Fig. 5.1
Diversity of morphotypes in basal primates. Displayed are representatives of the Strepsirrhini: (A) the nocturnal Aye-aye (Daubentonia madagascariensis, Daubentoniidae); (B) the diurnal Coquerel’s sifaka (Propithecus coquereli, Indriidae); (C) the nocturnal western woolly lemur (Avahi occidentalis, Indriidae); (D) the diurnal ring-tailed lemur (Lemur catta, Lemuridae); (E) the nocturnal gray mouse lemur (Microcebus murinus, Cheirogaleidae); (F) the nocturnal Milne-Edwards’ sportive lemur (Lepilemur edwardsi, Lepilemuridae); (G) the nocturnal greater slow lori (Nycticebus coucang, Lorisidae); (H) the nocturnal northern lesser galago (Galago senegalensis, Galagidae); and as a representative of the basal Haplorhini, (I) the Lariang tarsier (Tarsius lariang, Tarsiidae). (Photographs: A, C, D, E, G by E. Zimmermann; B, F by M. Hokan; H by H. Zimmermann; I by S. Merker)
This chapter gives a brief historical summary of research on basal primate vocalizations and then focuses on selected taxa for insight into the diversity, flexibility, and complexity of their acoustic communication systems. Cross-taxa variation in acoustic space is further explored with a comparative bioacoustic approach to assess the acoustic parameter space that is open for signal evolution. Likewise, a comparative approach is used to explore two of the most influential hypotheses explaining the evolution of primate acoustic communication: the phylogenetic hypothesis and the social complexity hypothesis. Finally, perspectives for future research will be addressed, particularly as relevant to modeling the evolutionary origins of primate vocal communication, including speech and language in humans.
5.1.2 Ecological and Social Challenges Shaping Vocal Communication in Basal Primates
Strepsirrhines and tarsiers were neglected groups of primates for a long time. Over the last few decades, however, empirically driven research on these groups has increased and revealed a unique species richness in different phylogenetic groups. Fascinating adaptations to various ecological and social challenges have been discovered, in particular, for nocturnal Malagasy lemurs (Mittermeier et al. 2010; Zimmermann and Radespiel 2014; Lehman et al. 2016), African galagos (Butynski et al. 2013), and Asian tarsiers (Groves and Shekelle 2010).
All tarsiers and most strepsirrhine species are arboreal and are adapted to life in complex, three-dimensional environments such as tropical rainforest, dry deciduous forest, and gallery/savannah forest (Table 5.1). Ring-tailed lemurs (Lemur catta) and some true lemurs (Eulemur spp.) represent the few basal primates that are semiterrestrial (Ward and Sussman 1979). With regard to moving and foraging in a three-dimensional world, Malagasy lemurs exhibit greater diversity in activity and locomotion patterns, diets, body sizes, and social life compared to African and Asian lorisiforms and tarsiers. Members of eight phylogenetic groups (genera) of the Malagasy lemurs and all members of the Lorisiformes (Table 5.1) are adapted to a nocturnal life, and all tarsiers are suggested to be secondarily nocturnal (descendants of a diurnal ancestor) (Martin and Ross 2005). Members of four phylogenetic groups of the Malagasy lemurs are diurnal (active solely during the day), and members of two groups became cathemeral (activity distributed approximately evenly throughout the 24 h of the daily cycle, or significant amounts of activity, particularly feeding and/or traveling, occur within both the light and dark portions of the 24-h cycle) (see Tattersall 1979).
Table 5.1
Vocal repertoire, acoustic spaces, and some eco-ethological features in basal primatesa,b
Common and Latin name | VRS | AS (Hz) | Clade | Family | FU | BM (g) | PR | AC | SOS | HAB | LOC | IIC | EC | REF |
---|---|---|---|---|---|---|---|---|---|---|---|---|---|---|
Aye-Aye Daubentonia madagascariensis | 6 | 174–9,200 | Le | D | 1 | 2775 | l | N | s | DDF, RF | QRL | creee /screech | 1–3 | |
Western Woolly Lemur Avahi occidentalis | 5 | 415–4,659 | Le | I | 3 | 912 | m | N | p | DDF | VCL | whistle | AC | 1, 4–7 |
Verreaux’s Sifaka Propithecus verrauxi | 6 | Le | I | 5,5 | 3250 | m | D | g | DDF, HF, SF | VCL | chorus | 1, 8–10 | ||
Silky Sifaka Propithecus candidus | 10 | Le | I | 5,5 | m | D | g | RF | VCL | chorus | 1, 11 | |||
Indri Indri indri | 9 | 106–1,237 | Le | I | 4 | 9,500 | l | D | p | RF | VCL | contact-seeking whistle | duet/chorus | 1, 12–15 |
Milne-Edwards’s Sportive Lemur Lepilemur edwardsi | 9 | 633–5,641 | Le | Le | 1 | 931 | m | N | p | DDF | VCL | screech | duet | 1, 9, 16–18 |
Grey Mouse Lemur Microcebus murinus | 10 | 8,000–40,000 | Le | Ch | 1 | 54 | h | N | s | DDF, SF | QRL | whistle | AC | 1, 19, 20 |
Coquerel’s Giant Mouse Lemur Mirza coquereli | 10 | 282–10,000 | Le | Ch | 1 | 310 | m | N | s | DDF | QRL | whistle | AC | 1, 21, 22 |
Western Fat-tailed Dwarf Lemur Cheirogaleus medius | 8 | 4,000–34,000 | Le | Ch | 1 | 195 | h | N | p | DDF | QRL | whistle | AC | 1, 22, 23 |
Red-fronted Brown Lemur Eulemur rufifrons | 8 | Le | L | 8,7 | 2250 | m | C | g | DDF, RF | QRL | 1, 24 | |||
Black Lemur Eulemur macaco | 13 | Le | L | 10 | 2050 | m | c | g | RF | QRL | 1, 25 | |||
Crowned Lemur Eulemur coronatus | 10 | 900–5,352 | Le | L | 6,2 | 1650 | m | c | g | RF | QRL | 1, 26 | ||
Black-and-white Ruffed Lemur Varecia variegata | 12 | Le | L | 4,7 | 3350 | l | d | g | RF | QRL | mew | chorus | 1, 12, 27 | |
Ring-tailed Lemur Lemur catta | 22 | 240–8,320 | le | L | 14,5 | 2200 | m | d | g | DDF | QRL, ST | infant contact call; mew | chorus | 1, 28 |
Greater Slow Loris Nycticebus coucang | 8 | 1,100–7,400 | Lo | Lo | 1 | 687 | n | p | RF | QR | click, tsic | AC | 29–31 | |
Southern Lesser Galago Galago moholi | 18 | 300–2,800 | Lo | G | 1 | 153 | n | s | DF, SF | VCL | click | AC | 32, 33 | |
Northern Lesser Galago Galago senegalensis | 17 | 100–8,100 | Lo | G | 1 | 313 | n | s | DF, SF | VCL | zek | AC | 32–34 | |
Demidoff’s Dwarf Galago Galagoides demidovii | 8 | 400–1,600 | Lo | G | 1 | 58 | n | s | DF, SF, RF | VCL | click | 23, 32, 33, 35 | ||
Thick-tailed Greater Galago Otolemur crassicaudatus | 15 | Lo | G | 1 | 1175 | n | s | DF, SF | QRL | click | 32, 36–38 | |||
Northern Greater Galago Otolemur garnetti | 11 | 215–1,539 | Lo | G | 1 | 836 | n | s | DF, SF | QRL | click | 32, 37, 39, 40 | ||
Spectral Tarsier Tarsius tarsier | 8 | 3,000–12,000 | T | T | 1 | 112 | n | p | RF | VCL | whistle | duet | 41–43 | |
Philippine Tarsier Carlito syrichta | 7 | 9,752–22,000 | T | T | 1 | 128 | n | s | RF | VCL | 44–47 |
Strepsirrhines show a rich variety of adaptations for locomotion (Fleagle 2013), from climbing and running quadrupedally (with no adaptations for leaping) to quadrupedal running, climbing, and leaping to specialized vertical clinging and leaping (Table 5.1). In contrast, all tarsiers are highly specialized for vertical clinging and leaping. Strepsirrhine primates, particularly lemurs, have developed a richly diverse diet, whereas all tarsiers are dietary specialists, feeding primarily on arthropods and small vertebrates. Habitat type, activity pattern, and the distribution of food are suggested to have major implications for foraging strategies, predator avoidance, sociality, infant care and, thereby, communication and cognition in primates (also see Ramsier and Rauschecker, Chap. 3; Snowdon, Chap. 6; Zuberbühler, Chap. 7).
Predation risk in basal primates is much higher than in monkeys and apes, in part because of their small to medium body size (Gursky and Nekaris 2007; Scheumann et al. 2007a; Fichtel 2012). Body sizes in lemurs vary about 300-fold from the smallest bodied extant primates, the mouse lemurs (30–60 g), to the largest bodied extant strepsirrhine, the indri (Indri indri, 9,500 g) (Table 5.1). In contrast, Lorisiformes (55–1,220 g) and Tarsiformes (110–140 g) exhibit much narrower body mass variations. Known predators of basal primates include carnivores, raptors, and reptiles such as snakes and monitor lizards. Since smaller bodied species have more predators than larger bodied ones, selection should favor crypsis, which also affects communication strategies.
In comparison to monkeys and apes, basal primates display a unique degree of flexibility in their patterns of social life (Table 5.1). All nocturnal strepsirrhines and tarsiers live in social networks with varying degrees of social cohesiveness, and they often have an individualized neighborhood system (as defined by Richard 1985; Radespiel 2000) in a transition state between solitariness and gregariousness (Müller and Thalmann 2000; Kappeler 2012; Lehman et al. 2016). Adults may travel or forage solitarily, but they often live in stable pairs, mixed-sex groups, or kin-related female groups in which members of social units are socially bonded and share unit-exclusive and unit-specific sleeping sites. If pair partners establish long-term pair bonds and share pair-exclusive home ranges and often sleeping sites but forage apart from each other, this social organization is termed a dispersed pair. Other nocturnal taxa have pair partners that also forage together, and that social organization is termed a cohesive pair. Cathemeral and diurnal lemurs are usually organized as cohesive pairs and families; only the diurnal ring-tailed lemurs are known to establish larger multi-male, multi-female groups, sometimes with more than one matriline. These varying degrees of sociality in basal primates pose specific social challenges that may shape the evolution of acoustic signaling systems.
Infant care patterns are more flexible in strepsirrhines and tarsiers than in monkeys and apes. In tarsiers and most nocturnal strepsirrhines, females leave their infants cached at safe shelters (e.g., tree holes, nests, or dense vegetation) for extended periods of time while foraging (Ross 2003; Zimmermann and Radespiel 2013). In contrast, two nocturnal genera of strepsirrhines, as well as most cathemeral and diurnal lemurs, maintain close body contact with clinging infants during foraging. These distinctions in infant care patterns are linked to specific challenges in the social and ecological world that these primates have overcome by evolving appropriate communication strategies in the acoustic domain. Taken as a whole, their basal phylogenetic status, substantial ecological diversity, and unique adaptations make basal primates excellent candidates for exploring the evolutionary origins of primate acoustic communication.
5.1.3 History of Research on Acoustic Communication in Basal Primates and the Discovery of Ultrasound in Vocalizations
The study of the origin and evolution of display behavior began more than 150 years ago with Darwin’s groundbreaking work on the Expression of the Emotions in Man and Animals (Darwin 1872). However, it was not until the middle of the 20th century that systematic research on the vocal expressions of basal primates began. In his influential work on the calls and facial expressions among primates, Andrew (1963) was the first to compare the vocalizations of primates from different clades, including some strepsirrhines and tarsiers, relying on new technologies that enabled researchers to record, visualize, measure, and quantify vocalizations.
More than ten years later, Klopfer (1977) stated in his review that although several descriptions of the vocalizations of basal primates were available, systematic studies on their roles in communication were not yet published. About the same time, research teams around the world were starting the first field projects on the ecology and behavior of free-ranging strepsirrhines in western, eastern, and southern Africa (Bearder and Doyle 1974; Petter et al. 1977; Nash and Harcourt 1986) and on the island of Madagascar (Jolly 1966; Charles-Dominique and Martin 1972), as well as projects studying tarsiers in the Philippines (Niemitz 1984), with the goal of illuminating the biology of these hitherto neglected primates. Petter and Charles-Dominique (1979) were the first to provide a catalogue of spectrographically presented sounds of all major groups of strepsirrhines categorized according to their presumed functions. Shortly afterwards, Zimmermann (1981) presented the first empirical evidence for ultrasound (>20 kHz) in primate vocalizations in the infant isolation calls of the Lorisiformes. Cherry et al. (1987) then documented ultrasound production by Lemuriformes, and recently, Ramsier et al. (2012b) described ultrasound production by Tarsiiformes.
5.2 Diversity and Flexibility of Vocalizations
In recent decades, field and laboratory research has revealed that basal primates have evolved a remarkable diversity of vocalizations with regard to acoustic structure, reflecting a much broader frequency range that was available for signal evolution than in monkeys and apes.
5.2.1 General Remarks on the Cross-Taxa Diversity of Vocalizations
As outlined in Sect. 5.1.2, basal primates differ more in activity patterns and social cohesiveness within their social networks than monkeys and apes; nevertheless, they may establish individualized and permanent social bonds. Consequently, these primates face fundamental challenges to survive and reproduce, such as avoiding predators, limiting the potential for costly conflicts between competitors within and, if in sympatry, between species, coordinating movements in time and space while searching for common sleeping and/or feeding sites and reunions of mothers and infants, mates, pairs or groups, in particular when members are dispersed during foraging. In response to these challenges, a rich diversity of vocalizations evolved for acoustic signaling in basal primates (Table 5.1 and Sect. 5.2.2).
To the human ear, the vocalizations of basal primates sound fairly mysterious and even atypical for primates. The series of short, broadband, and high-frequency click, tsic, or zek noises (Fig. 5.2) used as infant isolation calls by bushbabies and lorises sound like high-frequency human tongue clicks, whereas the series of short, broadband, and low-frequency clicks given during grooming in lemurs (Scheumann et al. 2007b) sound like a purring cat. A series of low-frequency, broadband, and noisy grunts and growls (e.g., Zimmermann 1995a), which sound like hissing cats or growling/snarling dogs and acoustically mimic large and dangerous carnivores, occur across all nocturnal strepsirrhines and are associated with threatening situations. The cries, whines, or barks of the bushbabies (Otolemur, Galago), which are used during advertisement or in alarm situations, resemble human infant crying, whining, or a dog barking. The mews of ring-tailed lemurs or ruffed lemurs (Varecia variegata), which govern reunions, resemble the moaning meowing of cats, whereas the melodious low-frequency songs and duets in indris and sportive lemurs (Lepilemur), which are given in a territorial defense context, resemble howling in coyotes or wolves. The high-frequency/ultrasonic, multi-syllabic, and rapid frequency-modulated trills and chirps of mouse lemurs (Microcebus) and the songs of tarsiers (Tarsius, Carlito), which are produced in a reunion or mating context, sound like chirps in mating songs of crickets and grasshoppers. These sounds are barely perceptible or imperceptible to the human ear, but they resemble singing in birds when transferred to the human hearing range. The tonal, high-frequency, and almost constant-frequency whistles characteristic of cohesion or disturbance/alarm contexts in lorises (Perodicticus, Nycticebus, Loris), woolly lemurs (Avahi), tarsiers (Tarsius), and small-bodied lemurs (Cheirogaleus, Microcebus) sound quite similar to whistling sounds in humans. Note, however, that sounding like these other sound sources does not mean that the sound production mechanisms are comparable (also see Fitch 2010).


Fig. 5.2
Infant isolation calls of basal primates. Displayed are the major similarities in acoustic structure within the phylogenetic group of the Lorisiformes
As in monkeys and apes, the high diversity of vocalizations in basal primates provides the potential for recognition even across distance and, consequently, for the gathering of dispersed members of social units at a particular site and a distinct time. A recent study in group-living lemurs pointed to the fact that vocal exchanges indicate the strength in social bonds between group members and allow grooming at a distance through vocal signaling networks (Kulahci et al. 2015). Furthermore, specific differences in acoustic signaling and recognition systems may represent an efficient premating isolation mechanism, contributing to species cohesiveness when living in sympatry (Zimmermann 2016).
5.2.2 Indexical and Prosodic Cues Conveyed in Voice and the Question of Functional Referentiality
Research studies on vocal communication in humans, monkeys, and apes have revealed that indexical and prosodic cues in voice are perceived and recognized (Altenmüller et al. 2013). Indexical cues are specific signatures that are linked to individuality, sex, kin, population, subspecies, or species. When conveyed vocally, these cues provide the substrate for recognizing the respective category without any additional visual cue. In contrast, prosodic or paralinguistic features constitute affective prosody (i.e., patterns of stress and intonation in acoustic expressions). Humans make use of these paralinguistic features in affective prosody not only to express what and how they feel but also to evoke or interpret these feelings in others, as well as to think about their own and others’ feelings as they make decisions. Although humans are unique in the goal-directed usage of prosodic cues in voice for decision making in groups and social manipulation, the acoustic communication of emotions and emotional contagiousness evoked by voice also are an important and potentially universal feature of monkey and ape societies (e.g., for governing group movements and reunions) (Altenmüller et al. 2013). Furthermore, monkeys and apes often vocally express emotions linked to specific individualized contexts, potentially leading to context-specific calls. When these calls are then responded to in a context-specific way without any additional visual cue, they are often termed functionally referential (see Wheeler and Fischer 2015; Snowdon, Chap. 6; Zuberbühler, Chap. 7). To what extent do basal primates share these building blocks in acoustic communication with monkeys and apes?
Current research on basal primates has revealed that these primates also exhibit a rich diversity of vocalizations in which indexical cues are conveyed, as is known for monkeys and apes. Vocal indexical cues are widespread across both groups of basal primates (the strepsirrhines and the tarsiers) and thus are present irrespective of social system and phylogeny. Individual-specific acoustic signatures are described from calls of all major phylogenetic groups of the strepsirrhines, such as the nocturnal, solitary-ranging mouse lemurs (Leliveld et al. 2011) and bushbabies (Kessler et al. 2014), the nocturnal, dispersed pair-living sportive lemurs (Rasoloharijaona et al. 2006), the cohesive pair-living woolly lemurs (Ramanankirahina et al. 2015), the cathemeral group-living crowned lemurs (Eulemur coronatus, Gamba and Giacoma 2007) and red-bellied lemurs (Eulemur rubiventer) (Gamba et al. 2011; Gamba et al. 2012b), the diurnal pair-living indris (Giacoma et al. 2010; Torti et al. 2013), and the group-living silky sifakas (Propithecus candidus) (Patel and Owren 2012) and ring-tailed lemurs (Macedonia 1986; Oda 2002). Individual-specific variation of long-distance calls was also revealed in Philippine tarsiers (Carlito, previously Tarsius, syrichta) (Řeháková-Petrů et al. 2012).
Sex-specific variation in acoustic dimensions also occurs in both groups of the basal primates, such as in sex-specific syllables of duetting songs in the nocturnal Milne Edwards’ sportive lemur (Lepilemur edwardsi) (Rasoloharijaona et al. 2006), the cathemeral crowned lemur (Gamba and Giacoma 2007), the diurnal indri (Giacoma et al. 2010; Torti et al. 2013), and nocturnal tarsiers (Niemitz et al. 1991; Nietsch 1999; Merker et al. 2010). The first evidence for vocal kin signatures and vocal kin recognition in basal primates was recently reported in gray mouse lemurs (Microcebus murinus) (Kessler et al. 2012, 2014).
Group, population, subspecies, and species-specific signatures in vocalizations are present in both groups of the basal primates. They are documented for strepsirrhines, such as the nocturnal, solitary-ranging lesser (e.g., Zimmermann 1990; Ambrose 2003) and larger bushbabies (Clark 1988; Masters 1991); the nocturnal, solitary-ranging lemurs (Hafen et al. 1998; Braune et al. 2008); the nocturnal, dispersed pair-living lemurs (Méndez-Cárdenas et al. 2008); the cathemeral group-living lemurs (Gamba et al. 2012a); the diurnal pair-living lemurs (Gamba et al. 2011) and group-living lemurs (Macedonia and Taylor 1985; Gamba et al. 2012b); and tarsiers (Nietsch 1999; Merker et al. 2009; Burton and Nietsch 2010).
In sum, vocal indexical cues are present in monkeys, apes, and humans (see Snowdon, Chap. 6; Zuberbühler, Chap. 7) but also in basal primates. Since these vocal cues are described from different phylogenetic clades (Zimmermann et al. 2013), such as tree shrews, bats, rodents, pigs, elephants, carnivores, dolphins and whales, vocal indexical cues likely represent universal features across all mammals, providing an important basis for sensory exploration, such as for the acoustic recognition of the respective categories. To what extent these acoustic cues for species, subspecies, population, group, sex, or kin recognition are used in the respective social system of the different phylogenetic groups of basal primates is an interesting but rather unexplored area in bioacoustic research.
Prosodic cues (referring to emotional/motivation states or potentially to particular categories in the external world, such as type of predator) are not only present in monkeys and apes but are also described for strepsirrhines, whereas nothing is known so far for tarsiers. As put forth by Morton (1977) and refined by August and Anderson (1987), motivation-structural rules should govern vocal production and favor the emergence of cross-taxa universals in vocal acoustics based on physiological constraints. Cross-taxa universals, conveying varying degrees of short-term stress or response urgency, were shown in the temporal pattern of different strepsirrhine vocalizations in which higher affect intensity, arousal, or response urgency was expressed by higher calling rates (Zimmermann et al. 2013). Likewise, a first cross-taxa comparison of the most commonly used calls in the vocal repertoire of lesser mouse lemurs of the genus Microcebus (Zimmermann 2016) pointed to cross-taxa universals in the acoustic contour of calls used either in the agonistic, pain, or alarm/disturbance context, whereas major species-specific divergences occurred in the acoustic contour of vocalizations used in the affiliation context (mating, group reunion, mother-infant reunion). These findings provide some support for the refined Morton motivation-structural rules and suggest that natural selection (predation, physiological constraints linked to short-term stress) limits cross-taxa vocal flexibility and favors universals in acoustic structure, whereas sexual and kin selection drives cross-taxa flexibility and divergence specifically.
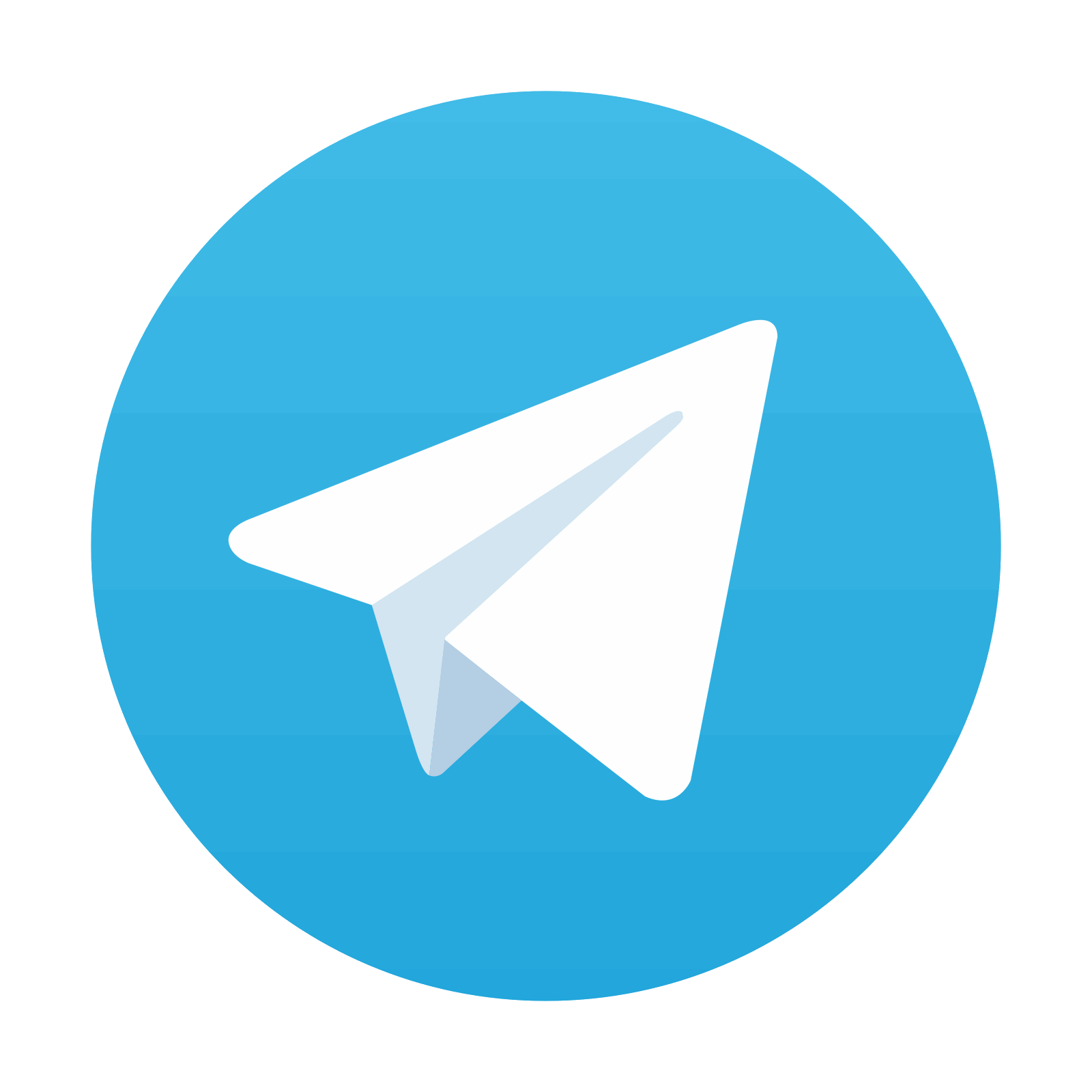
Stay updated, free articles. Join our Telegram channel

Full access? Get Clinical Tree
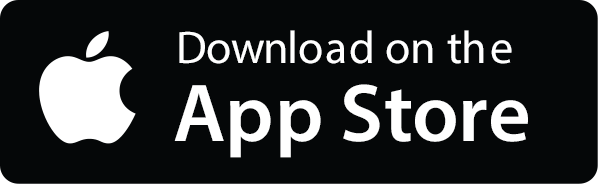
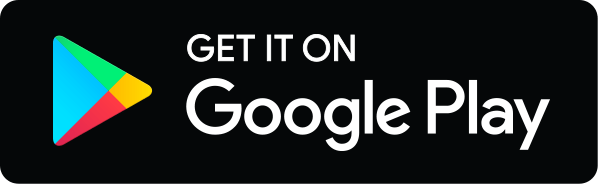