▪ A decrease in density and distribution of photoreceptors
▪ Loss of melanin granules, lipofuscin accumulation, and residual body accumulation in the retinal pigment epithelium (RPE)
▪ Accumulation of basal lamellar deposits, formed by a lipidrich granular material and collagen fibers, between the lamina basalis (plasma membrane) of the RPE cells and inner face of the basal membrane of RPE
▪ Changes in choriocapillaris
also be classified by the discreteness of their boundaries: hard (well defined), soft (not well defined), and confluent (contiguous limits between drusen).
genes functionally related to complement and immune processes, high-density lipoprotein (HDL) cholesterol, and mechanisms involving collagen formation, extracellular matrix production, and angiogenesis considered to be associated with the onset, progression, and bilateral involvement of early, intermediate, and advanced states of AMD (see Table 4.1 and Refs. (27,28,29,30,31,32,33,34) for more details) (35). Several pharmacogenetic studies found that variants in some genes are related to different treatment outcomes (Table 4.2) (36).
Table 4.1 GENETIC LOCI ASSOCIATED TO AMD | ||||||||||||||||||||||||||||||||||
---|---|---|---|---|---|---|---|---|---|---|---|---|---|---|---|---|---|---|---|---|---|---|---|---|---|---|---|---|---|---|---|---|---|---|
|
Anatomically, PR cells have four different regions: the outer segment (OS), the inner segment, the cell body, and the synaptic terminal. The OS of the PR is composed of membranous disks, which have a high concentration of visual pigment. Rhodopsin, the visual pigment of rods, is a G protein-coupled receptor that when stimulated by a photon of light undergoes a conformational change initiating a series of biochemical steps leading to the onset of the electric activity (3). Numerous studies of the human and animal retina show that excessive light exposure leads to photochemical injury causing damage to the outer segment of the PR. Excessive light exposure is considered to be an environmental factor associated with AMD (60), but the magnitude of this risk is hard to evaluate and is controversial. Even under normal light conditions, the PR incurs significant oxidative stress, due to the great energy requirement for visual phototransduction (61). This stimulation increases the oxygen-reactive species, damaging DNA and other macromolecular complexes important for the PR survival (62,63). For a longterm survival of the PR cells, it is important to have a healthy RPE participating in the visual cycle, renewing the PR OSs and producing the interphotoreceptor cell matrix (3).
Table 4.2 PHARMACOGENETICS AND AMD | |||||||||||||||||||||||||||||||||||||||||||||||||
---|---|---|---|---|---|---|---|---|---|---|---|---|---|---|---|---|---|---|---|---|---|---|---|---|---|---|---|---|---|---|---|---|---|---|---|---|---|---|---|---|---|---|---|---|---|---|---|---|---|
|
▪ Regeneration of bleached visual pigments (opsins)
▪ Formation and maintenance of the interphotoreceptor matrix and Bruch’s membrane
▪ Transport of fluids and ions between PRs and choriocapillaris
▪ Phagocytosis
of the PR cells are engulfed by the RPE. In the RPE, the disks fuse with lysosomes, forming phagolysosomes, where the contents are degraded. In young, healthy individuals, most of the disks are fully degraded, and lipofuscin accumulation is minimal, but over time, the self-limited phagocytic and degradative capacity of the RPE cells becomes more and more overloaded. This incompletely degraded membrane material accumulates in the form of lipofuscin in secondary lysosomes or residual bodies (67).
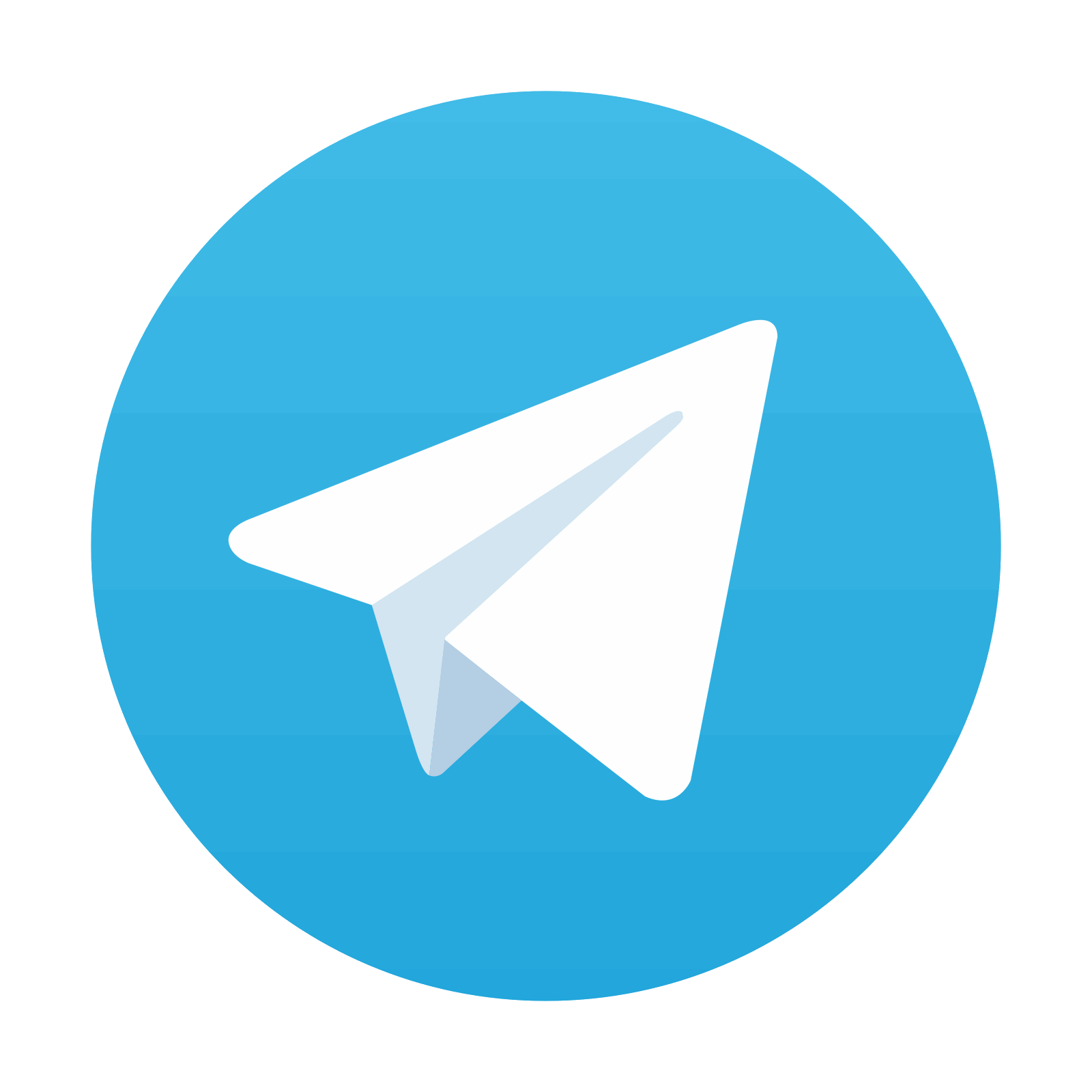
Stay updated, free articles. Join our Telegram channel

Full access? Get Clinical Tree
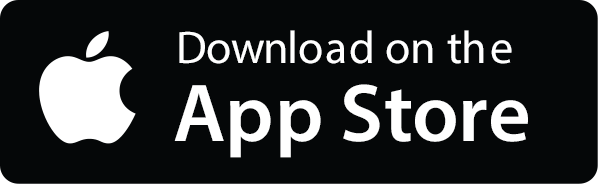
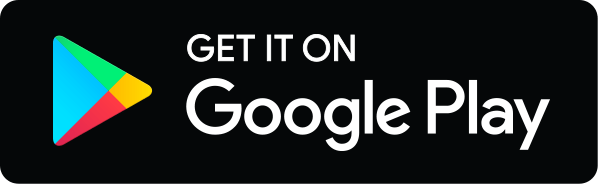
