Advances in instrumentation are part of the natural evolution of any surgical discipline. During this process, there are certain key junctures where the state of the art in technology truly augments the surgeon’s ability to manage higher levels of pathology. The present era of endoscopic sinus surgery has been hallmarked by extension of minimally invasive techniques to complex pathologies including advanced inflammatory disease, and pathology involving the orbit, skull base, and brain. Evolution of the armamentarium for endoscopic visualization has been a central feature in this. In this article, key historical elements are reviewed and the technical principles behind these important advances in endoscopic visualization are discussed.
Historical perspective
In 1879 Nitze is credited with the development of a small cystoscope that was subsequently used by Hirschman in 1901 for visualization of the maxillary sinus via an oroantral fistula. It had a small electric bulb and its use was mainly diagnostic. At approximately the same time, Reichert used a 7-mm endoscope in the surgical treatment of oroantral fistulas. Visualization of the maxillary sinus with an antroscope via inferior meatal access was published by Spielberg in 1922. Several years later in 1925, Maltz coined the term “sinuscopy” in promoting use of the endoscope for diagnosis. These early endoscopes were severely limited in depth of field, optical quality, and low illumination from flame or small electric bulbs ( Fig. 1 ). Many years later when the surgical microscope became available, Heermann published on the use of the microscopic for endonasal procedures in 1958.
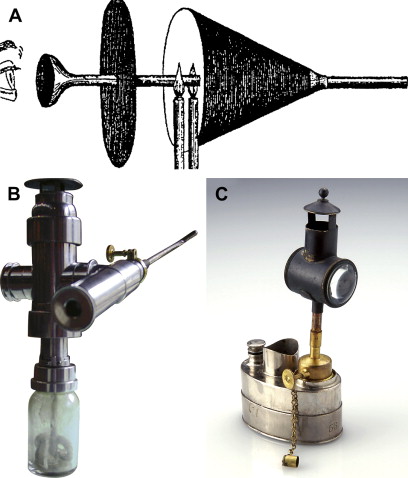
Substantial technologic improvements in fiberoptic design in the early 1950s enabled Harold H. Hopkins of Imperial College London to develop the rod optic endoscope, which became available in the early 1960s. The Hopkins rod endoscopes greatly enhanced light delivery and optical quality. Further improvements came when Karl Storz from Tuttlingen, Germany, built endoscopes with angled views from 0° to 30°, 70°, 90°, and 120°.
These superior endoscopes with fiberoptic cold light and endoscopic cameras provided Messerklinger with the necessary tools to compile the major reference “Endoscopy of the nose,” published in 1978. The Messerklinger technique, which was only mentioned briefly in the book, shifted the focus of surgery from radical procedures of the dependent sinuses to focused treatment of the site of obstruction. Stammberger, Draf, Wigand, and others then adapted use of the endoscope to endonasal procedures, and Kennedy coined the term “functional endoscopic sinus surgery” in 1985.
The historic effect of the introduction of the endoscope in endonasal sinus surgery is best put by Stammberger, “To put it bluntly, the endoscope emerged as the instrument that helped avoid unnecessary and unnecessarily radical surgery.”
Modern advances
Advances in endoscopic technology have encompassed technologic innovations in all parts to the circuit, including light source, the telescope itself, camera head, camera processor, and monitor. Application of endoscopic technique has also proliferated in parallel with the technology to capture and archive photos and streaming video. In this section, the authors highlight key technical aspects of these advancements.
The quality of an endoscope image is determined first by the source data, the surgical field itself. Increased bleeding in the surgical field will oversaturate the image, diminishing quality. This digression is important to consider, however, because the use of a quality circuit may potentially avert maneuvers that confound surgical precision, thereby reducing intraoperative hemorrhage. The advent of the xenon light source (peak wavelengths in the 800–1000 nm range) has markedly augmented visualization simply by increasing the light output. This issue has been studied in automobile headlights, where brightness of xenon sources has been observed to be 3 times greater than that of standard halogen sources, with white rather than yellow tinted light quality. Compared with halogen light, xenon is more durable and efficient, with greater life, less heat production, and less energy consumption.
The Hopkins rod telescope is unique in that it employs true optical media (a series of glass rods and lenses) rather than fiberoptics. The advantage is a clear rather than pixilated image ( Fig. 1 A, B). In addition, autofocusing cameras tend to focus on the pixels rather than the target of interest, further diminishing image quality ( Fig. 1 C). The Hopkins rod telescope also affords greater illumination, color contrast, and depth perception. Current endoscopes have been manufactured at viewing angles ranging from 0° to 120°, with diameters at small as 2 mm. Other advances in the telescope itself have included offset angled endoscopes where the light cable attachment has been modified. Typically the light cable attachment is 180° from the direction to which the lens angle is biased. Manufacturing has allowed for repositioning of this attachment either 0° or 90° from the angle of view, thereby reducing the presence of the light cable in the surgeon’s manual working field ( Fig. 2 A, B ).
Enhancements of digital camera technology have paralleled those for camera technology in common applications. Digital cameras use chips that process color information. Earlier technology employed 1-chip cameras where all color information was processed together, resulting in significant limitations in contrast and balance. Modern 3-chip cameras have spate chips to process each of the 3 primary colors, red, blue, and green. This markedly enhances video quality, but this definition is often lost when video clips are saved and archived, secondary to postprocessing by digital compression algorithms.
Endoscopic surgery has also benefited from enhancements of the viewing monitor. This is of crucial importance because it is common practice for surgeons to use the monitor as the basis of visualization during surgery. Standard definition monitors have used a 4:3 aspect ratio, with pixel density of 640 × 480 in horizontal and vertical dimensions, respectively. In contrast, high-definition monitors use a 16:9 aspect ratio and 1920 × 1080 pixel density. This 7-fold enhancement significantly improves visualization of color and contrast definition, and the augmented aspect ratio improves peripheral visualization within the surgical field.
High-definition cameras also use a scheme of progressive scanning, rather than interlaced scanning, which maximizes image quality on the monitor display. Interlaced scanning is a traditional (analog) technology where each frame is scanned in 2 fields, each containing a series of 262 lines (one frame is the odd lines and the other frame is the even lines). In the United States, video stream is displayed at 60 Hz, or 60 fields per second (NTSC interlacing). Each pair of fields is superimposed through the interlaced scanning process, such that this translates to 30 frames per second. International television uses an interlacing scheme of 312 lines and 50 fields, or 25 frames per second (PAL interlacing). Interlaced scanning suffers from twitter (flickering) of the image, and attempts to compensate for this resulting in some blurring of the image quality and loss of detail. In contrast, progressive scanning reads and displays each line (row of pixels) sequentially, and updates the image at a rate of 60 frames per second. In comparison with 30 interlaced frames per second, this markedly improves image resolution, and lack of image flicker reduces the surgeon’s visual fatigue. Another advantage of progressive scanning is that high-resolution still photographs can be captured for archiving.
The advantage of high-definition video has been demonstrated in an animal model of laparoscopic surgery, where 53 participants (mostly attending and fellow-level surgeons) subjectively graded high-definition camera/monitor systems as superior to standard definition with regard to anatomic orientation, instrument introduction, color, detail, and sharpness. A subset of this group (n = 20) also underwent skills assessment on a laparoscopic training simulator, including standardized tasks of hand-eye coordination, suturing, and knot tying. This analysis revealed significant performance advantage during knot tying with use of a high-definition camera/monitor. This improvement was more pronounced in less experienced operators, underscoring the advantage of high-quality visualization modalities in accelerating the learning curve.
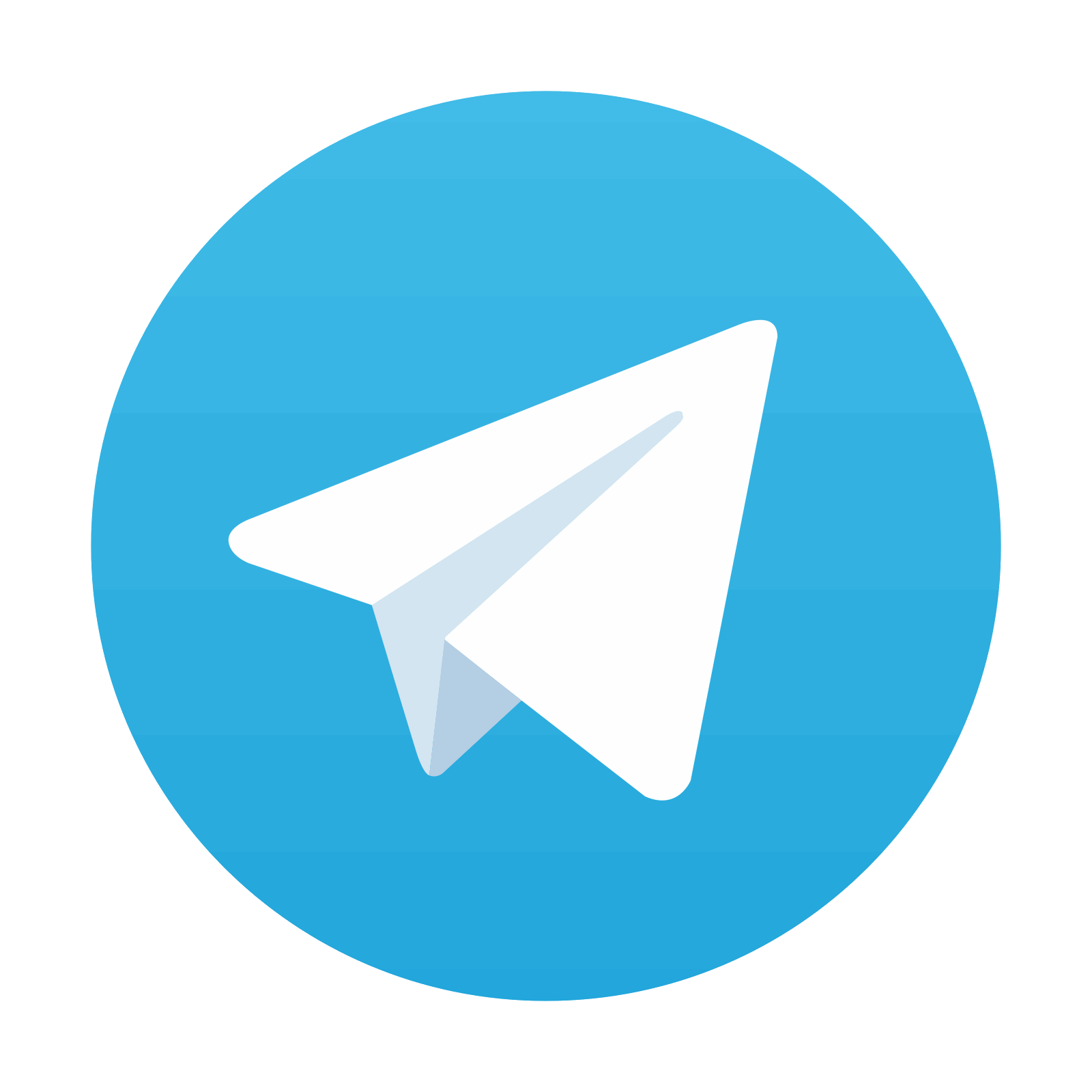
Stay updated, free articles. Join our Telegram channel

Full access? Get Clinical Tree
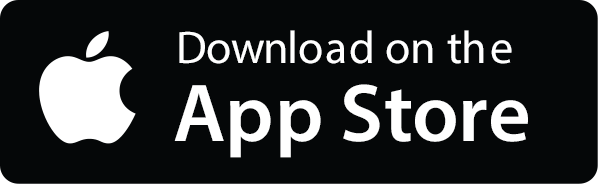
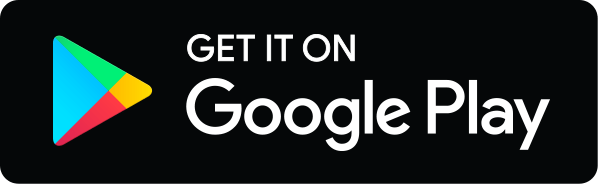