27
Epiretinal Membrane
Richard C. Lin
William F. Mieler
William J. Wirostko
Epiretinal membranes (ERMs) are proliferations of fibrous tissue along the inner retinal surface. Since their initial description by Iwanoff in 1865 (1), ERMs have been referred to by many names, including surface wrinkling retinopathy (2), macular pucker (3–5), cellophane maculopathy (6, 7), and wrinkling of the internal limiting membrane (ILM) (8). Other names are preretinal macular fibrosis (8, 9), macular ERM, primary retinal folds (10), idiopathic preretinal macular gliosis (11–13), and vitreoretinal interface changes (14). ERMs can be primary (or idiopathic) versus secondary; underlying conditions for secondary ERMs include retinal breaks with or without retinal detachment, blunt or penetrating ocular trauma, inflammation, vitreous hemorrhage, retinal vascular disorders, and ocular surgery (15).
When located within the macula, ERMs can produce visual symptoms, namely decreased visual acuity (VA), metamorphopsia, micropsia, or monocular diplopia (16). If symptomatic enough to bother patients, ERMs can be treated by pars plana vitrectomy (PPV) with membrane peeling. In this chapter, we will discuss the clinical features of ERMs, their pathogenesis, and operative considerations including preoperative evaluation and surgical approaches, along with results and complications of surgery.
Figure 27-1. A: Color fundus photograph OS of a dense opaque epiretinal membrane (ERM) following a rhegmatogenous retinal detachment. B: Fluorescein angiogram (FA) demonstrating late leakage of dye along the temporal border of the macular region from breakdown of the retinal vasculature under the ERM.
CLINICAL FEATURES
Most ERMs are peripheral to the macula and produce no visual symptoms (17). However, visual changes may develop if the ERM occurs in the macula. Symptoms include decreased VA, micropsia, metamorphopsia, and monocular diplopia. These may develop either slowly or rapidly (8, 15–19). Absolute scotomas are rare (8, 20). Mechanisms for visual changes involve ERM opacities overlying the fovea, macular traction and distortion, macular edema, or traction-induced ischemic changes (15–18). Severity of symptoms relates to ERM type, with a thin ERM producing the mildest symptoms. Eyes with thin idiopathic ERMs retain 20/200 vision or better in 95% of cases, and commonly maintain 20/50 VA (8, 19–21). Thin idiopathic ERMs are generally stable, produce no further decrease in VA after diagnosis in 87% of cases, and undergo no change in clinical appearance after 38 months in 83% of cases (11, 19). ERMs associated with proliferative vitreoretinopathy (PVR) are typically thicker and possess an avascular, fibrocellular appearance (Fig. 27-1) (22). Diabetic ERMs are commonly fibrovascular (23). Visual prognosis with these membranes may be limited by associated macular disease. Occasionally, visual symptoms from an ERM may spontaneously resolve after a PVD separates the ERM from the retina (24, 25).
Biomicroscopically, ERMs demonstrate a spectrum of clinical signs. In the mildest cases, the ERM may be just a glinting, shifting light reflex on the retinal surface seen only with red-free light (Fig. 27-2) (14, 16). In more severe cases, the ERM may be opaque and produce additional fundus changes. Superficial retinal striae may form adjacent to the ERM (Fig. 27-3) (12, 14). Retinal capillaries may become dark, dilated, and tortuous from ERM retinal traction. Major retinal vessels may appear straightened and drawn together (6, 14). Typically, retinal dragging, macular heterotopia, and tractional retinal detachment only occur when ERM contraction is severe (Fig. 27-4) (7, 12, 16). Pseudoholes may form (Fig. 27-5) (16, 26), and these represent contractions of perimacular ERM that do not extend over the fovea. Pseudoholes must be distinguished from lamellar and full-thickness holes since treatment for each is different. Pseudoholes contain retinal tissue and vessels in their base, have no retinal edge, display no cuff of subretinal fluid, and show no central hyperfluorescence on fluorescein angiography (FA) (12, 14). It should be remembered that ERMs can occur in eyes with full thickness macular holes (14). Microaneurysms, blot hemorrhages, and yellow hard exudates may develop, with or without retinal edema (8, 12, 16, 20). In cases of severe retinal thickening, one sees shadows of the superficial retinal vessels on the retinal pigment epithelium (RPE) (20). Frequently, FA demonstrates irregular leakage of dye in this region (14). Typical cystoid leakage may not be apparent because of retinal distortion. Retinal cystoid spaces may form as the retinal edema coalesces. One should always consider choroidal neovascularization (CNV) as an etiology for retinal edema and hard exudates in older patients. Cotton wool spots and fluffy white retinal opacities may form. These are felt to be from blockage of axoplasmic flow from retinal traction (27). They usually resolve within several days after ERM removal. Retinal pigmentary changes may occur, namely RPE hypertrophy and atrophy. These are poor prognostic signs and can be from ERM-induced retinal traction or the original event that induced ERM formation (trauma or inflammation). Occasionally, pigment may be seen within the ERM itself (14). This represents ingestion of melanin or hemosiderin by macrophages within the ERM, or the proliferation of RPE cells in the ERM. Intraocular inflammatory cells are usually not seen with the ERM. Their presence suggests an underlying inflammatory etiology (14). A thorough funduscopic examination with peripheral retinal assessment is recommended in all patients with ERM to rule out retinal vascular disease or the presence of a retinal break. FA may be obtained prior to surgery to document retinal changes, to rule out the presence of CNV, and to exclude the possibility of macular ischemia from retinal vascular occlusive disease. Optical coherence tomography (OCT) is also routinely obtained to document the appearance of the ERM, and it is also employed in the decision-making process of deciding whether or not to perform surgical removal of the ERM. The use of OCT will be discussed in greater detail later in this chapter.
Figure 27-2. Color retinal photograph OD demonstrating mild glistening and retinal sheen from a thin epiretinal membrane.
Figure 27-3. Color retinal photograph OS showing tortuous, dragged retinal vessels and retinal striae from an epiretinal membrane.
Figure 27-4. A: Color fundus photograph OD showing a very prominent epiretinal membrane (ERM) with mild pigmentation (possibly consistent with a combined hamartoma of the retina/retinal pigment epithelium). B: Fluorescein angiogram reveals marked dragging of the retinal vessels along with extensive leakage of dye from the retinal vasculature. C: Postoperative fundus photograph of the same eye after pars plana vitrectomy and ERM removal.
Figure 27-5. (A) Color retinal photograph OS displaying a pseudohole from an epiretinal membrane, with (B) accompanying optical coherence tomography (OCT). At the time of pars plana vitrectomy, no full thickness macular hole was detected.
TERMINOLOGY
No standard nomenclature is currently used to describe ERMs, although several systems have been proposed. Gass (14) offered the following classification of ERMs based on clinical appearance:
Grade 0: Translucent membranes unassociated with retinal distortion (cellophane maculopathy)
Grade 1: Membranes causing irregular wrinkling of the inner retina (crinkled cellophane maculopathy)
Grade 2: Opaque membranes obscuring the underlying vessels with prominent retinal distortion (macular pucker)
Joondeph (28) stratified ERMs into four grades depending on their appearance and anatomic severity:
Grade 1: Transparent ERMs characterized by changes in the light reflex without any appreciable distortion of the retinal tissue; the membrane is completely transparent and is in its earliest stage
Grade 2: Translucent ERMs obscuring some of the underlying retinal landmarks with mild distortion of the retinal architecture but no distinct striae
Grade 3: Translucent to opaque ERMs showing marked distortion of the underlying retina, frequently with retinal striae
Grade 4: ERMs exhibiting severe retinal distortion with complications such as tractional elevation or macular holes
PATHOGENESIS
Depending on the age range and race examined, ERMs occur in about 4% to 18% of the adult population and are bilateral in up to 20% of patients (17, 29–35). The stimulus for ERM formation remains poorly understood. In a recent study, no etiology for ERM formation was found in 68% of cases. In the remaining 32%, factors associated with ERM formation included retinal surgery (scleral buckling, laser photocoagulation, cryotherapy, or vitrectomy) (28%), ocular inflammation (2%), ocular trauma (<1%), or retinal vascular disease (<1%) (36). Additional risk factors for ERM are proliferative diabetic retinopathy (PDR), vitreous hemorrhage, and nonretinal ocular surgery such as cataract extraction (2, 4–6, 8, 12, 15.
Numerous cytokines, receptors, and extracellular matrix proteins have been implicated in ERM formation. In ERMs in diabetic eyes, for example, immunohistochemistry has identified insulinlike growth factor receptor/binding proteins, erythropoietin receptors, hypoxia inducible factor, angiopoietin, and vascular endothelial growth factor (39–42). These factors are likely to play a role in the proliferation of endothelial cells and the development of vascular ERMs. PVR membranes have been shown to express inflammatory mediators and growth factors such as interleukins, tumor necrosis factor alpha, urokinase, tissue plasminogen activator, endothelin 1, and platelet-derived growth factor (PDGF) (43–46). Interestingly, PDGF induces migration of RPE cells across the retina in vitro and in vivo (47–51). Finally, in addition to endothelial cells and RPE cells, glial cells are also likely to play a role in ERM formation through the expression of trophic factors, transcription factors, and cell cycle molecules such as NF-kappaB and cyclin D1 (52, 53).
Mechanical stimuli may also play a role in the formation of ERMs. In particular, the process of posterior vitreous detachment (PVD) has been proposed to cause ERM formation by introducing a small amount of trauma or causing discontinuities in the ILM. Several lines of evidence point to an association between PVD and ERM formation. First, the incidence of idiopathic membranes and PVD both increase with age. One study noted a 10-fold increase in ERMs, from 2% to 20%, when two groups of patients, aged 50 and 75 years, respectively, were compared (2). Second, PVDs have been estimated to be present in 80% to 95% of eyes with ERMs (2,6,11,20–22,29,54. Third, Wiznia (19) described 9% of eyes developing an ERM within 7 days of a PVD, and 41% within 18 months, which suggests that a PVD may directly cause ERM formation. Finally, in PVDs associated with retinal breaks, dispersion of RPE cells through the break can contribute to ERM formation.
On the other hand, a PVD is certainly not required for ERM formation. If PVDs are present in 80% to 95% of eyes with ERMs, this leaves 5% to 20% of eyes with ERMs but no PVDs. Moreover, an ERM can develop on the surface of an attached hyaloid membrane (57). These findings, in combination with the low incidence of ERMs superior to the macula (the site where PVDs often first occur), argue against the requirement of PVD for ERM formation (2). Foos (58) suggested that an ERM may actually induce a PVD by disturbing the vitreolaminar interface, and thus PVDs may be both a cause and an effect of ERMs.
Hirokawa et al. (56) studied 250 eyes with idiopathic ERMs and classified them into four categories based on relationship with the vitreous:
Group 1: No PVD
Group 2: Partial PVD but no vitreoretinal adhesion or traction to the area of preretinal macular fibrosis
Group 3: Partial PVD with vitreous traction to the area of preretinal fibrosis
Group 4: Complete PVD
Interestingly, the worst VA was found in eyes in Group 3 as opposed to patients with a complete PVD or no PVD whatsoever. This configuration of vitreous traction to the area of an ERM is now called vitreomacular traction (VMT) syndrome (18). It is readily diagnosed using OCT (as is discussed later in the chapter).
In short, the exact pathogenesis underlying ERM formation is not precisely understood and depends on a combination of chemical and mechanical factors. The stimulus for ERM formation differs in different clinical settings such as PDR or PVR. Whatever the initiating stimulus, ERM formation is likely an immune process of ocular wound healing and extracellular matrix protein remodeling (59, 60).
HISTOPATHOLOGY
ERMs are fibrocellular sheets of collagen with interspersed cells. Their composition most commonly involves collagen, glial cells, RPE cells, and macrophages (6, 37, 47, 61–63). They may vary in thickness and display a spectrum of histologic composition. In one study of 168 ERMs, one of six predominant matrices was present: glial cells (36%), fibrovascular tissue (17%), cortical vitreous (13%), RPE cells (10%), fibroinflammatory tissue (10%), or a combination (14%) (54). All ERMs contain collagen (63). Specific cells commonly found in ERMs include fibrous astrocytes, RPE cells, fibrocytes, and macrophages. Myofibroblasts, hyalocytes, and inflammatory cells occur less frequently (6, 37, 47, 61, 62). ERM histopathology varies with clinical scenario. Idiopathic ERMs frequently contain glial cells (15, 22, 37, 61–69), diabetic ERMs are commonly fibrovascular, and postretinal detachment ERMs typically contain RPE cells (54).
The cellular origin of ERMs remains debated. Previously, it was believed that glial cells proliferated through defects in the ILM and that RPE cells originated from breaks in the retina (22, 70). While this theory explained the frequency of glial cells in idiopathic ERMs and the presence of RPE cells in postretinal detachment ERM, it failed to explain the presence of RPE cells or macrophages within idiopathic ERMs (15, 22). Now, it is suspected that RPE cells can also originate from non-RPE cells that have undergone transformation into RPE cells, from transretinal migration of RPE cells or from developmental rests of epithelial cells on the surface of the retina, which have undergone activation (18, 68). The contribution from each source is unknown. Macrophages are felt to arise from either local tissue or blood. The latter is more likely, as macrophages are uncommon in normal retina (71). Furthermore, macrophages have been induced in ERMs by autologous intravitreal blood injection in an animal model (72). Recently, it has been demonstrated using electron microscopy that the cellular components of ERMs can lie strictly underneath the ILM (i.e., on the retinal side rather than the vitreous side); thus, breaks in the ILM are not required for ERM formation (73).
EVALUATION BY OPTICAL COHERENCE TOMOGRAPHY
OCT provides noninvasive, high-resolution cross-sectional imaging of the macula and is useful in the preoperative evaluation (Fig. 27-6) and postoperative follow-up of patients with ERMs (Fig. 27-7). OCT imaging is very helpful in identifying associated macular edema (Figs. 27-6 and Figs. 27-7) or VMT (Fig. 27-8), which can be difficult to detect by clinical examination or via FA. VMT refers to a configuration in which adhesion between the posterior hyaloid face and the retinal surface leads to traction in an anteroposterior direction, in contrast to the tangential traction exerted on the retinal surface by a contracting ERM. Do et al. (74) specifically examined the impact of OCT imaging on surgical decision making for ERMs. This study discovered that OCT imaging was more sensitive than clinical examination in detecting macular edema and VMT associated with ERMs. Of the 84 eyes in this study, 19 eyes were recommended for surgery based on clinical examination alone, while 33 eyes were recommended for surgery when the OCT findings were used in conjunction with clinical examination. Gallemore et al. (75) also found OCT to be much more sensitive than clinical examination in detecting VMT in eyes with a variety of conditions including ERMs.
ERMs have also been examined using spectral domain OCT, which provides faster and higher resolution imaging than time domain OCT. Again, spectral domain OCT imaging is useful in identifying macular edema, VMT, and photoreceptor defects that are not apparent on clinical examination (76). In short, OCT imaging is useful in determining the amount of adherence between an ERM and the retina, the amount of associated retinal edema, and the configuration of the ERM (77). This information is extremely helpful to the retinal surgeon both in making the decision as to whether or not to perform surgery, and once a decision is made, in guiding the surgery itself.
Figure 27-6. (A) Color photograph OS showing prominent epiretinal membrane (ERM), (B) fluorescein angiogram reveals moderate leakage, and (C) optical coherence tomography documents the ERM with a distinct edge, along with underlying macular edema.
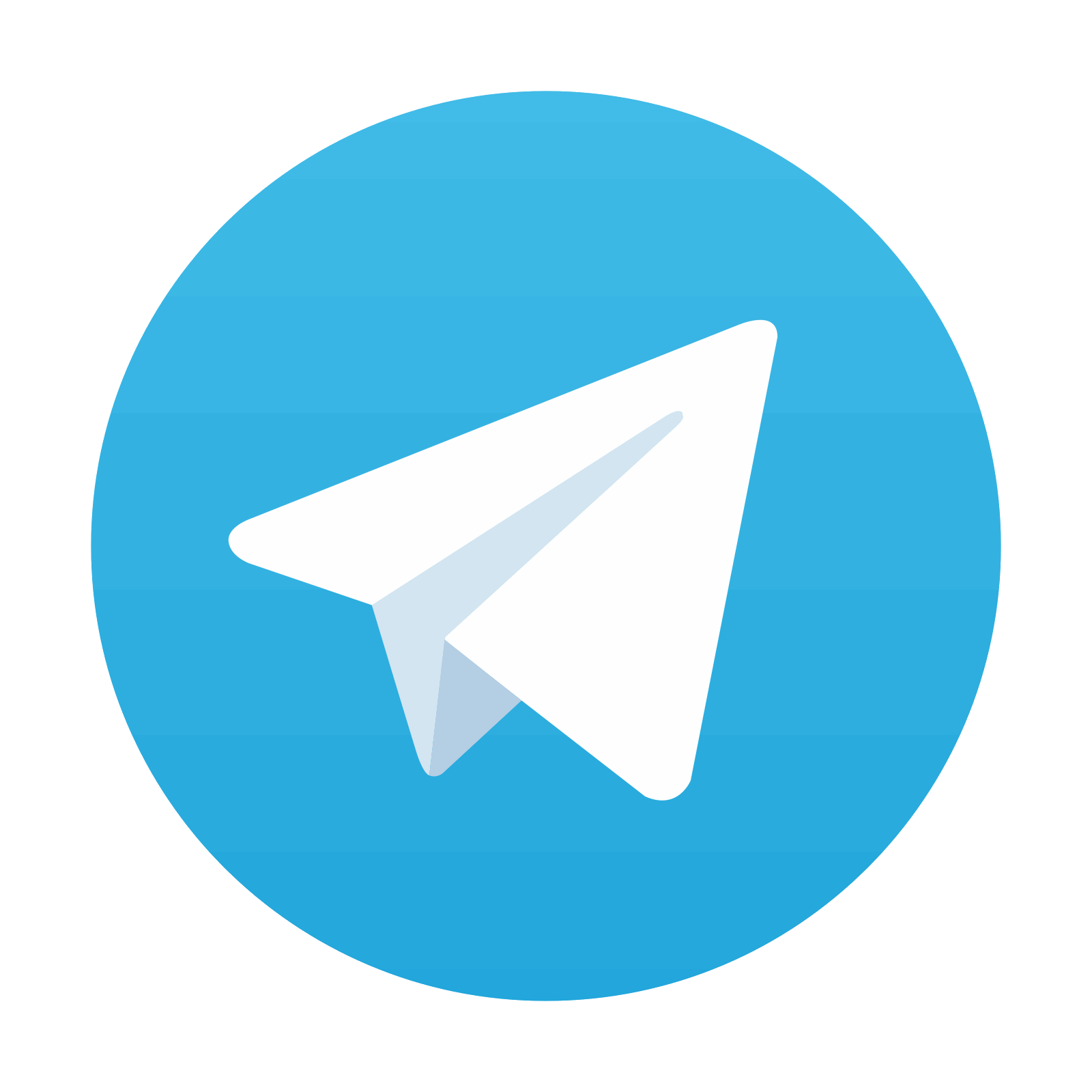
Stay updated, free articles. Join our Telegram channel

Full access? Get Clinical Tree
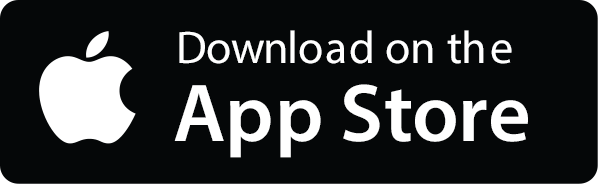
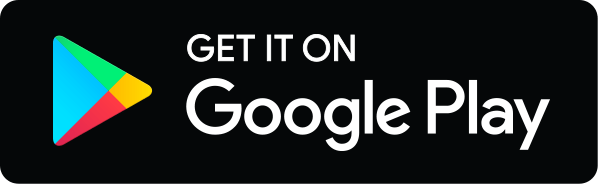