofuscin-laden macrophages. We propose that these could represent clumps of remnants of the photoreceptor ellipsoid zone. They appear to be hyperreflective compared to the ellipsoid, possibly because of the absence of the overlying tissue. In our case a strand of ellipsoid tissue running vertical alongside the margin of the hole as well as visible remnants of the ellipsoid lining the RPE are evident. We postulate that the young age combined with the abrupt formation of the hole may explain this phenomenon.
Finally, the uncontrolled availability of such devices may feed youngsters’ curiosity and experimentation, and we would like to urge public education regarding the danger posed by such devices. Moreover, it is quite possible that minor or outside-the-avascular-zone defects never reach the doctor’s office, and the cases presented may represent only the tip of the iceberg.
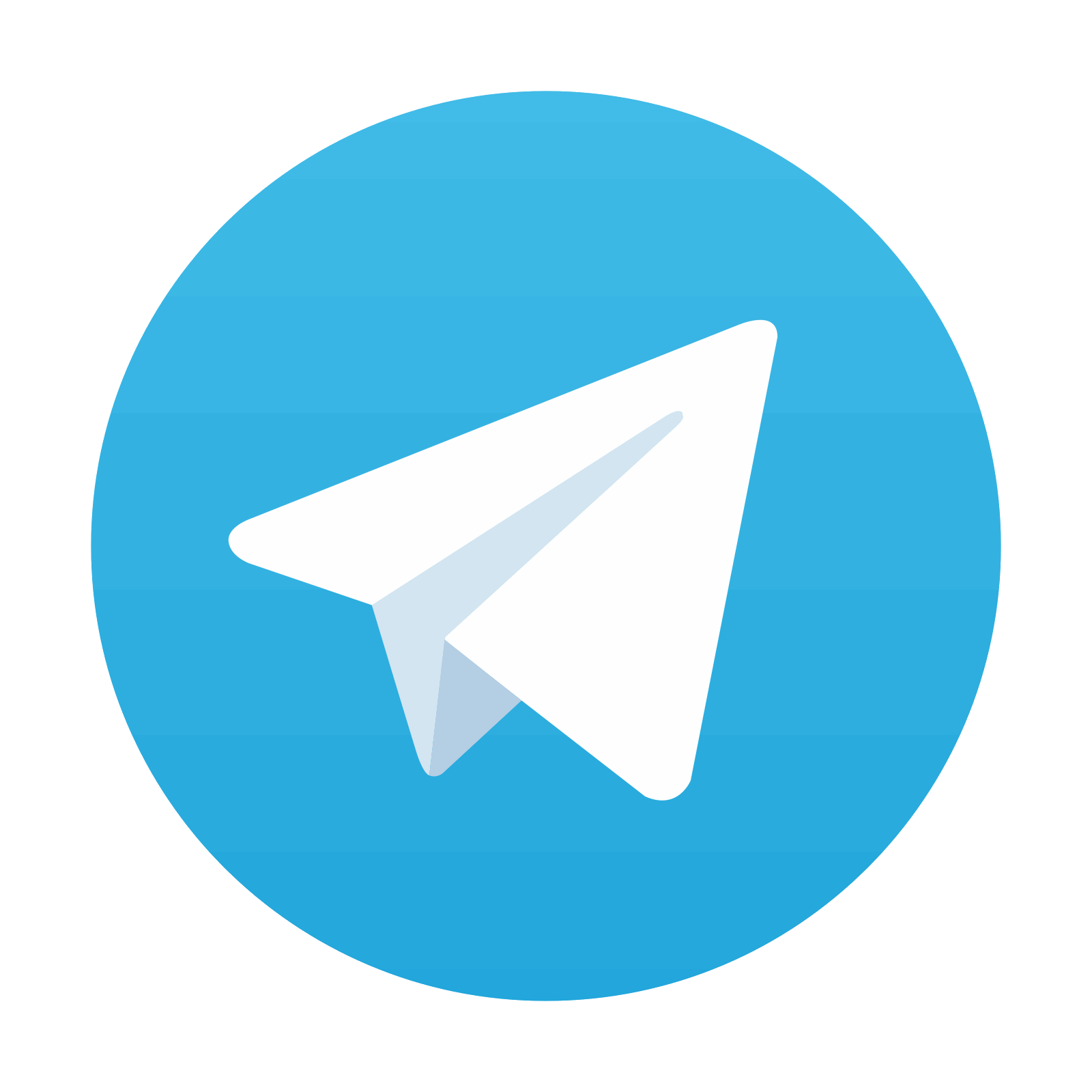
Stay updated, free articles. Join our Telegram channel

Full access? Get Clinical Tree
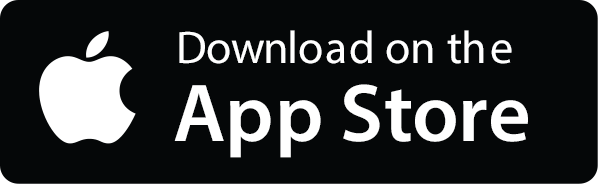
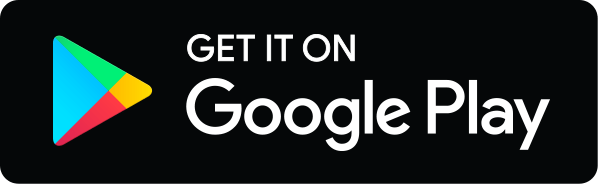