Fig. 4.1
Savanna (left panel), riverine forest (middle panel), and rain forest (right panel) differ prominently in terms of vegetation, humidity, wind, and their impacts on sound propagation, attenuation, and reverberation
4.3.1 Habitat Characteristics
The early Waser and Brown studies were carried out in two African rain forest sites in Uganda and Kenya. Both study sites were in mature forests with an understory of semiwoody plants shaded by a continuous canopy at 30 m with an occasional emergent reaching 50 m in elevation. The rainy and dry seasons occur twice annually in both forests, but seasonality is not pronounced, and there is considerable year-to-year variation.
Riverine forests are vegetationally simple relative to rain forests. In contrast to rain forest sites, the riverine forest canopy is incomplete, with only the occasional tree reaching 30 m in elevation. With more light penetrating the canopy, the understory can become very dense. Measurements were conducted in eastern Kenya where the forest comprises a strip of vegetation a few hundred meters wide that is subject to regular flooding and rainfall is highly seasonal.
Savanna measurements were conducted in central Kenya. The study sites consist of grassland with scattered thickets and small trees consistent with the acacia-commiphora thornbush characteristic of most of eastern and northern Kenya (Lind and Morrison 1974).
4.3.2 Studying Habitat Acoustics: Ambient Noise and Sound Transmission
Studies of habitat acoustics have varied considerably in methodology and, as discussed in Sect. 4.5, methodological variation often limits our ability to generalize results across habitats, seasons, and species. Michelsen (1978) pointed out some of the pitfalls plaguing sound propagation experiments, and many of the considerations discussed by Fischer et al. (2013) with regard to primate sound playback experiments are also relevant to studies of sound attenuation and degradation.
The Brown and Waser experiments attempted to deal with some common issues. For example, ambient-noise samples were designed to systematically sample the background noise generated by biotic and abiotic sources of sound as a function of time of day, season, and location within a habitat type. Sound transmission tests were broadcast through calibrated speakers and rerecorded at multiple distances, with tests replicated along orthogonal tracks both upwind and downwind. The initial inventory of broadcast sounds comprised pure tones, tone pulses, and tone pulse trains transmitted at octave intervals up to 4 kHz, spanning the frequencies and pulse rates observed in primate calls. Pure tone broadcasts were designed to measure attenuation and amplitude fluctuations, while the tone pulse and pulse train broadcasts measured reverberation time and modulation depth as functions of carrier frequency. Subsequently, broadcast stimuli consisted of representative rain forest and savanna monkey calls. Where possible, tests used a balanced design incorporating homologous calls from species living in the different habitats (Waser 1982; Gautier 1988) with all calls broadcast through both appropriate and inappropriate habitats. Call types were included only if it was possible to obtain exemplars recorded close to the vocalizer with very good signal-to-noise ratios and no apparent distortion, thereby ensuring the fidelity of the signal. In each site, ambient-noise measurements and sound transmission broadcasts were conducted at elevations appropriate to the primate inhabitants.
4.3.3 Primate Habitats: How They Differ Acoustically
4.3.3.1 Ambient Noise
Exposure to elevated levels of noise can produce four outcomes: permanent hearing loss, temporary hearing loss, masking of biologically important signals, and physiological and psychological stress (Miller 1974). Ambient noise generated from biotic sources can approach levels sufficient to produce permanent or temporary hearing loss. The noise of some chorusing insects (Young 1990) may exceed 110 dB (A scale), a level sufficient over time to produce hearing loss in most, if not all, species of birds and mammals (Miller 1974; Dooling et al. 2009). Primates presumably distance themselves from sources of dangerously high levels of ambient noise, and under most conditions the chief impediment posed by ambient noise is the masking of biologically important signals. Habitat noise will compromise the capacity of listeners to detect the presence of predators and prey, to hear alarm calls, to warn conspecifics, and to acoustically identify individuals and recognize species. These tasks differ in their complexity and require different signal-to-noise ratios to be executed accurately. Therefore, masking is a potential problem for all species of primates in all habitat types.
Ambient-noise surveys show that noise levels differ as a function of habitat type. Overall, ambient-noise levels are lowest in the savanna and highest in the riverine forest. Sound pressure levels are greatest for low-frequency sounds, decrease to a minimum in the vicinity of 1 kHz, and then increase for higher frequency bands in all three habitats. However, the details of the frequency spectrum for ambient noise differ significantly among habitats (Fig. 4.2).


Fig. 4.2
Ambient noise averaged across time of day in savanna (circles), riverine (squares), and rain forest (triangles) habitats. (Data after Waser and Brown 1986)
Biotic sound sources, wind noise, and wind-induced vegetation motion are the principal sources of ambient noise in all habitats, but their relative contributions differ among habitat types. In both savanna and riverine forest, the interaction of the wind with vegetation is the primary source of noise. Even in the riverine forest there is little shelter from the wind. As the sun heats the landscape, the air temperature near the surface exceeds that at higher elevations, and this temperature difference produces refraction, eddies, turbulence, and a sound shadow near the ground (Wahlberg and Larsen 2017). Hence, as surface temperatures rise in open habitats, wind-induced noise increases and is prominent from midmorning until late afternoon.
In the savanna, the ambient noise is largely governed by the wind, and only at 0600 h are biotic noise sources prominent. Later in the day, biotic sources of sound are overwhelmed by wind-induced noise. In the riverine forest, biotic sound sources, primarily insects, are conspicuous in the midmornings, producing a 4–8 kHz peak. In rain forest, noise levels are consistently high below 100 Hz and between 2–4 kHz. The 2–4 kHz noise levels in the rain forest are due to birds and insects and consistently exceed those in the savanna and riverine forest. Though the rain forest is noisy in the 2–4 kHz band, it is comparatively quiet between 200–1,000 Hz. Unlike the case for savanna and riverine forest, wind noise is rarely prominent in the rain forest, but dripping condensation and rain are significant sources of ambient-noise. Ambient-noise levels change as a function of time of day in the rain forest, as they do in both the savanna and riverine forest, but the change in ambient-noise levels is primarily due to changes in the acoustic behavior of biotic sources and not due to wind.
Sound level recordings indicate that ambient noise should most adversely impact acoustic communication in the riverine forest. To a human listener, however, the sound of wind in the ears makes hearing seem most challenging in the savanna. Sound level recordings do not adequately capture receptive wind noise produced by the interaction between wind and the listener’s head. Receptive wind noise is presumably governed by the shape of the pinna, the distribution of hair around the head and the ears, and other factors influencing turbulence around the head and in the ear canal (Shaw 1974). In fact, measuring background noise in the afternoon is often difficult due to wind-induced microphone noise, raising the possibility that some primates may have evolved specializations to counter this problem.
4.3.3.2 Attenuation
In an idealized anechoic environment, sound waves would be emitted from a point source and radiated in all directions equally. Sound attenuation would be governed by only one factor: spherical spreading. According to the inverse square law, the surface area of a sphere increases geometrically with increments of the radius, so the sound pressure level is reduced by (2r) or approximately 6 dB in all directions for each doubling of the distance. Excess attenuation is the decrement in sound amplitude greater than that expected by spherical spreading. In East African primate habitats, excess attenuation (measured 1 m above the ground) is more prominent in both the savanna and riverine forest habitats than in the rain forest (measured 7–15 m above the ground). The principal source of this difference is elevation above the ground; most rain forest primates are arboreal, while savanna and riverine forest species are often semiterrestrial. In addition, habitat differences in refraction, humidity, thermal gradients, and turbulence strongly influence sound propagation.
In both rain forest sites, attenuation for broadcasts at 200 Hz is less than that expected by the inverse square law (Fig. 4.3). These findings are consistent with the idea of a sound window for long-distance signaling in the arboreal environment (Morton 1975). The sound window, a range of frequencies within which sound attenuation is minimal, is consistently apparent at all source-receiver distances in rain forest habitats (Waser and Waser 1977; Waser and Brown 1984).


Fig. 4.3
Excess attenuation of broadcast tones as a function of frequency averaged across propagation distance in three primate habitats. (Data after Waser and Brown 1986)
In contrast, in both the riverine forest and savanna environments, excess attenuation generally increases with increments in broadcast frequency, and a sound window for long-range signaling is not apparent. Excess attenuation measurements are much more variable in the savanna and riverine forest sites, especially at longer source-receiver distances, than rain forest measurements. This difference is likely due to refraction, wind, and turbulence. Even relatively low wind velocity muted upwind recordings of white noise bursts by 15 dB or more relative to downwind recordings (Brown, unpublished observations).
The increase in excess attenuation as a function of propagation distance is approximately logarithmic in all three habitats (Fig. 4.4). That is, averaged across broadcast frequency, excess attenuation increases by a similar value for each doubling of propagation distance from 12.5 m to 25 m to 50 m. The growth in excess attenuation with propagation distance is modest in the rain forest habitat and steeper for both the savanna and riverine forest environments.


Fig. 4.4
Excess attenuation as a function of propagation distance averaged across broadcast frequency in three different primate habitats. (Data after Waser and Brown 1986)
4.3.3.3 Amplitude Fluctuations
Fluctuations in amplitude within 20-s tone broadcasts are presumably induced by moment-to-moment instabilities in thermal gradients, creating fluctuations in wind speed and wind direction, for example, within bubbles of turbulent air (Wiley 2015). Amplitude fluctuations are generally similar in all three habitats. Tones broadcast over short propagation distances (e.g., 12.5 m) do not fluctuate in amplitude, while at long propagation distances (e.g., 100 m), tones can warble as much as 10–15 dB. Amplitude fluctuations are also influenced by carrier frequency, and tones from 100 to 200 Hz are less susceptible to fluctuations in level. This suggests that, as a rule, signals in the 100 to 200 Hz region are more favorably propagated and more stable in amplitude than signals at other frequencies, especially in the rain forest habitat.
4.3.3.4 Reverberation
Reverberation is the persistence of sound after the emission of the signal is terminated. Reverberation is created when the signal is reflected from various surfaces in the environment: eventually the sound is absorbed and dissipated and the echoes decay to zero amplitude. The RT 60 , a measurement commonly used in architectural acoustics, is the time required for the amplitude of the reflections of the broadcast signal to decay 60 dB. In East African measurements, reverberation in the savanna is low (190 ms) for all pulse frequencies and propagation distances (Fig. 4.5). Discrete echoes are often audible in both forest habitats but are never apparent in the savanna. In both the riverine forest and rain forest sites, reverberation increases as a function of propagation distance up to approximately 600 ms averaged across carrier frequency. Some carrier frequencies are more prone to reverberation than others, and 500-Hz tone pulses in the riverine forest exhibit the longest reverberation times with RT 60 values approaching 750 ms. The reverberation time for pulses broadcast at 125-Hz are short in all three habitats, confirming that sounds with longer wavelengths are relatively resistant to scattering and reflection in all environments.


Fig. 4.5
Reverberation time (RT 60 ) as a function of propagation distance averaged across pulse carrier frequency in three different primate habitats. (Data after Waser and Brown 1986)
4.3.3.5 Modulation Depth
The persistence of sound due to reverberation poses an impediment for the perception of trilled and pulsed utterances. That is, the silent interval between each successive trill or pulse is not silent in a reverberant environment but is overlaid by the echo of the preceding pulse. Modulation depth is then the amplitude difference between the pulse and the interpulse interval. If reverberation is prominent, modulation depth is low; if reverberation is minimal, modulation depth is high. Pulsed signals retain greater modulation depth in savanna broadcasts than in forest broadcasts. Resistance to pulse train degradation in the savanna is most prominent at short propagation distances and for carrier frequencies of 200 Hz or more. In both forest habitats, modulation depth is significantly degraded for all carrier frequencies and propagation distances (Fig. 4.6). Modulation depth measurements are virtually identical for the two forest habitats.


Fig. 4.6
Percent modulation depth: (a) averaged across pulse carrier frequency and pulse repetition rate as a function of propagation distance; (b) averaged across pulse repetition rate and propagation distance as a function of pulse carrier frequency. The percent modulation depth was calculated as the peak-to-trough sound level difference within a pulse train relative to that broadcast. (Data after Waser and Brown 1986)
4.3.3.6 Call Degradation
In general, primate utterances are composed of a variety of notes or frequency elements emitted in a specific sequence or temporal pattern, and not all of these components will be equally susceptible to modification by absorption, reflection, scattering, and refraction. Scattering may disturb the apparent temporal pattern of a call by masking the reception of low-amplitude or silent elements, overlaying them with indirectly transmitted reflections of the previously emitted, loud components. In the case of the baboon wahoo call, for example, the reflection of the initially emitted wa might mask the reception of the subsequently issued hoo, especially in forested environments. As another example, forest-living gray-cheeked mangabey males code individual identity in the number of rapidly repeated pulses in the gobble of their whoop-gobble calls (Waser 1982); reverberation presumably obscures this information for listeners far from the source.
Similarly, attenuation is typically frequency specific. Therefore, at a distance from the vocalizer, the amplitude ratio of different elements is disturbed, changing the apparent emphasis of different frequencies in the utterance. Because scattering and attenuation are largely independent processes, signal degradation may occur independently in the frequency and time domains. That is, the frequency components of a signal may be differentially attenuated or absorbed, while the temporal envelope of the signal is preserved. Alternatively, scattering may disturb the temporal patterning of a signal without the call spectrum being changed by differential attenuation. Hence, metrics of degradation have been developed for both frequency and time domains (Brown and Waser 1988; Brown et al. 1995).
4.3.3.7 Time Domain Distortion Analysis
To characterize the magnitude of distortion in the time domain, the broadcast signal (recorded 1 m from the source in an anechoic chamber) can be cross-correlated with the corresponding waveform recorded at 12.5 m or 100 m. The height of the ordinate of a normalized cross-correlogram would be 1.0 if the two waveforms were identical and would incrementally become smaller as the two waveforms became progressively dissimilar. As an example, average exemplars for the blue monkey boom call at propagation distances of 1, 12.5, and 100 m are shown in Fig. 4.7. The waveform for exemplars recorded at 12.5 m or 100 m does not change much relative to the waveform of the broadcast signal, and the cross-correlations for the boom at 12.5 and 100 m are 0.96 and 0.94, respectively. Hence, the boom is quite resistant to distortion in the time domain.


Fig. 4.7
Mean waveforms of the blue monkey boom call at propagation distances of 1.0 m, 12.5 m, and 100 m. (Data after Brown and Waser 1988)
4.3.3.8 Frequency Domain Distortion Analysis
The frequency spectrum of the broadcast and propagated waveforms is provided by the Fourier transform, and distortion in the frequency domain may be measured by generating a difference spectrum derived by subtracting the propagated frequency spectrum from the broadcast frequency spectrum. If the spectrum of the broadcast signal is identical to that propagated and subsequently recorded in the natural habitat, the resulting difference spectrum would be represented by a horizontal line displaced below the original value by the mean attenuation of the signal as a whole. For example, Fig. 4.8 shows the frequency spectrum for the broadcast blue monkey trill (measured 1 m from the source in an anechoic chamber), the spectrum for the trill measured at a propagation distance of 12.5 m in the rain forest, and the corresponding difference spectrum. This difference spectrum shows both positive and negative values. Negative values indicate that the amplitude of the signal at 12.5 m, relative to the mean for the signal as a whole, exceeds that at 1 m, whereas positive values indicate that the relative amplitude of the signal at 1 m exceeds that at 12.5 m. The difference spectrum shows that the blue monkey trill is distorted in the frequency domain by the acoustics of the rain forest after only 12.5 m of transmission.


Fig. 4.8
Blue monkey trill call spectra at propagation distances of 1 m and 12.5 m shown in comparison with the difference spectrum for the 1 m and 12.5 m signals. (Data after Brown and Waser 1988)
4.4 The Acoustic Adaptation Hypothesis
The acoustic adaptation hypothesis can be tested by analyzing the degradation of calls broadcast in appropriate and inappropriate habitats (Brown et al. 1995). If the acoustic properties of the environment in which the signal is normally produced have acted as a source of selection then, for example, distortion should be lower for calls of rain forest monkeys broadcast in rain forest than for those same calls broadcast in savanna.
Overall the results showed that distortion scores were greater for broadcasts conducted in the savanna relative to those conducted in the rain forest, as shown in Fig. 4.9 where the majority of scores fell below the diagonal line. This effect was particularly strong for the values derived from the frequency domain analysis.


Fig. 4.9
Distortion as a function of habitat type. The results are pooled across species and transmission distance. The frequency-domain analysis data are plotted as solid squares, and the time-domain analysis data are plotted as open circles. Most scores fall below the diagonal line, indicating that distortion was greater for broadcasts conducted in the savanna relative to those conducted in the rain forest, particularly for the frequency-domain analysis. The x– and y-axes have no units because distortion scores are dimensionless integer numbers. Larger values indicate greater distortion that can be ranked in severity within a domain. (Data after Brown et al. 1995)
As shown in Fig. 4.10, many calls of rain forest monkeys (blue monkeys and grey-cheeked mangabeys) exhibited low distortion scores for broadcasts in the appropriate habitat (rain forest) relative to those conducted in the inappropriate habitat (savanna). However, the calls of savanna monkeys (vervet monkeys and baboons) exhibited similar distortion values for broadcasts conducted in both appropriate (savanna) and inappropriate (rain forest) habitats. The forest monkey calls that were most strongly distorted when broadcast in the savanna, the inappropriate habitat, included the blue monkey grunt, pyow, trill, ka-train, chirp, and the mangabey chorused grunt, scream, and loud grunt.


Fig. 4.10
Distortion in “appropriate” and “inappropriate” habitats. The solid squares show the results for the frequency-domain analysis; the results for the time-domain analysis are given by the open circles. The data points positioned below the diagonal line denote cases where distortion in the inappropriate habitat exceeded that in the appropriate habitat. Conversely, data points plotted above the diagonal line indicate the reverse. The x– and y-axes have no units because distortion scores are dimensionless integer numbers. Larger values indicate greater distortion that can be ranked in severity within a domain. (Data after Brown et al. 1995)
These findings are consistent with the idea that the structure of calls emitted by rain forest primates has been modified by natural selection to be relatively resistant to distortion, while in the savanna, with the availability of unobstructed visual signaling, frequent wind noise, and the absence of sound windows, the intensity of selection along this dimension has been relaxed.
The ratio of a call’s distortion scores in appropriate compared to inappropriate habitats would seem to be a reasonable index of acoustic adaptation. Some forest monkey calls, including the blue monkey ka-train, trill, and grunt, indeed had impressively low distortion ratios. But the details raise numerous puzzles. For example, the mangabey whoop-gobble, used in long-distance interactions between groups, is apparently one of the most distorted mangabey calls in both time and frequency domains and shows no obvious resistance to degradation in the appropriate habitat. Time domain distortion of the blue monkey boom call appears to be greater in appropriate than in inappropriate habitat. The blue monkey ka-train, usually a response to avian predators, has the lowest measured distortion ratio of any vocalization in the study, but other forest monkey alarm calls (blue monkey chirps and mangabey staccato barks) show no particular evidence of adaptation to minimize distortion. These interesting results highlight the need for additional studies, more sophisticated analyses, and more refined hypotheses (see Sect. 4.5.1).
4.4.1 The Audible Range of Short- and Long-Distance Calls
The audible range of primate calls is governed by habitat acoustics, signal parameters, and the auditory sensitivity of conspecific recipients. Calculations of audible range, therefore, should include five factors: (1) the spectrum and amplitude of the signal at the source; (2) propagation characteristics of signals broadcast in the natural habitat; (3) degradation and distortion of the signal as a result of habitat acoustics; (4) the spectra and amplitude of ambient noise in the natural habitat; and (5) masked auditory thresholds of conspecific listeners for signals embedded in environmental background noise. Masked auditory thresholds have been measured in blue monkeys and grey-cheeked mangabeys for eleven vocalizations using positive-reinforcement operant-conditioning procedures in a laboratory setting (Brown and Waser 1984). For the sake of comparison, audible distance was also calculated for the utterance hey shouted by twelve undergraduate students. Audible range was calculated for vocalizers and recipients at an elevation of 1.5 m (humans) and 8 m (blue monkeys and mangabeys).
The audible distance for these twelve primate calls ranged from 79 m for the blue monkey chirp to nearly 2 km for several mangabey vocalizations (Fig. 4.11). The loudest hey recorded produced an audible distance of 434 m in the rain forest habitat, while the modal distance for this utterance was just 217 m. These results suggest that the audible distance of some forest monkey calls is nearly an order of magnitude greater than that for shouting humans. Differences in the elevation of the source and receiver are the principal parameters that restrict the audible range of humans relative to forest monkeys. Excess attenuation is inversely associated with elevation. Because humans are not arboreal, their loudest utterances will become inaudible after modest propagation distances. The same factor restricts the audible range in the savanna for baboons and vervet monkeys.


Fig. 4.11
Audible distance estimates (in meters) for eleven monkey calls and the human utterance hey. (Data after Brown 1989)
Primates analyze sound to detect the movements of conspecifics, predators, and prey; to identify by voice the age, gender, and social rank of troop members; and to acoustically recognize both individuals and species. These tasks differ in their perceptual complexity and require different signal-to-noise ratios to be calculated with precision. Call discrimination requires more favorable signal-to-noise levels than detection does; call recognition requires a more favorable signal-to-noise level than discrimination does (Dooling et al. 2009). The audible distance calculation is based on masked thresholds, and it would be interesting to see how the communicative range shrinks as the task shifts from detection to discrimination to recognition.
4.5 Evolutionary Aspects of Primate Vocal Signals
The notion that primate vocal signals evolve within constraints set by the acoustic environment, which motivated early experiments, has since expanded into a variety of more specific questions. Four of these fall under the general rubric of the acoustic adaptation hypothesis.
4.5.1 Have Some Calls Evolved to Maximize Active Space?
Sound propagation experiments focused on primates (e.g., Sugiura et al. 2006; Bezerra et al. 2012) have largely reinforced early generalizations that sound attenuates less at lower frequencies, but if the vocalizer is near the ground, sounds propagate poorly at all frequencies (Wiley 2015). Consistent with these generalizations, primate vocalizations thought to function in territorial defense are significantly lower in frequency than other calls in the repertoire, and species with larger home ranges generally produce calls with a lower dominant frequency (Mitani and Stuht 1998). Most extant primates are arboreal, and arboreal adaptations were central to the evolutionary emergence of primates. Phylogenetic analyses confirm that the presence of vocalizations that exploit the arboreal habitat’s advantage for long-distance acoustic signaling is an ancestral trait (Wich and Nunn 2002). Nevertheless, this trait has been lost by most terrestrial and semiterrestrial primates, perhaps because such species have few opportunities to call (or listen) from elevated sites.
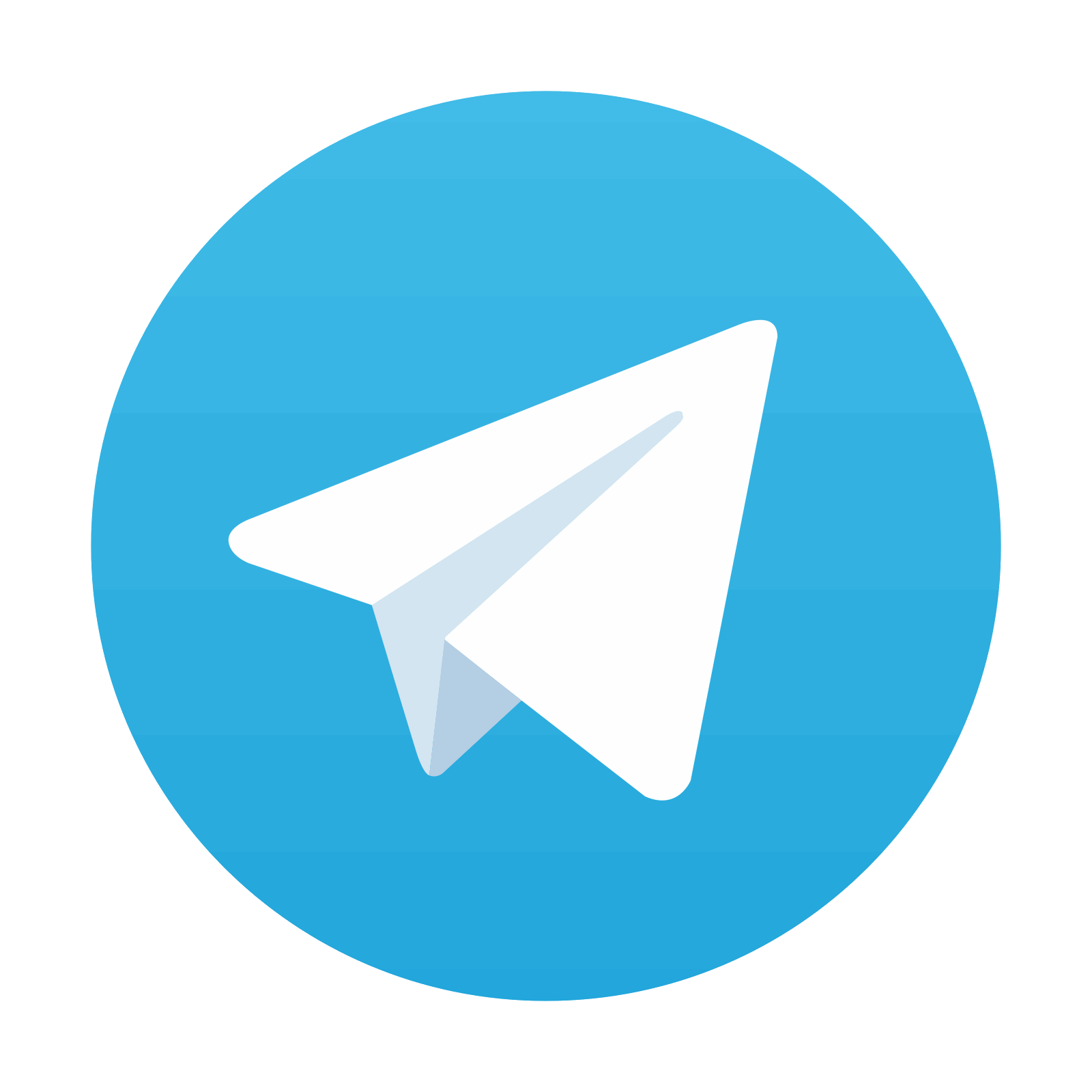
Stay updated, free articles. Join our Telegram channel

Full access? Get Clinical Tree
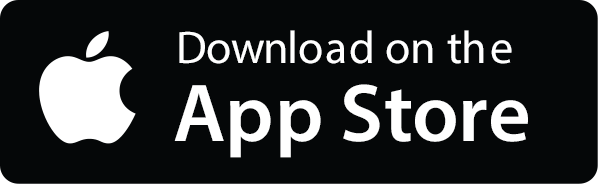
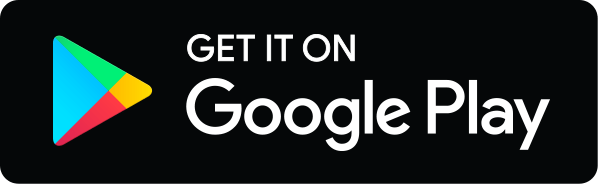