Clinical background
Clinical manifestations of dry age-related macular degeneration (AMD) include drusen, retinal pigment epithelium (RPE) hyperplasia, RPE depigmentation, and geographic atrophy (GA) ( Box 68.1 ). The prevalence of early AMD is 18% in the population aged 65–74 years and 30% in those older than 74 years. GA is the advanced atrophic form of dry AMD. Approximately 3.5% of people aged 75 and older have GA, and its prevalence is greater than 20% among persons aged 90 and older. In the 70s and 80s, choroidal new vessels (CNVs) are approximately twice as common as GA, but GA is more common in the oldest group.
- •
Drusen
- •
Retinal pigment epithelium (RPE) hyperplasia
- •
RPE depigmentation
- •
Geographic atrophy
In many patients, small areas of GA develop near the fovea, often in areas of resorbed drusen or mottled hypo- and hyperpigmentation ( Figure 68.1 ). Over time these areas enlarge, creating multifocal GA surrounding the fovea. The areas may then coalesce, first into a horseshoe-shaped area of atrophy and later into a ring of atrophy, still sparing the foveal center. Finally, the fovea becomes atrophic, and the patient must use eccentric vision for all visual tasks. When the fovea is surrounded by atrophy, the patient may be able to read single small letters on a visual acuity chart but may have great difficulty reading because the surrounding scotomata block off parts of words and sentences. Similarly, these patients may have difficulty recognizing faces, because parts of the face are obscured by the scotomata. In addition, these small spared areas pose a great challenge for low-vision rehabilitation, in that too much magnification will put more of the word in the blind area and may actually make reading more difficult. Patients often use two areas for fixation, a central one for small print and an eccentric one to see the larger picture. This pattern of disease progression can lead one to underestimate the severity of visual impairment associated with GA if one measures central visual acuity solely.

GA is an important cause of moderate and severe visual loss among AMD patients. In a large natural history study, 40% of the patients with GA and vision of 20/50 or better worsened by 3 or more lines on an Early Treatment of Diabetic Retinopathy visual acuity chart over a 2-year follow-up period. A total of 27% of the patients with this good baseline visual acuity worsened to 20/200 or less by 4 years of follow-up. The degree of impairment in dim environments at baseline is predictive of subsequent visual acuity loss. Thus, there is very significant decline in visual acuity during a 2-year period among patients with GA.
The appearance and rate of enlargement of atrophy are very symmetric between eyes in patients with bilateral GA, who constitute the majority of GA patients. The enlargement rate appears to be characteristic of the individual, and this between-subjects factor is more significant than is the size of the GA in estimating the subsequent rate of GA enlargement.
Choroidal neovascularization is relatively uncommon in patients with bilateral GA without CNVs in either eye at baseline. In contrast, patients with CNVs in one eye and GA without CNVs in the fellow eye have a much higher rate of developing CNVs in the eye with GA. It is not yet understood why some patients are more likely to develop CNVs, and others are more likely to develop GA.
The differential diagnosis of dry AMD includes Stargardt disease, central areolar choroidal atrophy, Doyne honeycomb dystrophy, drug toxicity, Best disease, adult vitelliform dystrophy, and some forms of retinitis pigmentosa ( Box 68.2 ). Membranoproliferative glomerulonephritis type II (MPGN II) can be associated with drusen, GA, and CNVs.
- •
Stargardt disease
- •
Central areolar choroidal atrophy
- •
Doyne honeycomb dystrophy
- •
Drug toxicity
- •
Best disease
- •
Adult vitelliform dystrophy
- •
Membranoproliferative glomerulonephritis type II
- •
Some forms of retinitis pigmentosa
Pathology
The abnormal extracellular matrix (ECM) of AMD eyes includes basal laminar deposit, basal linear deposit, and their clinically evident manifestation, soft drusen. The RPE deposits cytoplasmic material into Bruch’s membrane throughout life, possibly to eliminate cytoplasmic debris or as a response to chronic inflammation (see Pathophysiology , below). Histologically, AMD eyes exhibit abnormal extracellular material in two locations: (1) between the RPE plasmalemma and the RPE basement membrane (basal laminar deposit); and (2) external to the RPE basement membrane within the collagenous layers of Bruch’s membrane (basal linear deposit). Although basal laminar deposit persists in areas of GA, basal linear deposit disappears, which is consistent with the notion that basal linear deposit arises mostly from the RPE–photoreceptor complex. Basal linear deposit may be more specific to AMD than basal laminar deposit. Soft drusen can represent focal accentuations of basal linear deposit in the presence or absence of diffuse basal linear deposit-associated thickening of the inner aspects of Bruch’s membrane. Soft drusen can also represent a localized accumulation of basal laminar deposit in an eye with diffuse basal laminar deposit.
Drusen represent the earliest clinical finding in AMD ( Box 68.3 ). Drusen composition and origin have been analyzed extensively. Small (i.e., 63-µm-diameter) drusen generally do not signify the presence of AMD. Excessive numbers of small hard drusen, however, can predispose to RPE atrophy at a relatively young age. Soft drusen are usually pale yellow and large with poorly demarcated boundaries. Many different molecules have been identified in drusen, most of which seem to be the product of oxidative and inflammatory processes ( Table 68.1 ). Many of the molecular constituents of drusen are synthesized by RPE, neural retina, or choroidal cells, but some are derived from extraocular sources.
- •
Accumulation of abnormal extracellular material (basal laminar and basal linear deposit)
- •
Geographic atrophy (loss of photoreceptors and subjacent retinal pigment epithelium and choriocapillaris)
- •
Choroidal neovascularization
α 1 -Antichymotrypsin |
α 1 -Antitrypsin |
Alzheimer’s amyloid β peptide |
Advanced glycation end products |
Amyloid P component |
Apolipoprotein B and E |
Carbohydrate moieties recognized by wheatgerm agglutinin, Limax flavus agglutinin, concanavalin A, Arachea hypogea agglutinin, and Ricinis communis agglutinin |
Cholesterol esters |
Clusterin |
Complement factors (C1q, C3c, C4, C5, C5b-9 complex) |
Cluster differentiation antigen |
Complement receptor 1 |
Factor X |
Heparan sulfate proteoglycan |
Human leukocyte antigen-DR |
Immunoglobulin light chains |
Major histocompatibility antigen class II |
Membrane cofactor protein |
Peroxidized lipids (derived from long-chain polyunsaturated fatty acids, i.e., linolenic acid and docosahexanoic acid, which are normally found in photoreceptor outer segments) |
Phospholipids and neutral lipids |
Tissue inhibitor of matrix metalloproteinases-3 |
Transthyretin (major carrier of vitamin A in the blood) |
Ubiquitin |
Vitronectin |
Areas of GA have a loss of RPE cells as well as overlying photoreceptors and subjacent choriocapillaris atrophy. Choriocapillaris may be lost as a result of RPE loss, and assessment of the region immediately outside the area of GA indicates that the RPE cells are lost first. In many patients with GA, there is increased fundus autofluorescence in the area surrounding the GA, termed the junctional zone. This autofluorescence is a product of RPE cells laden with lipofuscin. Lipofuscin, particularly the N -retinylidene- N -retinylethanolamine component (A2E: see Pathophysiology , below), is harmful to RPE cells and may be partly responsible for GA progression. Using a scanning laser ophthalmoscope (such as the Heidelberg retinal analyzer, HRA) with argon blue light and a barrier filter and with image averaging techniques, one can obtain a high-resolution fundus autofluorescence image ( Figure 68.1 ). Areas that are dark have lost autofluorescence because there is RPE atrophy or attenuation. Areas that are bright have increased lipofuscin deposition. The pattern of increased autofluorescence may be useful in predicting the rate of enlargement of areas of GA.
Etiology
The prevalence of both CNVs and GA is much higher in white populations as compared with black populations. Smoking increases the risk of developing GA and may also increase its rate of progression. The presence of drusen measuring 250 µm or greater and pigmentary abnormalities are risk factors for the development of GA. The way that inflammatory and immunologic factors relate to the development of AMD is not yet clear, but is an active area of investigation.
Pathophysiology
Aging–AMD overlap
Some of the biochemical and histopathological features of AMD seem to occur as a normal part of aging (e.g., lipofuscin accumulation in RPE cells and oxidative damage). Up to 65% of the proteins identified in drusen are present in drusen derived from AMD as well as healthy age-matched donors. Approximately 33% of the drusen-derived proteins from AMD donors, however, are not observed in healthy donor drusen. Thus, despite the fact there is some degree of continuity between aging changes in the photoreceptor–RPE–Bruch’s membrane–choriocapillaris complex and aging changes associated with AMD, aging and AMD seem to be distinct conditions ( Box 68.4 ).
- •
AMD is associated with chronic oxidative damage to the outer retina, retinal pigment epithelium, Bruch’s membrane, and choriocapillaris, and impaired function of antioxidant systems
- •
AMD is associated with chronic inflammation
- •
Increased risk of drusen, geographic atrophy, and choroidal new vessels is associated with mutations in components of the complement pathway, which is part of the innate immune system
- •
Oxidative damage can activate the complement pathway
- •
AMD risk-enhancing mutations not directly involving the complement pathway are also linked to inflammation and/or oxidative damage
It is hypothesized that the photoreceptor–RPE–Bruch’s membrane–choriocapillaris complex is a site of chronic oxidative damage, which is most pronounced in the macula ( Figure 68.2 ). This damage incites inflammation, mediated via complement activation, at the level of RPE–Bruch’s membrane–choriocapillaris. Patients with mutations in components of the complement system are less able to modulate the inflammatory response, resulting in excessive cellular damage and accumulation of extracellular debris. These changes, which involve modification of the ECM, cause additional inflammation and cell damage. This chronic inflammatory response involves cellular components of the immune system as well as the classical and alternate pathways of the complement system. Accumulation of abnormal extracellular material (including membranous debris, oxidized molecules, ECM molecules, and components of the complement system) is thus a sign of chronic inflammatory damage, is manifest in part as drusen and pigmentary abnormalities, and fosters the development of the late sequelae of AMD in susceptible individuals, i.e., GA and/or CNVs. Many treatments for AMD under investigation are based on concepts related to this hypothesis of pathogenesis. Evidence regarding this hypothesis is considered below.

Lipofuscin increases the risk of oxidative damage to RPE cells and possibly to photoreceptors and choroidal capillaries
Lipofuscin comprises a group of autofluorescent lipid–protein aggregates present in nonneuronal and neuronal tissues. Undegradable products of photoreceptor outer-segment metabolism are the major source of RPE lipofuscin. Lipofuscin accumulates in RPE cells as they age, increases the risk of oxidative damage to RPE cells, reduces RPE phagocytic capacity, and can cause RPE death.
The reaction product of ethanolamine and two retinaldehyde molecules, A2E, is the major photosensitizing chromophore in lipofuscin that causes reactive oxygen species (ROS) production. A2E interferes with lysosomal enzyme activity, reduces lysosomal protein and glycosaminoglycan degradation, and inhibits RPE phagolysosomal degradation of photoreceptor phospholipid. RPE cells with excessive A2E exhibit membrane blebbing and extrusion of cytoplasmic material into Bruch’s membrane. Excessive RPE lipofuscin (and A2) accumulation may play a critical role in the pathogenesis of GA.
AMD is associated with oxidative damage
Aging is associated with increased oxidative damage and impaired function of antioxidant systems (see Zarbin for references). RPE susceptibility to oxidative damage increases with aging. Epidemiological studies, AMD histochemistry, and drusen biochemistry indicate that oxidative reactions play a central role in AMD pathophysiology. Each of these areas will be considered briefly.
Epidemiology
Age, smoking, and race are associated with at least a twofold increased risk of AMD. The effect of age on risk may indicate that oxidative damage must be gradual and cumulative for AMD to develop. Also, it may be a sign that mitochondrial DNA damage plays a role in AMD pathogenesis (please see below). Smoking depresses antioxidants (e.g., decreases plasma vitamin C and carotenoids), induces hypoxia and ROS, and alters choroidal blood flow.
White individuals have a relatively higher risk of large drusen, pigmentary abnormalities, and exudative AMD complications compared with blacks. Differences in melanin content may underlie, in part, the racial differences in the risk of advanced AMD. Melanin reduces lipofuscin accumulation in RPE cells, possibly by interacting with transition metals and scavenging radicals to function as an antioxidant. However, the role of ethnicity may reflect the importance of other genetic differences among races. Different ethnic groups may have different genetic mutations underlying the risk of developing AMD. This notion is consistent with the different clinical manifestations of AMD in different ethnic groups. In contrast to whites, for example, soft drusen are only a moderate risk indicator for developing CNVs among Japanese patients, and the 5-year risk of developing CNVs in the second eye is relatively low among Japanese patients.
The Age-Related Eye Disease Study (AREDS) demonstrated that, among patients with extensive intermediate drusen, at least one large druse, noncentral GA in one or both eyes, advanced AMD in one eye, or vision loss in one eye due to AMD, supplementation with ascorbic acid, vitamin E, beta-carotene, zinc oxide, and cupric oxide reduced the risk of developing advanced AMD from 28% to 20% and the rate of at least moderate vision loss from 29% to 23%. The AREDS data may mean that oxidative damage plays a role in the progression of AMD in its clinically evident intermediate and late stages and that disease progression can be altered with antioxidant supplementation. However, zinc also affects the complement system (e.g., inhibits C3 convertase activity), and C3a des Arg (a cleavage product of C3a that reflects complement activation) levels are higher in patients with AMD versus controls.
AMD histochemistry
Using biomarkers of oxidative damage in postmortem eyes from AMD patients, Shen and coworkers found that many eyes with advanced GA showed evidence of widespread oxidative retinal damage, primarily in the inner and outer nuclear layers. The authors posited that a subpopulation of patients with GA have a major deficiency in their oxidative defense system.
Iron is an essential element for enzymes involved in the phototransduction cascade, in outer-segment disc membrane synthesis, and in the conversion of all- trans -retinyl ester to 11- cis -retinol in the RPE (see He et al for references). Free Fe 2+ catalyzes the conversion of hydrogen peroxide to hydroxyl radical, which causes oxidative damage (e.g., lipid peroxidation, DNA strand breaks). Iron accumulation in the RPE and Bruch’s membrane is greater in AMD eyes than in controls, including cases with early AMD, GA, and/or CNVs. Some of this iron is chelatable. One patient with GA also had iron accumulation in the photoreceptors and internal limiting membrane as well as increased ferritin (which sequesters intracellular iron) and ferroportin (an iron export protein that transports unutilized/unstored intracellular iron). Increased intracellular iron causes oxidative photoreceptor damage. Although iron overload is a feature of AMD pathobiology, it is not clear that iron overload is a cause of AMD.
Drusen biochemistry
Advanced glycation end products occur in soft drusen, in basal laminar and basal linear deposits, and in the cytoplasm of RPE cells associated with CNVs. Advanced glycation end products induce increased expression of cytokines known to occur in CNVs. Carboxymethyl lysine, a product of lipoprotein peroxidation or sequential oxidation and glycation, is present in drusen and CNVs. One study of drusen protein composition reported oxidative protein modifications in tissue inhibitor of matrix metalloproteinases-3 and vitronectin. Also, carboxyethyl pyrrole (CEP) protein adducts, which are uniquely generated from the oxidation of docosahexaenoate-containing lipids, were present and were much more abundant in drusen from AMD versus age-matched control donors. (Docosahexaenoic acid is abundant in the outer retina, where it is readily susceptible to oxidation. ) Gu and coworkers found that the mean level of anti-CEP immunoreactivity in AMD human plasma was 1.5-fold higher than in age-matched controls. Sera from AMD patients demonstrated mean titers of anti-CEP autoantibody 2.3-fold higher than controls. In addition to being consistent with the notions that AMD is associated with oxidative damage and that CEP immunoreactivity and autoantibody titer may predict AMD susceptibility, these results indicate that the immune system may play a role in AMD pathogenesis.
AMD is associated with chronic inflammation
Anatomic studies provided initial evidence for the role of inflammation in the early and late stages of AMD (see Zarbin for references). In addition to membranous debris that is probably derived from RPE cells, drusen contain complement components C3 and C5, components of the membrane attack complex (C5b-9), complement factor H (CFH), and C-reactive protein (CRP) ( Table 68.1 ). Bioactive fragments of C3 (C3a) and C5 (C5a) are present in drusen and induce vascular endothelial growth factor expression in RPE cells. These findings provide a mechanistic explanation for the fact that confluent soft drusen are a risk factor for CNVs in AMD eyes. In fact, CNVs cannot be induced by laser photocoagulation in C3-deficient mice. Thus, the presence of proinflammatory molecules in drusen creates a stimulus for chronic inflammation in the RPE–Bruch’s membrane–choriocapillaris complex that can result in some features of late AMD.
Drusen, GA, and CNVs are associated with mutations in components of the complement pathway, which is part of the innate immune system
Three enzyme cascades comprise the complement system ( Figure 68.3 ): the classical pathway (activated by antigen–antibody complexes and surface-bound CRP), the alternative pathway (activated by surface-bound C3b, microbial pathogens, or cellular debris), and the lectin pathway (activated by mannose, a typical component of microbial cell walls, and oxidative stress).

Mutations in the CFH gene ( CFH ), as well as in the closely related genes CFHR1 , CFHR2 , CFHR3 , CFHR4 , and CFHR5 , are strongly associated with both increased and decreased risk for AMD. The CFH Y402H variant (in which tyrosine is replaced with histidine at amino acid 402) is located within a binding site for CRP and is associated with increased risk for drusen, GA, and CNVs. Individuals homozygous for the CFH Y402H variant have a 48% risk of developing GA or CNVs by age 95 years versus a risk of 22% in noncarriers. Nonetheless, one study reported that no single polymorphism (including Y402H) could account for the contribution of the CFH locus to disease susceptibility. Altered interactions of CRP and CFH may lead to changes in C3b activation. Homozygotes with the 402HH genotype have a 2.5-fold increase in CRP in the RPE and choroid compared with 402YY controls, independent of AMD disease status. CFH and FHL-1 with the Y402H mutation display reduced binding to CRP, heparin, and RPE cells, which can cause inefficient complement regulation at the cell surface, particularly when CRP is recruited to sites of injury.
Mutations in factor B (BF) and complement component 2 (C2) alter the risk of AMD. Both risk and protective BF and C2 haplotypes exist. These effects may be independent of CFH mutations. C2 is involved in C3 cleavage via the classical pathway, and BF is involved in C3 cleavage via the alternative pathway ( Figure 68.3 ).
A single nucleotide polymorphism in the C3 gene (R80G) was strongly associated with AMD in both an English and a Scottish group of patients. The estimated population-attributable risk was 22%.
Oxidative damage can activate the complement system
Cultured immortalized RPE cells that have accumulated A2E and are irradiated to induce A2E photo-oxidation form C3 split products in overlying serum. Thus, products of the photo-oxidation of bis-retinoid lipofuscin pigments in RPE cells may serve as a trigger for the complement system. Given the relative abundance of lipofuscin in the submacular RPE, this trigger could predispose the macula to chronic inflammation and AMD. These experiments link RPE lipofuscin, oxidative damage, drusen, and inflammation, all of which have been implicated in AMD pathogenesis.
Oxidative stress reduces the ability of interferon-γ to increase CFH expression in RPE cells. Interferon-γ-induced increase in CFH is mediated by transcriptional activation by STAT1, and its suppression by oxidative stress is mediated by acetylation of FOXO3, which enhances FOXO3 binding to the CFH promoter and inhibits STAT1 interaction with the CFH promoter.
Hollyfield and coworkers described an animal model that links oxidative damage and complement activation to AMD. Mice were immunized with mouse serum albumin adducted with CEP, a unique oxidation fragment of docosahexaenoic acid that is present in drusen of AMD eyes. Immunized mice developed antibodies to the hapten, fixed C3 in Bruch’s membrane, accumulated drusen, and developed lesions resembling GA. Choroidal neovascularization was not observed. An intact immune system is required for these events, which may mean that both the adaptive and innate components of the immune system are required for the development of GA.
Among patients with defective complement regulation and MPGN II, drusen tend to occur in the macular region (as do GA and CNVs). One may speculate that oxidative damage is more pronounced in the macular region, and, as a result, chronic inflammatory damage is more concentrated in the macula.
AMD risk-enhancing mutations not directly involving the complement pathway are also linked to inflammation and/or oxidative damage ( Table 68.2 )
An AMD susceptibility locus on chromosome 10 (10q26) seems to play a major role in AMD pathogenesis. The protein encoded by the hypothetical gene LOC387715 (with an alanine-serine polymorphism as the likely disease-causing variant) in 10q26 may be involved in AMD pathogenesis and is flanked by PLEKHA1 and high-temperature requirement factor A1 ( HTRA1 ). Although two groups found that mutations in HTRA1 are associated with increased risk of AMD ( Table 68.2 ), another group found that this gene was only indirectly associated with AMD. Mutations in CFH , BF , C2 , and HTRA1 seem to confer risk in an independent, log-additive fashion.
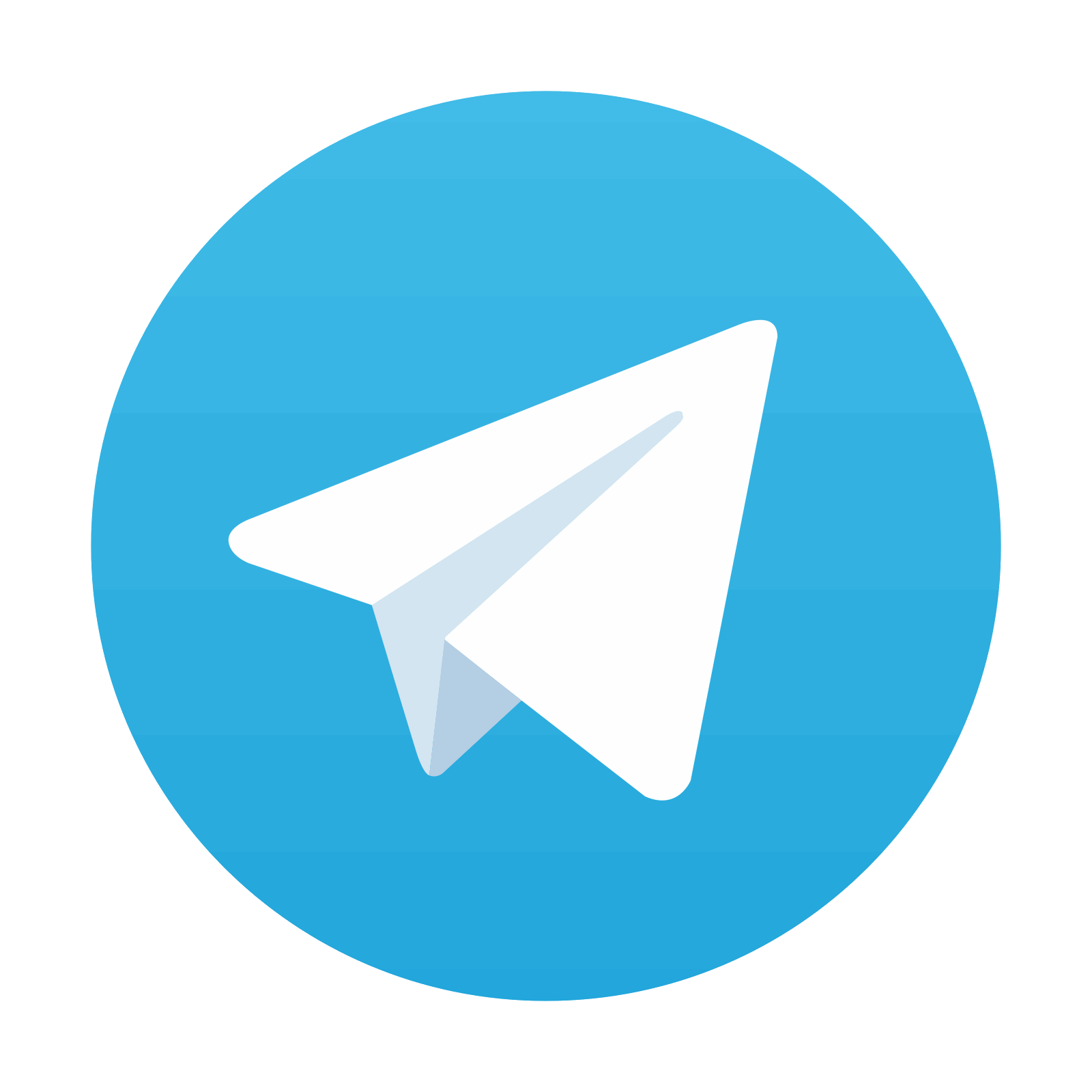
Stay updated, free articles. Join our Telegram channel

Full access? Get Clinical Tree
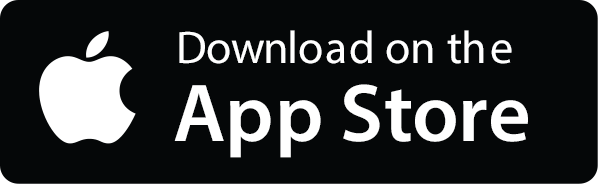
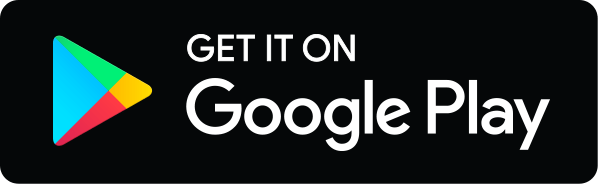
