Table 31.2
Components of the Facial Nerve
Type | Component | Function |
Efferent | Branchial motor | To supply the stapedius, stylohyoid, posterior digastric, and muscles of facial expression, including the buccinators, platysma, and occipitalis muscles. |
Visceral motor (general visceral efferent) | Preganglionic parasympathetic fibers passing through the greater superficial petrosal nerve. Stimulates the lacrimal, submandibular, and sublingual glands. Also glands within the mucous membrane of the nose and hard and soft palates. | |
Afferent | General sensory (general somatic afferent) | Sensory fibers from the skin of portions of the auricle and external auditory canal. These fibers pass through the nervus intermedius. |
Special sensory | Taste from the anterior two-thirds of the tongue. |
The facial nerve motor nucleus is located in the caudal aspect of the ventrolateral pons. Efferent motor fibers take a circuitous path anteromedially to loop around the abducens nucleus and then enter the internal auditory canal with the cochlear and superior and inferior vestibular nerves. The mnemonic “7 UP over COKE” reminds one that in the distal internal auditory canal, the CN VII is superior to the cochlear nerve. Motor fibers then take a sharp turn posterolaterally as they pass through the geniculate ganglion (ganglion for taste and sensory fibers) and enter the middle ear space. In the middle ear, the motor nerve passes just superior to the oval window and stapes footplate. The motor nerve then makes its “second genu” as it leaves the middle ear to enter the mastoid bone. As the motor branch exits the stylomastoid foramen to enter the body of the parotid gland, fibers innervate the auricular, stylohyoid, posterior digastric, and occipitalis muscles. In the face, the nerve arborizes extensively. Classic descriptions depict the nerve dividing into an upper and lower division at the pes anserinus (Latin for “goose foot”), and then into five major branches at the anterior edge of the parotid gland: temporal, zygomatic, buccal, marginal, and cervical. Table 31.3 presents some surgical pearls and landmarks for these main branches. More recent cadaver dissections demonstrate significant variability in branching pattern with eight to 14 large branches in most individuals.5 The facial nerve lies deep to the superficial muscular aponeurotic system (SMAS) and all of the muscles of facial expression except for the mentalis, buccinator, and levator anguli oris.6
Table 31.3
Branches of the Facial Nerve
Branch | Surgical Pearls/Landmarks |
Frontal (Temporal) | Innervates the frontalis muscle. Typically 2–4 branches cross the zygomatic arch between roughly 2 cm anterior to the root of the helix and 2 cm posterior to the junction of the arch and the body of the zygoma. The nerve is quite deep over the zygomatic arch, beneath the parotidomasseteric fascia. The nerve enters the deep aspect of the temporoparietal fascia starting 15 mm superior to the arch and 15 posterior to the lateral orbital rim. |
Zygomatic | Innervates the orbicularis oculi. Relatively well-protected under the orbicularis oculi and zygomatic major muscles. |
Buccal | Innervates the zygomaticus major, making it the main driver of smile. Innervates orbicularis oris. There are typically several large buccal branches, often with contributions from the upper and lower divisions of the facial nerve. Quite exposed between the anterior edge of the parotid gland and the zygomatic major muscle. Branches can be seen directly on the surface of the masseteric muscle, deep to the parotidomasseteric fascia. Some research suggests that terminal branches innervate the “medial canthal” segment of the orbicularis muscle, which is most important for lower eyelid tone and blink. Terminal branches pass inferior to the orbit to innervate the glabellar musculature (corrugator, procerus). |
Marginal mandibular | Innervates the lower lip depressors. Easiest branch to injure due to its long course. Usually passes within 1 cm of the angle of the mandible but has been found several centimeters inferior to the mandible lateral to the facial artery. Always above the inferior border of the mandible anterior to where the facial artery crosses the mandible. |
Cervical | Innervates the platysma. Relatively unimportant. Injury can cause smile asymmetry as a result of loss of lip depressor function of the platysma. |
Efferent parasympathetic fibers originate in the superior salivary nucleus in the pontine tegmentum. These fibers traverse the cerebellopontine angle completely separate from the motor root, within the nervus intermedius (which also contains afferent taste fibers), before joining the motor fibers at the geniculate ganglion. Some efferent parasympathetic fibers exit almost immediately as the greater superficial petrosal nerve (GSPN). The GSPN is joined by sympathetic fibers jumping off the internal carotid artery as the deep petrosal nerve to form the vidian nerve. The vidian nerve enters the pterygopalatine ganglion where preganglionic parasympathetic nerves destined for the lacrimal gland synapse. Postganglionic parasympathetic fibers then leave the pterygopalatine fossa, joining the infraorbital and then lacrimal nerves to reach the lacrimal gland. Efferent parasympathetic fibers destined for the submandibular and sublingual salivary glands pass through the geniculate ganglion and leave the main motor nerve in the mastoid segment as the chorda tympani nerve. These fibers synapse in the submandibular ganglion.7
Afferent taste fibers from the anterior two-thirds of the tongue travel with the lingual nerve before joining the chorda tympani nerve, with cell bodies in the geniculate ganglion and a final target within the nucleus solitarius. Touch sensory fibers from the auricle and ear canal are the second afferent pathway. These fibers also have cell bodies in the geniculate ganglion and pass through the nervus intermedius before terminating in the spinal tract of the trigeminal nerve.4 This is one of many ways that the facial and trigeminal nerves are closely aligned.
Embryology
During the third week of gestation, the facioacoustic primordium appears and differentiates into CNs VII and VIII. By the fifth week of gestation, the nervus intermedius, geniculate ganglion, chorda tympani, and greater superficial petrosal nerves are visible. The branchial arches are each associated with a CN, and the facial nerve is the nerve associated with the second branchial arch derivatives, which include all of the targets described above, as well as the stapes, styloid process, stylohyoid ligament, and portions of the hyoid bone. By the sixth week of gestation, the first and second branchial arches give rise to small condensations of mesoderm termed the hillocks of His. These fuse to form the auricle around the 12th week of gestation. Malformations of the pinna suggest a developmental anomaly within the second branchial arch and should alert the physician to possible abnormalities in the structure and/or function of the facial nerve.8
The parotid gland begins as an outpouching of oral ectoderm during the sixth week of gestation. The facial nerve grows anteriorly as the parotid gland grows posteriorly, eventually encapsulating much of the facial nerve. The parotid gland is the last major salivary gland to encapsulate, which is why it contains lymph nodes. The facial nerve divides the parotid gland into “superficial” and “deep” lobe; however, these two regions are not distinct entities.9 There may be lymph nodes in both the superficial and deep lobes. This fact must be kept in mind when considering regional lymphadenectomy for advanced cutaneous malignancies.10
Epidemiology
It is difficult to estimate the relative frequency of facial palsy etiologies. Most data are kept at large academic facial nerve centers that probably do not represent a general population of patients. The most common etiologies of facial paresis among surgical patients at the University of Pittsburgh Facial Nerve Center between 1963 and 1996 were acoustic neuroma surgery (25%), Bell palsy (17%), parotid and skin neoplasms (8%), varicella zoster (6%), trauma (6%), and genetic or congenital causes (6%).11 The Massachusetts Eye and Ear Infirmary Facial Nerve Center recently reported a series of 2000 patients presenting with facial palsy, not necessarily requiring procedural intervention. The most common etiologies were Bell palsy (38%), acoustic neuroma surgery (10%), cancer (7%), iatrogenic injuries (7%) varicella zoster (7%), congenital palsy (5%), and Lyme disease (4%).12 About 60% of patients in both series were female. Because most patients with Bell palsy will recover to normal facial function, they are underrepresented in series from facial nerve centers. It is thought that roughly 70% of cases of facial paresis are caused by Bell palsy.
Nearly 10% of patients with Bell palsy have had a prior episode.13 Findings of studies are mixed as to whether the prognosis is worse for a second attack. Recurrent ipsilateral facial palsy necessitates a careful investigation. In one study, 20% of patients with a second bout of paresis on the same side were found to have a tumor along the course of the facial nerve.14
Classification of Peripheral Nerve Injury
Understanding the fundamentals of peripheral nerve injury provides a useful framework for thinking about how much recovery to expect and is critical to the correct implementation and interpretation of electrophysiologic (EP) facial nerve studies (see Electrophysiologic Testing section below). Seddon initially classified peripheral nerve injury into three types: (1) neuropraxia, (2) axonotmesis, and (3) neurotemesis.15 Sunderland expanded on Seddon’s work by further subclassifying the neurotemesis category, which resulted in five distinct degrees of nerve injury.16 Table 31.4 displays the theoretical characteristics of each type of nerve injury along with a range of possible outcomes from electromyography (EMG) and electroneuronography (ENOG).
Table 31.4
Classification and Characteristics of Facial Nerve Injury
Category | Degree of Injury | ||||
Seddon’s classification5 | Neurotemesis | Axonotmesis | Neuropraxia | ||
Sunderland’s classification6 | First degree Conduction block | Second degree Axonal continuity | Third degree Endoneural tubule | Fourth degree Funiculus | Fifth degree Complete nerve trunk |
Recovery6 | Complete <2 weeks | Complete or mild | Residual deficit | Some spontaneous recovery that is rarely useful | If untreated, rare, and residual deficit, if treated |
Wallerian degeneration5,6,12,15 | Does not occur | 6–21 days, often >14 days | 6–21 days often <14 days | 100% by 3–5 days | |
ENOG7–17,19–21 | Normal | There is a >50% chance of an incomplete recovery when the ENOG response is reduced by ≥90% | 100% denervation by 3–5 days | ||
EMG (resting)20–22,25, 29,30 | Absent pathologic spontaneous activity | Pathologic spontaneous activity is present after 14–21 days, which heralds a high probability (80 %+) of an incomplete recovery | |||
EMG (volitional)20–22,25,29–30 | Volitional responses can be intact, reduced, or absent. Early intact volitional responses at multiple sites or improvement in the firing pattern suggest a good outcome. Absent (or minimal) volitional predicts a poor recovery | No volitional activity |
Each nerve fiber consists of an axon that contains cytoplasm, aptly named axoplasm. The axoplasm conducts electrical impulses along the axon. The axon is surrounded by a myelin sheath made of Schwann cells (in peripheral nerves) and other connective tissues. This sheath is often referred to in the literature as the endoneurium, neural tubule, or endoneural tube. Several axonal sheaths are bundled and held together by connective tissues (called the perineurium) to form the funiculus or fascicle. Finally, several fascicles are held together by areolar connective tissue, which, in the facial nerve, becomes more compressed outside the temporal bone, where it is called the epineurium. The epineurium, perineurium, and endoneurium can be viewed as three “protective layers” of connective tissue that serve to shelter the axon (Fig. 31.2).17
Sunderland’s five degrees of nerve injury are based on the extent of damage to various functional anatomic components and layers of the nerve.
▪ First-degree injury (neuropraxia): First-degree injuries are the result of a conduction block that typically occurs after nerve compression or ischemia. There may be localized damage to the myelin sheath, but there is no axonal degeneration; axonoplasmic continuity remains intact distal to the lesion. Therefore, neuropraxic injuries continue to conduct a neural impulse if an electrical stimulus is delivered distal to the site of lesion. This is the basic premise on which ENOG testing is based. Recovery from this type of injury is spontaneous and complete.
▪ Second-degree injury (axonotmesis): In this degree of injury, complete interruption of the axoplasm contained within the axon is seen. The endoneural tubule, however, is preserved. Anterograde axonal degeneration (Wallerian degeneration) occurs, resulting in the peripheral end organ being isolated from its corresponding neuron. This loss of nerve supply to the end organ is referred to as denervation. Because the endoneurium remains intact, the axon can regenerate toward its original target through the intact tubule. This leads to a better prognosis in terms of recovering motor function following the injury. Preservation of the endoneurium prevents synkinesis.
▪ Third-degree injury (neurotemesis): Third-degree injury involves damage to the endoneural tube and its contents. Retrograde disturbances are more significant because regeneration can now occur across disrupted endoneural tubules. Axons may reach functionally related end organs, or they may enter totally foreign endoneurial tubes. This results in internal disorganization because some axons do not regenerate to their original end organs. The resulting abnormal healing creates a distorted and less efficient firing pattern, with the clinical manifestations of synkinesis and/or contracture. Synkinesis is the involuntary contraction of an erroneously reinnervated muscle during a contraction of the normally innervated muscle.17 For example, a patient’s eye may blink when she attempts to smile. Synkinesis can also manifest as increased baseline tone (contracture). Overall recovery is longer and usually incomplete in third-degree injuries.
▪ Fourth-degree injury (neurotemesis): Fourth-degree injuries involve damage to the funiculus and its contents. The entire funiculus is involved, and all bundles are breached. Funicular bundles become so disorganized that they are no longer distinguishable from the surrounding connective tissues of the epineurium. Large numbers of regenerating axons escape and infiltrate foreign tubes. Some spontaneous recovery can occur, but it is of little functional value.
▪ Fifth-degree injury (neurotemesis, complete nerve transection): A fifth-degree injury involves transection of the entire nerve trunk. The majority of axons do not reach their designated funiculi or endoneural tubule because of the separation of the nerve ends and scarring. Recovery will not occur without surgical intervention, and complete restoration of function is impossible, even if the nerve ends are repaired.
Clinical Features
History
One of the most important aspects of the clinical evaluation is to try to diagnose the cause of facial palsy. Many patients with facial paresis are told they have Bell palsy by their physicians when, in fact, their presentation is not consistent with Bell palsy. Bell palsy should progress from normal facial function to maximum facial weakness in less than 72 hours. Viral causes of facial paresis involve the entire hemiface, including the forehead. Varicella zoster can present identically to Bell palsy with delayed onset or no onset of a vesicular skin eruption. Lyme disease also presents identically to Bell palsy with occasional bilateral facial paralysis. Thus, all patients presenting with rapid-onset peripheral facial weakness in Lyme disease–endemic areas should be considered for this diagnosis as well.
Gradual-onset, recurrent or fluctuating facial paralysis is not Bell palsy and should raise suspicion of a neoplasm along the course of the facial nerve. The parotid bed and neck should be carefully palpated for asymmetry or masses that could indicate a neoplasm. These patients require imaging and should be referred to an otolaryngologist for further evaluation.
Physical Examination
Physical examination of the patient with facial paralysis should follow the regional approaches proscribed in the Sunnybrook and House-Brackman grading scales. Each “zone” should be evaluated for resting, dynamic, and synkinetic findings. Patients may present in the acute phase, shortly after paralysis or much later in the course when function has started to return.
Bell palsy involves all branches of the facial nerve. Weakness in only part of the face is not consistent with a viral etiology and suggests pathology involving specific distal branches of the nerve. The finding of involvement in just one part of the face is a particular cause for concern with regard to perineural invasion by facial cutaneous squamous cell carcinoma and should prompt a careful history and physical examination for any prior or current skin cancers. Facial weakness caused by perineural invasion can appear months to years after removal of the primary skin malignancy.
Forehead
The eyebrow and forehead are important for facial expression and impact a person’s perceived age. Forehead movement and creases will be intact in central facial paralysis but absent in complete peripheral paralysis. In children and young adults, forehead paralysis will not usually result in brow ptosis, whereas in older adults forehead paralysis is associated with severe brow ptosis. Patients with synkinesis will often exhibit subtle brow elevation with smile and eye closure that may be amenable to botulinum toxin injections.
Eyelids
Evaluation of the periocular region should follow a system based on anatomic zones and the three conditions that distinguish the phases of facial nerve recovery. In this chapter, brow evaluation is discussed separately (see Forehead section above).
Upper eyelids are primarily responsible for ocular surface protection but also provide a seal against liquids, keep light out, and move the tears across the ocular surface to the lacrimal puncta. Evaluation should note resting position, as well as gentle and forced closure. To assess synkinesis, the lids should be observed after asking the patient to smile. Lagophthalmos should be measured with the patient in the upright and supine positions. Many patients will demonstrate 2 to 3 mm of upright lagophthalmos but 8 to 19 mm in the supine position. This discrepancy may be greatest in patients who have had a gold or platinum weight implanted. The eyelashes should be assessed for lash ptosis, particularly if surgery is anticipated. Meibomian gland function should be assessed.
In the early phase, the ocular surface is at high risk for exposure keratopathy or frank corneal ulceration. The cornea and conjunctiva should be observed closely under the slit lamp, with both fluorescein and lissamine green staining. In the late phase, an increased tear lake may be seen as a result of synkinetic stimulation of the lacrimal gland, especially when eating (gustatory lacrimation).
The lower eyelids provide protection to the inferior one-fourth of cornea. They may be well positioned immediately after a paralytic insult but tend to become ectropic and retracted. The lid must be assessed for horizontal laxity, as well as anterior lamellar shortening, which may progress rapidly in the first few months of paralysis. They should be assessed at rest and with forced closure. Typically, the lower lid rises in the supine position, masking the inferior corneal exposure. Synkinesis manifests with horizontal shortening of the palpebral fissure and lower lid elevation, particularly with talking, smiling, or chewing.
Midface
The midface contains all of the lip elevators. Patients with complete facial paralysis are unable to smile on that side of the face, which makes social interaction more challenging. Buccinator weakness causes difficulty chewing on that side, as the food bolus cannot be held between the teeth, and flapping or “puffing out” of the cheek during speech. An underappreciated consequence of facial palsy is nasal obstruction, caused by deviation of the nose toward the intact side, as well as loss of muscular support around the affected nostril and nasal sidewall (Fig. 31.3).
It is common for patients with synkinesis to have decreased elevation of the corner of the mouth with smile because of synkinetic firing of the lip depressors (platysma, depressor anguli oris), risorius, and buccinator. The most severe form of this is a “frozen smile,” where the upward and downward force on the oral commissure is roughly equal and there is minimal movement of the mouth with attempted smile. Synkinetic contracture of the lip elevators also contributes to poor smile excursion and can cause mild to severe midface pain.
Lower Face
Lower facial paralysis is relatively well tolerated compared with paralysis of the periocular and midface regions. The most bothersome result is lack of lower lip depression making the smile asymmetric. In older individuals, platysma paralysis will make the appearance of the neck asymmetric as well.
Lower facial synkinesis can impact the ability to generate a smile, as discussed above. The mentalis muscle is commonly involved in synkinesis manifested as unsightly chin dimpling and/or lower lip elevation during smile and eye closure.
Facial Nerve Function Grading Scales
Clinical facial nerve grading scales provide a convenient language that providers can use to convey what they see on physical examination. They are also useful in outcomes research. The House-Brackmann18 and Sunnybrook scales (Fig. 31.4) are the two most commonly used physician-graded facial nerve grading scales in use today. They were developed in different ways by different types of clinicians, and for different purposes. House was a neuro-otologist interested in measuring what happens to facial function after different kinds of injury to the main trunk of the facial nerve. The original scale has since been updated to include a regional assessment of facial function.19 In contrast, the Sunnybrook system20 was developed by physical therapists and physicians interested in accurately measuring clinical change in facial function after an intervention meant to improve that function. As such, the Sunnybrook system is a more continuous scale that collects more finite information. Both the House-Brackmann and Sunnybrook scales are valid and reliable tools that are best used in their separate, intended ways.


Investigations
Electrophysiologic Testing
EP testing is a valuable, objective tool to quantify facial nerve function. Electroneurography (ENOG) and electromyography (EMG) are the two tests that are most useful today.
Electroneuronography
The ENOG examination was originally proposed and popularized by Esslen21–23 and Fisch23,24 in the late 1970s. The goal of ENOG is to measure the amount of neural degradation that has occurred distal to the site of nerve injury by measuring the muscle response to electrical stimulation of the main trunk of the facial nerve. ENOG measures the compound muscle action potential (CMAP) of the facial muscles following this electrical stimulus. The electrical stimulus is delivered distal to the site of nerve injury. The amount of denervation is represented by comparison of the CMAP from the affected side of the face to the nonaffected side. In effect, ENOG differentiates first-degree “conduction block” injuries (neuropraxia) from those that have developed Wallerian degeneration (second to fifth degree).
The CMAP represents the sum of the action potentials of all axons within a nerve. Normally, an electrical stimulus depolarizes all of the nerve fibers at roughly the same time, causing the distal motor units to fire synchronously.15 These action potentials would then be summated to create a CMAP much larger than the individual action potentials from single axons. Fig. 31.5A, portrays several near-simultaneous motor unit action potentials being summated into a compound response. If very few axons are stimulable, the compound action potential is smaller than expected (Fig. 31.5B).

Patients with facial neuromas or those recovering from a facial nerve injury often have fibers in different states of recovery.25 This means that the action potentials may not occur at the same time and will not summate and can, in fact, cancel each other creating a low CMAP (Fig. 31.5C). This can sometimes result in discrepancies between the ENOG and EMG responses. The implications for interpreting these discrepant results are discussed in the Interpretation of EMG section below.
Interpretation of Electroneuronography.
ENOG’s primary utility is in determining the long-term prognosis of facial function. Additionally, some authors have tried to use ENOG to determine which patients might benefit from surgical intervention such as decompression of the facial nerve.25 Patients who demonstrate facial paresis in the setting of a normal ENOG study (i.e., no signs of Wallerian degeneration) are likely to have a conduction block (first-degree injury) and will tend to have a satisfactory recovery.26
Electromyography
EMG is the recording of motor unit action potentials (MUAPs). MUAPs are the spikes in electrical activity generated when a motor unit fires. A motor unit consists of a motor neuron and the corresponding muscle fibers innervated by the neuron. Each motor unit consists of the neuron and its axon, which has multiple synaptic junctions that are affiliated with corresponding muscle fibers. These synaptic junctions are called myoneural junctions. Each myoneural junction and muscle fiber generates a small electrical potential when activated. The synchronized discharge arising from all of the axon’s myoneural junction potentials combine to form the larger MUAP.
EMG differs from ENOG most importantly in that EMG relies on the patient’s nervous system to generate an impulse proximal to the site of injury and to pass this stimulus through the facial nerve to the target muscle fibers, which is measured. ENOG is an artificially generated electrical impulse applied to the facial nerve distal to the site of injury.
Performing EMG.
Recordings are made from various mimetic muscles that are innervated by the facial nerve. Typically, five muscle sites are tested corresponding to the five main facial nerve distributions (frontal, zygomatic, buccal, marginal mandibular, and cervical). Electrical activity at each test location is recorded both at rest and during attempted volitional movement. Granger demonstrated that patients with voluntary EMG potentials in four to five muscles 72 hours after paralysis onset has a 91% chance of a favorable outcome.27 Abnormal resting potentials are a sign of neural degeneration and appear as early as 7 days28 but more frequently after 10 to 14 days postinjury.29,30
Interpretation of EMG.
Several authors have recommended using the presence or absence of pathologic spontaneous activity (fibrillation potentials and/or positive sharp waves) with a goal of separating out those patients who will recover spontaneously from those patients who will require further intervention.31–33 Under this system, a first-degree injury is defined as decreased or absent EMG activity without pathologic spontaneous activity after 10 to 14 days. Inversely, the presence of pathologic spontaneous activity along with decreased or absent volitional activity is categorized as a second or higher degree of injury and thus predicts a poor outcome. It is important to understand that this classification system is not valid until after 10 to 14 days when the abnormal spontaneous responses will appear.
Prognostic Value of EMG.
Volitional EMG responses at more than four of the five recording sites is very predictive of a good recovery (House-Brackmann I or II). Several studies have clearly shown the utility of EMG to prognosticate following facial nerve injury.31,33 The high positive and negative predictive values of EMG studies performed 2 weeks or later after the onset of injury suggests that EMG may be the study of choice for patients who present to the clinic several weeks after the onset of their injury, as well as for patients who are undergoing long-term monitoring.
EMG and ENOG findings associated with good and bad outcomes are summarized in Table 31.5.
Table 31.5
Good versus Bad Outcome Indicators
Good Outcome Indicators | Bad Outcome Indicators |
Normal ENOG after 14–21 days | Weakness at time of trauma |
Late-onset of Wallerian degeneration | Early-onset Wallerian degeneration |
Normal resting potentials on EMG after 14–21 days | Progressive decay of ENOG |
Early volitional motor units <4 days | Abnormal spontaneous activity on EMG after 14–21 days |
Improving volitional EMG | Delayed recovery > 3 months (Bell palsy) functional & EMG |
Volitional EMG recorded at 2 + sites (4–5 better) | No Volitional EMG or Volitional EMG only at 1 recording site |
Timing Considerations for ENOG and EMG
When performing ENOG and EMG, it is important to consider how much time has elapsed since the onset of the injury. Table 31.6 suggests a timeline for EMG and ENOG evaluations. Key issues related to timing of the evaluation include the following:
1. ENOG should never be performed earlier than 3 days following injury because abnormal ENOG responses rely on the onset of Wallerian degeneration, which requires a minimum of 72 hours to occur.
2. Early signs of denervation on ENOG are a poor prognostic sign, as they herald a more severe nerve injury.
3. EMG can be performed at any time after the injury. Early responses on voluntary EMG, especially at multiple locations, are a positive indicator for recovery and rule out complete nerve transection.
4. EMG’s prognostic value improves when testing can be deferred until 10 to 14 days after the onset of injury. This will allow signs of abnormal healing or abnormal resting potentials to occur. However, when facial nerve decompression is being considered, delaying treatment may compromise recovery.
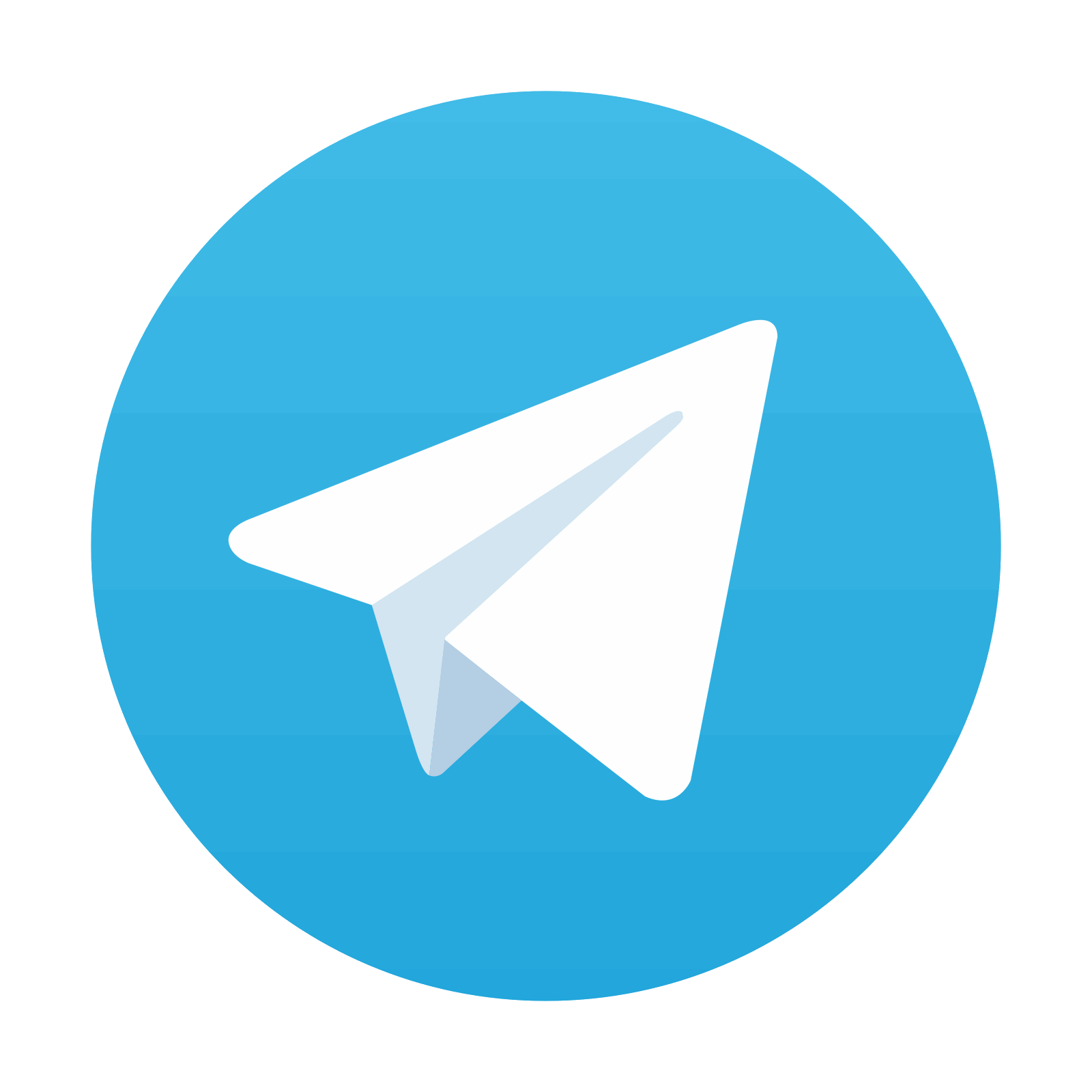
Stay updated, free articles. Join our Telegram channel

Full access? Get Clinical Tree
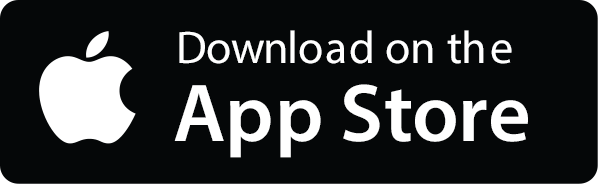
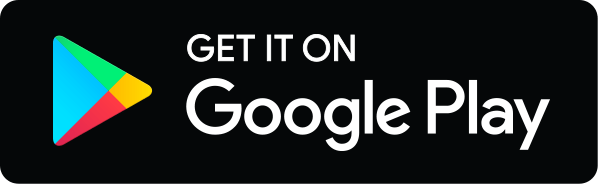