Excimer Laser Surface Ablation: Photorefractive Keratectomy (Prk) and Laser Subepithelial Keratomileusis (Lasek)
Scott Barnes
Dimitri T. Azar
The field of refractive surgery has been greatly impacted by the development of the excimer laser. This high-energy ultraviolet (UV) laser has been employed in various industrial and medical applications. The term excimer is a contraction of excited dimer, which was a term first used by photochemists in 1960 to describe an energized molecule with two identical components. However, rather than two identical components, most excimer lasers involve a high-energy complex (“excited complex”) of halogen and inert atoms of noble gases, achieved under a very high voltage electrical field (1,2). Such excited dimers are molecules that have minimal binding in the ground state but are more closely bound in a higher energy (“excited”) state. Various combinations of halogens and gases can produce dimers in wavelengths between 193 and 351 nm. This high-energy complex, being unstable, decays and releases energy in the form of a photon of UV radiation. These photons are then amplified, phased, and collimated to form a laser beam (1, 2, 3).
Originally developed in 1975 (4), the excimer laser was employed for research in physical chemistry and for various industrial uses, precisely etching a number of polymers (5, 6, 7, 8). This process was originally called ablative photodecompensation but has evolved into the term photoablation (3,9,10). The energy of a UV photon, excited between 193 and 351 nm, exceeds the covalent bond strength of many molecules; ablative photodecompensation directs this UV energy toward a specific molecule, breaking chemical bonds, which increases pressure in a confined volume thus ejecting the molecular fragments into the atmosphere (5,9,11,12).
Taboda and colleagues (13,14) reported the first use of an excimer laser on corneal tissue in 1981 at the U.S. Air Force School of Aerospace Medicine. In 1983, Trokel and colleagues (15) reported that UV light could predictably ablate corneal tissue while producing minimal damage to surrounding structures (16,17). Kruger and associates (18) evaluated four excimer generated wavelengths in their interaction with corneal tissue: argon-fluoride (193 nm), krypton-fluoride (248 nm), xenon-chloride (308 nm), and xenon-fluoride (351 nm). The 193-nm wavelength gave more precise and smoother results compared to the increased surrounding thermal damage noted with the longer wavelengths.
EXCIMER LASER ABLATION
The property of the excimer laser beam that makes its interaction with the stromal surface of the cornea unique compared to other lasers, such as those driven by yttriumaluminum-garnet (YAG) or CO2, is the ability to impart very high energy to such tissue without a correspondingly high thermal component (1, 2, 3,19). This is due to the ability of the mixture of a rare gas (argon, krypton, or xenon) and a halogen (fluoride, chloride, or bromide) to produce a wavelength (especially ArF = 193 nm), which is extremely well absorbed by the covalent bonds (carbon-nitrogen and carbon-carbon) found supporting the corneal epithelium and stroma (1, 2, 3).
Direct bond breakage with a high-energy photon is a photochemical interaction. Although the laser energy is largely converted to heat with typical thermal effects seen in the ejected fragments (20), minimal thermal injury to the adjacent tissue is seen, presumably due to both the highly specific and effective photon absorption and the rapid photoablation process (21). Measurements of intrastromal thermal gradients show that local temperature elevation increases with laser repetition rate, drops exponentially with increasing distance from the edge of the ablation zone, and does not exceed 5°C at the edge of the keratectomy (22). There is some controversy regarding the degree of photochemical versus photothermal mechanism with laser
ablation (23); shorter wavelengths such as 193 nm may involve a total photochemical process, whereas longer wavelengths, such as 248 to 308 nm, may involve a photothermal process. At any rate, short wavelength pulses delivered at a low repetition rate may help limit local heating, thereby producing less coagulation of protein adjacent to the ablation zone.
ablation (23); shorter wavelengths such as 193 nm may involve a total photochemical process, whereas longer wavelengths, such as 248 to 308 nm, may involve a photothermal process. At any rate, short wavelength pulses delivered at a low repetition rate may help limit local heating, thereby producing less coagulation of protein adjacent to the ablation zone.
As energy density increases above threshold, each pulse of laser light removes a constant amount of corneal tissue proportional to the amount of energy striking the cornea. The amount varies with the wavelength used to produce the light. Kruger et al. (18), Kruger and Trokel (24), and Puliafito et al. (25) studied ablation rates. They observed that in wavelengths greater than 193 nm, the threshold for corneal ablation increased as the laser repetition rate decreased. However, at 193 nm, the threshold was constant regardless of variance in repetition rate. This observation supports the photochemical theory of excimer ablation at 193 nm, with thermal mechanisms becoming a greater issue only at longer wavelengths.
Puliafito et al. (22) used high-speed photography to analyze excimer laser ablation and noted that fragments are ejected from the corneal surface in a mushroomshaped smoke plume for several hundred nanoseconds after the laser exposure. Increases in plume size and initial ejection velocity are seen with increasing exposure energy.
EXCIMER LASER SURFACE ABLATION: ANALYSIS OF LASER-CORNEAL INTERACTIONS
Use of UV radiation brings a concomitant concern of mutagenesis and carcinogenesis. Most carcinogens have been shown to be mutagens as well (26). UV radiation-induced mutation increased proportionally with DNA absorption (27). Due to the low density of keratocytes in the stroma, carcinogenesis resulting from stromal photoablation may be unlikely. Several studies have failed to show cellular mutations or carcinogenic changes associated with 193-nm irradiation. Nuss et al. (28) evaluated the repair of damaged pyrimidine dimers by observing unscheduled DNA synthesis after photoablation. Such DNA synthesis did not increase after 193 nm linear ablation compared to that seen following diamond knife incisions as a control. Interestingly, there was a statistically significant increase in such DNA synthesis after 248 nm irradiation. Theories for decreased toxicity with 193 nm include a general lack of cytotoxic breakdown products at that wavelength, damage that can be repaired by the molecule’s cellular environment, absorption of the wavelength by some type of protective protein coat, or that mutagenic repair processes are not possible due to the extent of tissue destruction caused by 193 nm energy (29).
Skin and corneal models further illustrate the influence of such high-energy irradiation. Skin irradiation with 193 nm light produced the least DNA damage and cytotoxicity, 248 nm energy produced the greatest damage, and 308-nm exposure resulted in a moderate degree of toxicity (29). Further, 193 nm irradiation of the cornea produces fluorescence between 295 and 425 nm, levels at which mutations or cataract development would be likely (30). However, the highly attenuated energy of such fluorescence makes the level of exposure 10,000 times lower than the minimum average annual exposure to solar UVB radiation. Trokel et al. (15) examined the results of 193 nm excimer ablation of the cornea in 1983. Puliafito and colleagues (31) observed the histopathology of linear corneal ablation using 193 and 248 nm. Both reports confirmed preservation of normal corneal microstructure adjacent to the ablation zone with 193 nm, and Puliafito’s study showed disorganization of such microstructure at least 10 μm into the adjacent stroma after exposure to 248 nm pulses. Further, electron microscopy revealed a submicrometer zone of electron density adjacent to the ablation site. Kerr-Muir and associates (32) postulated that this may represent a type of “pseudomembrane” that may “seal” cells and material transected by 193 nm ablation. This may account for the optically clear cornea adjacent to the 193-nm ablation zone and the loss of transparency in the cornea adjacent to the 248 nm ablation. Additionally, Peyman and colleagues (33) reported a significant coagulative effect, corneal necrosis, stromal opacification, and endothelial cell damage with 308 nm irradiation. Based on this and other supporting studies, argon-fluoride (193 nm) has become the most common gas mixture used in clinical lasers.
ENDOTHELIUM AND SURFACE ABLATION
Given the well-known findings of late-onset corneal edema following the initial Japanese technique of radial keratotomy involving incisions in the endothelium, such endothelial damage from excimer laser refractive surgery remains a major concern.
In spite of the near complete absorption of 193 nm photons within 1 μm of tissue, minor endothelial effects have been noted. Dehm and colleagues (34) reported minimal endothelial effects with 193 nm linear “incisions” to the same corneal depth used with diamond knife radial keratotomy (RK); the endothelial effects were similar to those seen in standard RK incisions. Interestingly, significant loss of endothelial cells was noted with 248 nm “incisions.” After surface ablation with 193 nm light, Hanna and associates (35) reported the presence of electron dense granules in rabbit endothelium, which migrated through Descemet’s membrane and eventually dissipated over several weeks. This phenomenon may be a transient pressureinduced injury experienced during ablation. The impact of
the excimer laser energy on the cornea produces an acoustic shock wave resulting in an audible snap. The resultant shock wave has been photographed in the air above the eye (36) and recorded underneath the cornea using piezoelectric transducers. Zabel and colleagues (37) have shown that this shock wave can generate up to 100 atm of pressure on the endothelium. However, no obvious disruption of the endothelium has been noted. In fact, acute morphologic studies of primate and human endothelium have failed to show endothelial changes unless the laser beam approaches Descemet’s membrane (38,39). Clinically significant endothelial cell loss after excimer laser photorefractive keratectomy (PRK) has not been recorded (39, 40, 41), and in fact, endothelial morphology seems to improve after PRK in contact lens wearers, perhaps reflecting the elimination of the lens.
the excimer laser energy on the cornea produces an acoustic shock wave resulting in an audible snap. The resultant shock wave has been photographed in the air above the eye (36) and recorded underneath the cornea using piezoelectric transducers. Zabel and colleagues (37) have shown that this shock wave can generate up to 100 atm of pressure on the endothelium. However, no obvious disruption of the endothelium has been noted. In fact, acute morphologic studies of primate and human endothelium have failed to show endothelial changes unless the laser beam approaches Descemet’s membrane (38,39). Clinically significant endothelial cell loss after excimer laser photorefractive keratectomy (PRK) has not been recorded (39, 40, 41), and in fact, endothelial morphology seems to improve after PRK in contact lens wearers, perhaps reflecting the elimination of the lens.
EXCIMER LASER RADIAL KERATOTOMY
Clinical excimer laser refractive surgery involved application within the known framework of RK. Although incisions are produced with diamond knives, laser RK actually involves excisions, as the tissue is actually removed through ablation. In 1985, Cotliar and co-workers (42) obtained approximately 5 diopters (D) of corneal flattening on enucleated human eyes with laser radial excisions. They showed that the excision depth and amount of flattening was proportional to the amount of laser energy. Steinert and Puliafito (43) developed a system to perform RK laser excisions on rabbit eyes in vivo. Aron-Rosa and colleagues (44) were the first to report outcomes of laser RK in a series of human eyes scheduled for enucleation; 2 to 4 D of flattening was attained with the central corneas remaining clear up to 3 weeks after ablation. Interestingly, to achieve similar degrees of corneal flattening, excimer linear excisions needed to be less deep than incisions with a knife, perhaps due to the ablation’s removal of tissue rather than the tissue splitting of standard RK.
The advantage of excimer laser versus standard RK was in the increased precision and reproducibility. Depending on delivery systems, laser RK could be an office-based procedure, with the patient in either the supine or upright position, under topical anesthesia. The potential for customized treatment patterns for individual refractive errors combined with possible real-time adjustments via corneal topography during the course of the treatment held great appeal. However, several concerns arose regarding the width of the excisions and their effect on the structural and optical integrity of the cornea. The expected epithelial plug filling the laser RK excisions would be present for months or years. The cornea would remain weakened as the epithelium gradually converted to a fibroblastic scar. Such scars may be more dense and produce more glare than those associated with standard RK. These concerns led to the abandonment of the excimer laser RK procedure in favor of a more novel approach to refractive ablation.
EXCIMER LASER PHOTOREFRACTIVE KERATOTOMY
The goal of direct ablation of the superficial central cornea over a wide area is to maintain clarity and optical function while creating a new anterior corneal curvature. Although this might more precisely be called laser anterior keratomileusis, it has become known as photorefractive keratectomy (PRK). To treat myopic refractive errors, corneal tissue is ablated centrally with progressively less removal toward the periphery, resulting in corneal flattening. Conversely, to treat hyperopic refractive errors, more corneal tissue is removed in an annular pattern in the periphery, resulting in corneal steepening. More complex patterns of preferential tissue removal are employed to address correction of astigmatism.
LASER DELIVERY SYSTEMS FOR PRK
Broad-beam delivery models generally employ a diaphragm system, which places a movable circular aperture in the laser path (45). This diaphragm operates similarly to that of a camera system, which can either expand or contract during the exposure. The central cornea receives ablation with every pulse, whereas the successive peripheral areas receive correspondingly less ablation. Combining the diaphragm with moving parallel slit blades can achieve elliptical or cylindrical ablations depending on the type of astigmatism. Treatment times are much faster with such systems, thereby lessening the chance of corneal dehydration, patient discomfort, and the tendency to drift from fixation. However, the resulting “step-like” ablations with broad-beam lasers may be a disadvantage. Although this can be minimized by moving the diaphragm with each pulse, the significance of this ablation pattern is debated. It is theorized that a step-like ablation may stimulate a more aggressive healing response.
A variant of the diaphragm system employs a series of fixed apertures (46). Moving the apertures, which range from small to large, through the beam with successive pulses will cause central corneal flattening in a similar manner as the expanding diaphragm system.
Another delivery model involves the use of an ablatable mask on or near the cornea (47). The mask is made of a synthetic material that is gradually ablated by the laser beam. The shape of the mask could be thought of as similar to a spherical or toric hard contact lens. As the laser pulses gradually break through the polymer, the underlying cornea will be ablated in a pattern mirroring the shape of the mask. The different shapes of the mask (myopic, hyperopic, cylindrical) are responsible for the different refractive
changes in the ablated cornea. Most mask systems are used in conjunction with a broad-beam system and therefore have the attendant advantages and disadvantages.
changes in the ablated cornea. Most mask systems are used in conjunction with a broad-beam system and therefore have the attendant advantages and disadvantages.
Scanning spot or scanning beam delivery models (48,49) use small spots (0.2 to 1 mm) or a small rectangular beam to rapidly move across the corneal surface in a variety of patterns depending on the capabilities of the motor and computer control mechanisms. The main advantage of such systems centers on the potential for a smoother ablation due to the overlapping beam and multidirectional scanning. Another advantage is the possibility of large treatment zones with multiple ablation profiles without the need for masks or apertures. The flying spot system is particularly suited for customized ablations by microprocessor-controlled “pixel-by-pixel” corneal ablations (50). However, the scanning system (particularly the flying spot) ablates such a small area of the cornea with each pulse that much longer treatment times are necessary as compared to the broad-beam systems. This longer treatment time may be associated with corneal dehydration and increased patient drift; however, active eye tracking devices, infrared or video-based, have been and are being developed for use in such systems.
SURFACE ABLATION: EARLY ANIMAL STUDIES OF PRK
The difficulty in finding a suitable and practical animal model to simulate the human cornea has hindered the study of the optical changes induced by excimer ablation. Of the animals available for practical laboratory evaluations, only primate and cat corneas have a Bowman’s layer visible on light microscopy, and this is markedly thinner than in the human. The aggressive wound healing response demonstrated by most animal species, as compared to humans, has made direct laboratory comparison with, and prediction of, human responses questionable.
Marshall and colleagues (51,52) ablated 7-mm disks from monkey and rabbit corneas; smoother ablation surfaces and borders were found with 193 nm than those seen with 248 nm. The rabbit corneas developed much more haze than seen in the single monkey cornea studied. The average time for reepithelialization was 4 days. Krauss et al. (3) summarized unpublished data from Mandel and co-workers, who found opacification in the relatively deep ablation zone of monkey corneas within 1 week after exposure. Partial regression of the initial flattening as well as progressive corneal thinning was noted over several months. When using 3 mm disk ablations in monkeys, Marshall et al. (52) noted that although all ablation zones were initially clear, all but the shallowest ablations showed some degree of opacification by 1 month. However, by 6 months, all but the deepest ablations were free of haze. In these monkeys, the reepithelialization was complete by 1 to 2 days. Pathology analysis showed a stromal reaction with an increase in keratocytes and vacuole formation. However, by 8 months, the only evidence of disorganization was found in the stromal fibers under the epithelium. Marshall’s group theorized that these disorganized stromal fibers acted as a “substitute” Bowman’s membrane capable of supporting reepithelialization.
Hanna et al. (35,48,49) used a moving slit-laser system with rabbit corneas. Variable amounts of corneal haze were noted on pathology examination. Hanna et al. attributed this effect to variations in laser technique or uncontrolled differences in wound healing. Del Pero and colleagues (53) performed 4- to 7-mm myopic and hyperopic ablations in monkey corneas. All corneas were noted to have some degree of anterior stromal haze but this had diminished in all cases by the 12th month. An initial, unintended overcorrection was noted with gradual regression observed over a number of months. Fantes et al. (54) and McDonald and colleagues (45) confirmed the previously observed healing effects including the regression of the initial overcorrection, the rapid reepithelialization, the appearance and regression of stromal haze, and the increased activity of keratocytes within the ablation zone.
Tuft and colleagues (55) examined their results after ablations with rabbit corneas. A specific fluorescent dye was used to demonstrate new connective tissue at the stromal base with accompanying epithelial hyperplasia. The development of the connective tissue was reduced with the use of topical steroids after the ablation; however, the epithelial hyperplasia appeared unchanged. Goodman and co-workers (56) reported very different results with their contoured myopic ablations in rabbit corneas. No evidence of epithelial hyperplasia or new collagen formation was found in ablation depths under 50 μm. However, corneal opacification was noted in all ablations to 100 μm.
SURFACE ABLATION: EARLY HUMAN TRIALS OF PRK
With the nearly universal finding of at least some degree of corneal haze and resultant opacification in the animal studies, the human trials of PRK understandably focused on the incidence and time course of haze and permanent scarring. Cintron (57) asserted that in contrast to rabbit corneas, known to respond vigorously to wounds by synthesizing scar tissue, higher vertebrates, such as humans and monkeys, are more sluggish in healing. Although this might address some concerns related to the rabbit corneas, it did not help explain the haze and scarring that was seen in the studies involving monkeys.
In general, corneal clarity after PRK in humans is significantly better than that which was seen in the animal studies, including the nonhuman primates. The improved lasers and delivery methods of the commercial ophthalmic laser
systems, compared to using scientific excimer systems in an optical research bench or even a prototype ophthalmic laser system, may contribute to this favorable observation.
systems, compared to using scientific excimer systems in an optical research bench or even a prototype ophthalmic laser system, may contribute to this favorable observation.
L’Esperance et al. (58) and Taylor and colleagues (59,60) reported their results with the first PRK ablations on humans in the United States. The 5-mm ablations in 1987 were limited to blind eyes or eyes scheduled for enucleation. The initial results were similar to those seen in the animal studies with a 3-day reepithelialization, no recurrent erosions, minimal inflammation, and a mild anterior haze noted by the second week. The initial refractive correction was noted to regress by about 33%, and the histopathology on the enucleated eyes suggested new collagen formation. McDonald and co-workers’ (61) initial myopic PRK in humans involved nine blind eyes whose range of refractive error was −1.74 to −11 D. Rapid reepithelialization was attained by the fifth day with no recurrent erosions. A third of the corneas were clear at the 6-month follow-up examination, whereas two thirds had only minimal haze. Once again, the initial refractive results in the 5-mm ablations were followed by a regression proportional to the degree of applied ablation.
Seiler and colleagues (62) published their results with PRK in blind and normally sighted humans. Reepithelialization occurred within 3 days and the initial overcorrection was followed by subsequent regression. The 3-month follow-up revealed that 12 of the 13 sighted eyes achieved correction within 1 D of that intended; 10 of those 13 eyes kept that level of correction at the 6-month exam. Stromal haze was present in most eyes at the 6-month exam but judged to be clinically insignificant. One patient’s complaint of halos around lights at night after his 3.5-mm-diameter ablation illustrates the possible benefit of a larger ablation zone. Although large ablation zones would tend to reduce some of the glare or halos, the size of ablation is dictated by the mechanics of the laser delivery system and the concern of corneal effects with wider and deeper ablations.
CORNEAL HAZE AFTER PRK
The depth of the ablation for myopic correction increases linearly for the dioptric power but as a square exponential function of the diameter of the optical zone (63). Most of the early clinical studies indicated that the frequency and severity of corneal haze increased proportionally to the degree of attempted correction, especially over −6 D (64, 65, 66, 67). Taylor and colleagues (68) reported an essentially linear relationship with mild corneal haze and attempted correction; however, more severe haze (grade II or higher) was uncommon in corrections under −10 D.
Although animal studies and early clinical observations in humans supported the idea that haze was correlated with ablation depth, problems with smaller optical zones began to surface. Halos and glare in dim light combined with the lack of margin for error in centration with small optical zones led researchers to increase the ablation zone to 6 mm. Interestingly, the human corneas not only tolerated the increased ablation zone and resultant increased depth but actually showed a reduced incidence of haze, greater predictability of outcomes, and less tendency of regression in refractive effect (69, 70, 71, 72, 73, 74, 75, 76). Anecdotal as well as published evidence has suggested that a large optical zone in conjunction with an aspheric peripheral “blend” zone will give improved results (73); however, other reports fail to confirm a beneficial effect from a multizone peripheral contour (76). At any rate, in the human cornea there appears to be a serendipitous effect of less corneal haze and improved predictability and stability associated with a 6-mm treatment zone in spite of the increased ablation depth (Fig. 75-1)
OBJECTIVE ANALYSIS OF CORNEAL HAZE AFTER PRK
Lohmann and co-workers (77,78) reported their observations using techniques for quantifying light scattering based on the Scheimpflung principle. Excellent visual function was demonstrated in PRK patients compared with spectacle- or contact lens-wearing controls. Their report further identified the importance of differentiating “back-scattering,” which is visible to the observer, from “forward scattering,” which may be noticed by the patient. Harrison and colleagues (79) found no evidence of forward scatter in 24 PRK patients 1 month after treatment; however, no patient had a haze score greater than 0.5. This was in contrast to Lohmann’s findings of significant forward scatter 1 month after treatment, but their study used 4-mm optical zones compared to 6-mm zones used in the Harrison study.
Braunstein and co-workers (80) measured the backscatter and noted that such light was well correlated with the degree of clinical haze. It was further noted that ablations of less than 80 μm have much less backscatter than those with greater depths. Schallhorn and colleagues (81) used the stray-light meter developed by van den Berg and Ijspeert (82) to objectively measure the forward-scattered light falling on the macula in patients after PRK with 6-mm ablation zones. There was a 5% average increase in forward scatter at 1 month, but the measurements at 3, 6, 9, and 12 months were unchanged from preoperative levels. When measured after pupil dilation, however, the forward scatter was increased compared to the preoperative levels through the sixth month, but had returned to baseline by the 12-month exam.
Maldonado and associates (83) examined slit-lamp photographs of 40 eyes after PRK utilizing computerized digital analysis. Backscattered light showed a weak but positive correlation with the amount of haze and the degree of correction ranging from −6 to −22 D. Although the initial
appearance of haze appeared quite uniform throughout the ablation zone, there was a significantly greater reduction in central as compared to peripheral haze throughout the postoperative course.
appearance of haze appeared quite uniform throughout the ablation zone, there was a significantly greater reduction in central as compared to peripheral haze throughout the postoperative course.
![]() FIGURE 75-1. Postoperative haze formation. Subepithelial haze may present by 1 month postoperatively, peak between 3 and 6 months, and gradually subside by 12 to 18 months. The slides represent trace (A), mild (B), and moderate haze (C). (From Thompson V, Seiler T. Excimer laser photorefractive keratectomy for myopia. In: Azar DT, ed. Refractive surgery. Stamford, CT: Appleton and Lange, 1997:427, with permission.)(see color image) |
BASIC TECHNIQUES FOR EXCIMER LASER PRK
Mechanical Epithelial Removal
To alter the refractive shape of the cornea, the stroma must be ablated. There are numerous methods of removing the overlying epithelium. One common method employs either a blunt or sharp surgical blade (Fig. 75-2A). The epithelium is manually removed, exercising caution not to nick Bowman’s layer. The periphery is removed first, preserving the central epithelium until later in order to prevent dehydration of this critical area of ablation.
A variant of the manual debridement involves the use of a small, battery-operated rotating brush (Fig. 75-2B). This modification of an “electric toothbrush” used a brush head slightly larger than the desires ablation zone. The brush is designed to initially remove the peripheral epithelium, occasionally leaving a small central zone that can be easily removed with a blunt instrument (Fig. 75-2C,D).
Chemical Epithelial Removal
The desmosomes and hemidesmosomes of the epithelium can be loosened or broken with various chemical agents. Local application over the desired area of ablation may be accomplished through pledgets or cellulose sponges. Chemical leakage has led to the development of numerous “wells” that maintain the agent within the desired boundaries. Topical anesthetics (proparacaine and tetracaine) are effective but take 5 to 10 minutes to loosen the epithelium. Lidocaine, cocaine, and aminoglycosides have also been used but have largely been supplanted by ethanol. A 15% to 20% dilution can rapidly loosen the epithelium, often in 15 to 20 seconds.
Laser Epithelial Removal
The excimer laser itself can be used to remove the epithelium. This process, termed transepithelial ablation, involves ablating the epithelium over an area slightly larger than the desired stromal treatment zone. The technique employs the visualization of a slight cobalt blue fluorescence seen with ablation of epithelium but not stroma. The earlier, broad-beam laser systems were better suited for this approach as they could easily create a planar ablation also known as PTK. As epithelium can vary in thickness over the surface of the
cornea, a modification of the laser removal has emerged. Stopping the ablation just prior to, or at the moment of first penetration through the epithelium and then manually removing the residual basal layer has been termed laser-scrape. This technique avoids placing an irregular ablation pattern on the stroma as the laser “breaks” through a nonuniform epithelial layer. Flying spot laser systems and increasingly large treatment zones without accompanying large PTK options have limited the usefulness of this technique.
cornea, a modification of the laser removal has emerged. Stopping the ablation just prior to, or at the moment of first penetration through the epithelium and then manually removing the residual basal layer has been termed laser-scrape. This technique avoids placing an irregular ablation pattern on the stroma as the laser “breaks” through a nonuniform epithelial layer. Flying spot laser systems and increasingly large treatment zones without accompanying large PTK options have limited the usefulness of this technique.
![]() FIGURE 75-2. Technique of photorefractive keratectomy (PRK). Epithelium may be manually removed using a sharp blade (A) in a gentle “pulling” fashion or by using a rotating soft brush (B) similar to an “electric toothbrush.” The brush technique often leaves a residual central portion of epithelium (C), which can easily be removed with a blunt “hockey-stick” blade (D). The exposed Bowman’s membrane, and underlying stromal bed, is then ablated (E). A bandage contact lens is placed over the ablated surface during the initial phase of reepithelialization (F). (Fig. 75-2A from Thompson V, Seiler T. Excimer laser photorefractive keratectomy for myopia. In: Azar DT, ed. Refractive surgery. Stamford, CT: Appleton and Lange, 1997:423, with permission.) |
Laser Ablation
After Bowman’s layer is exposed, the excimer laser is applied directly to the surface in order to remove the appropriate tissue (Fig. 75-2E). In recent years, numerous modifications have been made regarding the actual mechanics of laser ablation, some of which were previously covered in the section on laser delivery systems. Myopic ablations involve primarily the central cornea, hyperopic ablations are more peripheral-based, and astigmatic ablations involve a combination. More precise applications of laser ablation have been accomplished through various eye-tracking systems (i.e., video based, radar based, or infrared based).
Postablation Treatment
The ablated surface is then covered with a bandage contact lens and postoperative medications are begun (Fig. 75-2F). Topical steroids and antibiotics are mainstays of treatment. Nonsteroidal antiinflammatory drops are to be used with
caution. Oral narcotics may be necessary in the first few days. The epithelial defect resolves over 3 to 7 days, at which time the contact lens is removed. The antibiotics are discontinued after the epithelial defect heals and a slow taper of the steroids is thought to reduce the incidence of visually significant subepithelial haze.
caution. Oral narcotics may be necessary in the first few days. The epithelial defect resolves over 3 to 7 days, at which time the contact lens is removed. The antibiotics are discontinued after the epithelial defect heals and a slow taper of the steroids is thought to reduce the incidence of visually significant subepithelial haze.
RESULTS OF HUMAN PRK TRIALS
In the United States, about 2000 patients were treated with the excimer laser as part of the clinical studies supervised by the Food and Drug Administration (FDA). In the Summit phase III study (84), 90.7% of patients had uncorrected visual acuity of 20/40 or better, 66.3% achieving 20/20 or better, and less than 1% of eyes lost two lines of best corrected acuity. In the VISX FDA study (85), 85% of eyes had an uncorrected acuity of 20/40 or better, with only 1% losing two lines of best corrected visual acuity. Several other studies have followed eyes for at least 12 months after PRK (86, 87, 88, 89, 90, 91, 92, 93, 94, 95, 96, 97, 98, 99, 100, 101, 102, 103, 104, 105, 106, 107). The number of eyes with uncorrected acuity of 20/40 or greater varied from 27% to 100%, with 34% to 100% of eyes within 1 D of emmetropia. Eyes losing two or more lines of best corrected acuity varied from 0 to 22%.
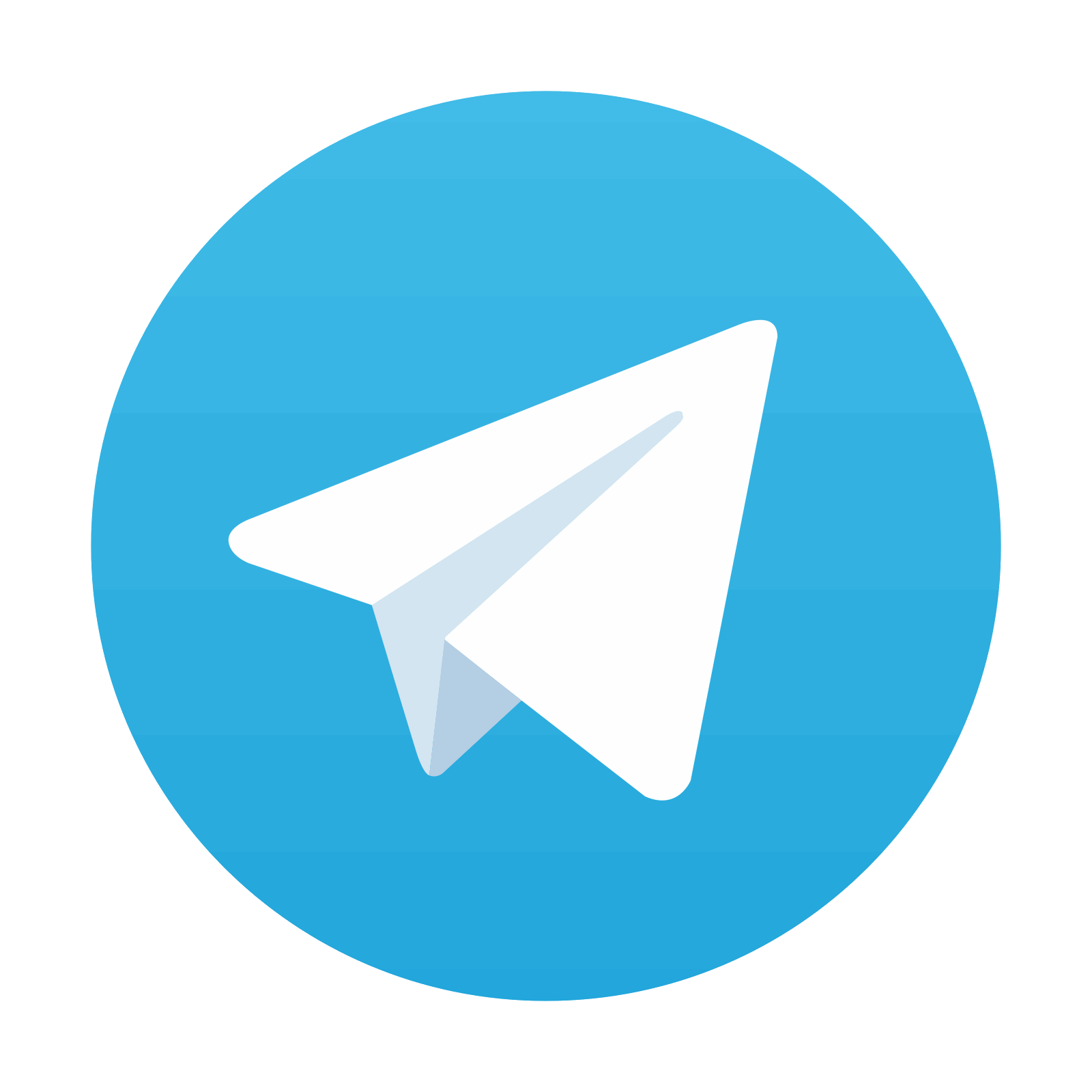
Stay updated, free articles. Join our Telegram channel

Full access? Get Clinical Tree
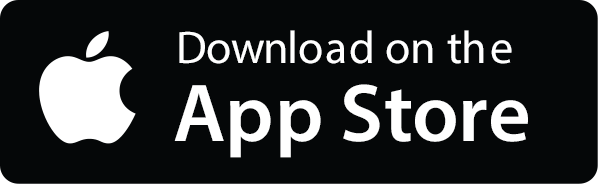
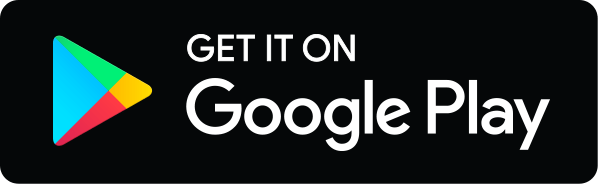