30 Diagnosis, Evaluation, and Treatment of Facial Nerve Disorders
Facial nerve disorders can be emotionally devastating to patients, and they can have both social and functional effects on daily living. The diagnosis, evaluation, and treatment of these disorders is a complex process that requires a thorough understanding of pertinent anatomy, pathophysiology, testing modalities, and treatment options. This chapter provides an overview of the clinical management of facial nerve palsies with an emphasis on acute facial nerve disorders and general principals of facial nerve surgery.
Anatomy
Motor input to the face begins in the primary somatomotor cortex located in the precentral gyrus (Brodmann areas 4, 6, and 8). The signal then courses through the corticobulbar tract, the internal capsule, the basal pons via the pyramidal tracts, and most of the fibers ultimately decussate to the contralateral facial nucleus. Some fibers remain on the original side and innervate the ipsilateral facial nucleus. The inferior (dorsal) facial motor nucleus innervates the lower musculature. Forehead sparing is seen with central lesions because the forehead receives motor input from the superior (ventral) facial nucleus which receives input from both sides.1 A peripheral lesion of the facial nerve before branching at the pes anserinus will cause paralysis of all segments of the ipsilateral face, and a distal injury beyond the pes anserinus will result in a focal paralysis.
The facial nerve serves numerous roles. The special visceral efferent fibers from the facial motor nucleus innervate the muscles of facial expression, the posterior belly of the digastric muscle, the stylohyoid, and the stapedius muscles. General visceral efferent fibers carry signals from the superior salivatory nucleus in the pons to provide lacrimal and salivary function. Special visceral afferent fibers convey taste from the anterior two-thirds of the tongue. General visceral afferent fibers carry sensation from the palate via the geniculate ganglion, and general somatic afferent fibers via the geniculate ganglion carry sensory signals from the auricle and external auditory canal.
Falloppio (also known as Fallopius) (Fig. 30.1) identified the facial nerve course within the temporal bone around aD 1550.2 The facial nerve (Fig. 30.2) can be divided anatomically into six segments. The intracranial (first) segment enters the fallopian canal medially at the porus acousticus.4 The meatal (second) segment includes the nerve portion from the porus acousticus to the narrowest area (0.68 mm average) at the meatal foramen.3 The facial canal is approximately 30 mm in length from Bell’s bar to the stylomastoid foramen.4 A periosteal band reinforces the meatal foramen, and the nerve occupies 83% of the canal at this location. In comparison, the nerve only occupies 73 and 64% of the tympanic and mastoid segments, respectively.4,5 These unique attributes of the meatal foramen make it a likely place for facial nerve compression when there is neural edema. The labyrinthine (third) segment of the facial nerve is the shortest and thinnest segment (3 to 5 mm) within the facial canal.6 It occupies the region between the cochlea and the labyrinth. The tympanic (fourth) segment is also known as the horizontal segment and runs through the middle ear. After the second genu, the mastoid (fifth) segment runs vertically from the region of the horizontal semicircular canal to the stylomastoid foramen where it exits to become the extratemporal (sixth) segment.
Blood supply to the facial nerve comes from three major sources: (1) branches from the anterior inferior cerebellar artery (AICA), (2) a branch of the middle meningeal artery, and (3) a branch of the postauricular artery. Direct branches from the AICA supply the nerve as it travels from the pons to the internal auditory canal (IAC). A loop of the AICA usually gives rise to the internal auditory artery. Within the facial canal, the nerve receives blood supply from the petrosal branch of the middle meningeal artery and the stylomastoid artery, which branches from the postauricular artery.7 The petrosal artery provides the main blood supply to the geniculate ganglion and later anastomoses with the stylomastoid artery. The postauricular artery, which enters the temporal bone through the stylomastoid foramen, supplies the mastoid and tympanic segments of the nerve. Further nourishment to the nerve is supplied via the anastomotic vascular system within the epineurium.8
Pathophysiology of Nerve Injury
Both Sunderland and Seddon have developed terminology to describe the spectrum of nerve injury.9,10 Sunderland has described five degrees of injury, which correspond to Seddon spectrum of neuropraxia, axonotmesis, and neurotmesis.10 First-degree injury (neuropraxia) is the mildest form of injury and produces a nerve conduction block where axoplasmic flow is still intact. Purely neuropraxic injuries typically recover fully. Second-degree injury (axonotmesis) involves axonal injury, but the endoneurium remains intact. Wallerian degeneration of the nerve does occur and axoplasmic flow is impaired, but the nerve still tends to recover. Third-degree injuries affect the axon, myelin, schwann cell layer, and endoneurium. Synkinesis (involuntary simultaneous movement in multiple muscle groups) can occur from aberrant regeneration of the nerves because of loss of normal architecture; recovery is incomplete. Fourth-degree nerve injury is more severe and involves the endoneurium and perineurium. Severe synkinesis or mass motion is possible, and the entire unilateral face may appear to move as one unit. The most severe nerve injuries are termed fifth-degree injuries, also called neurotmesis. In a fifth-degree injury, the entire cross-section of the nerve including epineurium is affected; significant recovery is not expected without repair.
Physical Examination
A complete head and neck examination should be completed on all patients presenting with facial paralysis. Emphasis should be placed on the otologic examination, palpation of the neck and parotid glands, and a complete clinical cranial nerve examination. The history should include questions regarding onset and duration of paralysis, history of recurrent paralysis, trauma, head and neck surgery, infections, travel history, other neurologic symptoms, family history of cranial nerve disorders, and risk factors for neoplasm.
Facial motion should be closely inspected and graded using the House-Brackmann facial nerve grading system (Table 30.1).11 Patterns of paralysis that are incomplete and do not fit a classic House-Brackmann grade should also be described in terms of the degree and region (s) of the face affected. It is useful to observe the patient at rest as well as performing a variety of facial motions such as a smile, pucker, eye closure, nose wrinkle, rapid blinking, and showing of the mandibular teeth. Cranial nerve III, the oculomotor nerve, opens the eye actively via contraction of the levator palpebrae muscle. One must be careful not to confuse partial eye closure with simple relaxation of the oculomotor nerve that allows the eyelid to close partially because of gravity. Wrinkling of the periorbital skin secondary to contraction of the orbicularis oculi muscle, which is innervated by the facial nerve, is a clear indication that the facial nerve is at least partially functional. A mastoid dressing may also confuse a novice clinician by pressing down on the brow creating the appearance of partial eye closure. An additional potential for examiner error is to confuse movement of a facial unit resulting from vigorous movement of the contralateral side with active movement of the injured side. To eliminate this error, the examiner may place a finger in the midline of the face (e.g., on the glabela or philtrum) to prevent the translation of forces across the midline during examination. Clinical photography and/or videography are useful tools to help follow the progression of facial paralysis. If the patient has a complete paralysis, electrical testing may be indicated.
Facial Nerve Testing
The goal of facial nerve electrophysiologic testing is primarily focused on differentiating mild neuropraxic injuries that are likely to recover with severe axonal injuries that may require intervention. Typically, surgical exploration for decompression or repair is not indicated in patients with incomplete facial nerve paralysis, except in cases of neoplasms. However, in those patients with complete facial paralysis, electrical testing is often useful to identify those patients who might benefit from surgical intervention by differentiating primarily neuropraxic palsies from those with severe axonal degeneration.
Topognostic Testing
Topognostic testing attempts to localize a facial nerve lesion by evaluating deficiencies in facial nerve function on physical examination. For example, a lesion proximal to the chorda tympani and distal to the greater superficial petrosal nerve (GSPN) should, theoretically, be associated with dysgeusia on the affected side with preservation of lacrimal gland function. Additionally, one would expect a lesion proximal to the GSPN to cause impairment in tear production and consequently an abnormal Schirmer test. Gantz concluded that the best way to assess facial nerve degeneration from a conduction block sufficiently in Bell’s palsy is by the use of intraoperative evoked electromyogram (EEMG). When he correlated his EEMG findings with topognostic testing, he found that the Schirmer test was accurate in only 61% of patients.12 Topognostic testing is useful for theoretical discussions and teaching purposes, but because of abundant anatomic variations with the facial nerve and its branches, caution should be used when trying to localize a lesion using this method.
Maximal Stimulation Test
The maximal simulation test (MST) utilizes an electrical source such as a Hilger nerve stimulator. The Hilger nerve stimulator is battery powered and has a two-ended probe attached by wire to the current generator (Fig. 30.3). The current intensity is adjustable. The stimulus wand has a metallic ball on the end of each probe. To perform the test, the patient’s skin is cleansed, and conducting gel is placed on the probe and the skin to enhance conduction. One end of the probe is placed near the stylomastoid foramen and the other end is placed on the face or neck in areas corresponding to the five major branches of the facial nerve: (1) the forehead and eyebrow, (2) the periorbital area, (3) the nasolabial region, (4) the lower lip, and (5) the neck or platysma region.13 The patient should be informed that they might feel mild discomfort from electrical stimulation. Some patients, especially children, may require sedation.
The stimulator delivers a 0.6-ms pulse. The intensity is started at a low (e.g., 1 mA) current and is gradually increased to a supramaximal stimulus that is defined by the highest intensity tolerated by the patient, up to 10 mA. The supramaximal stimulus is typically at least 5 mA.14 The MST is usually started on the 3rd day after the onset of paralysis to allow time for proximal axonal degeneration to reach the stylomastoid foramen. The test is repeated for several days, up until 2 weeks or until return of some facial function is seen or when complete loss of response to stimulation occurs. The paralysis is graded by comparing the affected side to the normal side. The response is categorized as: (1) equal, (2) minimally decreased (less than or equal to 50% of the normal side), (3) markedly diminished (less than or equal to 25% of the normal side), or (4) absent.6 With Bell’s palsy, if the two sides of the face are equal with maximal stimulation at postonset day 10, there is a 92% chance of complete resolution of the paralysis. Approximately 86% of patients who had markedly diminished or absent responses to maximal stimulation at day 10 ultimately had incomplete facial motion recovery. Those patients that did recover completely after having a markedly diminished or absent response to maximal stimulation in a study by May had been operated upon.14 Although the MST has merit and is available to most clinicians, it is limited in that it requires excellent patient cooperation and depends on the subjective comparison of facial motion by the clinician, many of whom have limited experience with the test.
Table 30.1 House-Brackmann Facial Nerve Grading System
Grade | Description | Characteristics |
I | Normal | Normal facial function |
II | Mild dysfunction | Gross: slight weakness noticeable on close inspection; may have very slight synkinesis At rest: normal symmetry and tone Motion: Forehead: moderate to good function Eye: complete eye closure with minimal effort Mouth: slight asymmetry |
III | Moderate dysfunction | Gross: obvious, but not disfiguring difference between sides; noticeable, but not severe synkinesis, contracture, and/or hemifacial spasm At rest: normal symmetry and tone Motion: Forehead: slight to moderate movement Eye: complete eye closure with effort Mouth: slightly weak with maximal effort |
IV | Moderately severe dysfunction | Gross: obvious weakness and/or disfiguring asymmetry At rest: normal symmetry and tone Motion: Forehead: none Eye: incomplete closure Mouth: asymmetric with maximal effort |
V | Severe dysfunction | Gross: only barely perceptible motion At rest: asymmetry Motion: Forehead: none Eye: incomplete closure Mouth: slight movement |
VI | Total paralysis | No movement |
Adapted from reference 11.
Electroneuronography
Electroneuronography (ENoG) is an EEMG, elicited by transcutaneous electrical stimulation of the facial nerve while recording the compound muscle action potentials (CMAPs) from the facial musculature. ENoG testing, like the MST, requires waiting for 3 days after nerve injury to allow Wallerian degeneration to occur. Premature testing will be misleading, as it will demonstrate normal responses regardless of the severity of proximal nerve injury. Both MST and ENoG are electrophysiological compromises wherein the nerve is stimulated distal to the stylomastoid foramen because stimulating more proximally through the temporal bone requires much higher levels of stimulation than extratemporal stimulation and would be extremely painful. Transtemporal magnetic stimulation has been shown to easily penetrate the temporal bone with minimal pain to elicit a proximally generated facial nerve response.15 Further studies and advancements are required to better focus the proximal site of magnetic stimulation before it can be an effective clinical tool.
Figure 30.3 Hilger facial nerve stimulator, Model H3. (WR Medical Electronics Company, Minnesota, United States.)
The benefit of ENoG over the MST is that ENoG allows for objective measurements of facial activity, thereby eliminating the need for a potentially subjective comparison between sides by the clinician. Its disadvantage is that it is rarely available to most clinicians, typically requiring referral to a tertiary center. ENoG is performed by placing bipolar surface recording electrodes along the nasolabial fold in positions that allows for maximal EMG response.16 A ground electrode is placed on the patient’s neck. The buccal and zygomatic branches are stimulated with a bipolar surface stimulator placed near the stylomastoid foramen. The maximal response is recorded on both sides and repeated to ensure intertest consistency. The peak-to-peak amplitudes of the action potentials are measured in microvolts (μV) and compared with the normal side (Fig. 30.4)6 The results are reported as the percent reduction of the paralyzed side compared with the unaffected side according to the following formula: percent degeneration = 100 − (paralyzed side amplitude (μV)/unaffected side amplitude ([μV]) × 100. The percent reduction in CMAP amplitude is considered proportional to the degree of facial nerve axonal degeneration.
The latency of the response (time in milliseconds from onset of stimulation to the beginning of the CMAP) is also measured. Unlike amplitude, clinical studies have not shown a strong correlation of latency to prognosis, but latency should be noted to avoid confusing a true facial nerve response from an early response elicited by inadvertent stimulation of the nearby masseter muscle.
While it would be convenient to simply tape the bipolar recording electrodes at the nasolabial fold (i.e., “standardized lead placement”), studies have shown that these recording electrodes need to be slightly adjusted while watching the EMG response to obtain the maximal response (i.e., “optimized lead placement”).16 Simply altering the recoding electrodes a few millimeters can create marked differences in the measured response. Thus, training and experience in ENoG testing are required to maximize accuracy.
Figure 30.4 An electroneuronography sample from a patient with a right-sided facial weakness shows the peak-to-peak amplitude of the facial response on the right side (B1) is only approximately 58% compared with the amplitude of the response on the left side (A1). This corresponds with a 42% amplitude reduction or degeneration on the right side.
Patients with facial nerve paralysis from inflammatory etiologies, such as Bell’s palsy, demonstrate a diminished CMAP on the side of the paralysis when axonal degeneration is present. ENoG can also be used to evaluate facial palsies because of trauma. In addition, patients with neoplasms can also be assessed to rule out subclinical neural changes despite clinically normal function by determining if they have decreased CMAP amplitude on the affected side as well as a prolonged latency between stimulus and onset of CMAP.17
Radiologic Evaluation
Computed tomography (CT) studies and magnetic resonance imaging (MRI) are both useful in the work-up of a facial paralysis thought to be related to neoplasm, cholesteatoma, or other structural problem. CT scanning is most useful for osseous architecture while MRI can evaluate the facial nerve, soft tissues, and inflammatory processes exceedingly well (Fig. 30.5). While many clinicians do not advocate imaging for all cases of facial paralysis that are acute and consistent with classic idiopathic Bell’s palsy, one should have a low threshold for obtaining imaging modalities when patients exhibit symptoms of neoplasm (Table 30.2), history of trauma, or signs and symptoms of another structural insult to the nerve.
CT scanning is the modality of choice for temporal bone trauma and middle ear disease and should be performed with thin 1 to 2 mm high resolution cuts in both axial and coronal planes with emphasis on the temporal bones, cerebellopontine angle (CPA) cisterns, brainstem, and parotid glands inferiorly to the angle of the mandible.18 If technically feasible, 1.5 mm axial sections through the second genu and mastoid segments of the facial nerve can add additional information about the status of the nerve within the fallopian canal.18 Intravenous (IV) contrast should be considered if there is suspicion of neoplasm.
Table 30.2 Clinical Features of Facial Nerve Dysfunction Secondary to Neoplasm
• Slowly progressive paralysis beyond 3 wk • No return of facial function after 6 mo • Facial hyperkinesia (e.g., hemifacial spasm) • Other cranial neuropathies • Recurrent ipsilateral paralyses • Palsy of a single facial nerve branch • Pain |
Adapted from reference 44. mo, months; wk, weeks.
Gadolinium-enhanced MRI with T1- and T2-weighted 3 mm fine-cut axial, sagittal, and coronal sequences through the brain, brainstem, temporal bones and IACs, and parotid glands will adequately assess the facial nerve structures from the brainstem to the extratemporal portion. The use of fat suppression is important when evaluating a previously operated field, especially if adipose grafting or a reconstructive flap was used during closure. Standard MRI imaging of the brain without thin sections (3 mm) through the IACs is insufficient to rule out facial nerve pathology including vestibular and facial schwannomas.
Vestibular schwannomas as well as intracanalicular facial neuromas will enhance with gadolinium on T1-weighted imaging and cause displacement of cerebrospinal fluid in the IAC and/or CPA causing a corresponding hypointense region on T2-weighted sequences. One should use caution when interpreting contrast-enhanced MRI sequences of the IAC during the acute phase of facial palsy. Neuritis has been shown to cause enhancement of the nerve, and this enhancement can mimic a small intracanalicular vestibular schwannoma. Bell’s palsy tends to cause enhancement in multiple segments of the facial nerve with the epicenter most commonly at the perigeniculate area. It is not associated with significant enlargement of the nerve itself, and the enhancement can persist for at least 4 months.19
MRI evidence of facial nerve inflammation secondary to Ramsay Hunt syndrome has been shown to persist for up to 6 months even after return of facial function in some patients.20,21 Although some authors suggest that the borders of the enhancing region (sharp for neoplasms versus “dull and cloudy” for inflammation) can be used to distinguish differing pathologies, a conservative approach is to allow time for inflammation to subside and repeat an enhanced MRI in 6 months.21
Facial Palsies
Facial nerve palsies can be complete or incomplete, unilateral or bilateral, congenital or acquired. Inflammation of the facial nerve is the most common cause of paralysis. The preponderance of evidence indicates that most “idiopathic Bell’s palsies” are because of the herpes simplex virus (HSV) whereas a lesser number are caused by the varicellazoster virus (VZV).22 Four percent of idiopathic palsies may recur. Bilateral facial palsies are much less common than unilateral problems. Bilateral palsies occur in less than 2% of all facial palsies and are often associated with diseases that are systemic.23 Examples of systemic diseases affecting the facial nerve include sarcoidosis, Guillain-Barre syndrome, botulism, Lyme disease, poliomyelitis, syphilis, osteopetrosis, and meningitis.
Bell’s Palsy
The term “Bell’s palsy” has been erroneously employed by many health care providers to describe all facial palsies. Furthermore, while it was historically used to refer to those facial palsies that truly have no identifiable etiology, numerous studies over the last three decades have shown that most sudden facial palsies are because of herpes simplex.
Figure 30.5 A computed tomography scan of the temporal bones from a patient with a right-sided facial neuroma shows a bone defect (arrows) on both the axial (A) and coronal (B) sections. Axial (C) and coronal (D) T1-weighted magnetic resonance imaging following administration of gadolinium contrast shows an enhancing neoplasm (arrowheads) on the facial nerve.
Approximately 51% of all facial nerve disorders are ultimately diagnosed as Bell’s or idiopathic facial palsy after proper evaluation (Table 30.3).6 The treatment of Bell’s palsy remains controversial with different groups advocating combinations of observation, corticosteroids, antivirals, and surgical decompression. The underlying pathophysiology is largely agreed to be ischemic necrosis of an edematous facial nerve within the fallopian canal with the greatest site of entrapment at the meatal foramen.4 Historical intraoperative findings, MRI,19 histopathology,24,25 and intraoperative electrophysiologic testing12,26 support this mechanism of nerve injury.
Corticosteroids have been used for the treatment of Bell’s palsy for their antiinflammatory properties. A recent review by Salinas et al reported a 33% rate of incomplete recovery of facial function in patients who did not receive corticosteroids. Patients who received corticosteroids had significantly less incidence (23%) of incomplete recovery 6 months after randomization. Corticosteroids have also been shown to reduce the absolute risk of synkinesis and autonomic dysfunction by 14% without an increased risk of adverse effects.27 Multiple studies have concluded that corticosteroids are beneficial for patients with Bell’s palsy.27,28
HSV and VZV have been implicated in the pathophysiology of Bell’s palsy. In 1975, Adour showed that patients with Bell’s palsy were significantly more likely to have serum antibodies to HSV compared with matched controls.29 More recently, muscle biopsies and cerebrospinal fluid have been collected from patients within 72 hours of acute Bell’s palsy onset as well as from controls with chronic otitis. Using polymerase chain reaction (PCR), 10% of patients had detectable levels of the viral DNA, compared with no patients in the control group.30 Although PCR does not appear to be a good diagnostic test for Bell’s palsy, it does suggest a role for HSV-1 and VZV in the pathogenesis.
Although corticosteroids are widely accepted as beneficial in the treatment of Bell’s palsy, monotherapy with antiviral medication has not generally been successful.31 A meta-analysis by Almeida et al in 2009 looking at 18 trials with 2786 patients found that although antivirals alone were not associated with benefit, the combination of corticosteroids and antiviral agents seems to have a synergistic effect with a trend toward improved benefit versus either drug alone.27 Furthermore, because antiviral medications are not associated with a high rate of adverse events and are available in relatively affordable formulations, their use in Bell’s palsy carries low risk.27,31 Conclusive evidence regarding the absolute benefits of antiviral medications needs further investigation.
If surgical decompression for Bell’s palsy is considered, it should include the meatal foramen segment of the facial nerve as it exits the IAC. The efficacy of various treatment options is difficult to assess because 85% of patients recover spontaneously.32 Neurophysiologic testing attempts to identify which patients are among the 15% who will fail to recover spontaneously and might benefit from surgical decompression. ENoG appears to be the best available prognostic test.14,33
Gantz’s thesis on the surgical management of Bell’s palsy suggests that ENoG in combination with voluntary EMG can more accurately identify those patient’s with Bell’s palsy who will most likely benefit from surgical decompression of the facial nerve.34 Patients who have greater than 90% neural degeneration on ENoG and the absence of voluntary EMG potentials have the highest probability of poor recovery. This poor prognosis group benefited from surgical decompression of the facial nerve including the portion medial to the geniculate ganglion when performed within 2 weeks of onset of total paralysis. Although Gantz’s findings are specific to Bell’s palsy, the principles of using ENoG for prognosis have been extrapolated to other types of facial paralysis including trauma and Ramsay Hunt syndrome.
The benefit of surgical decompression for recurring palsies appears to be more certain with all reported patients being free of recurrences.35,36 Some authors believe decompression is indicated to help exclude an occult neoplasm, which may not be identified on radiographic or clinical examination.37
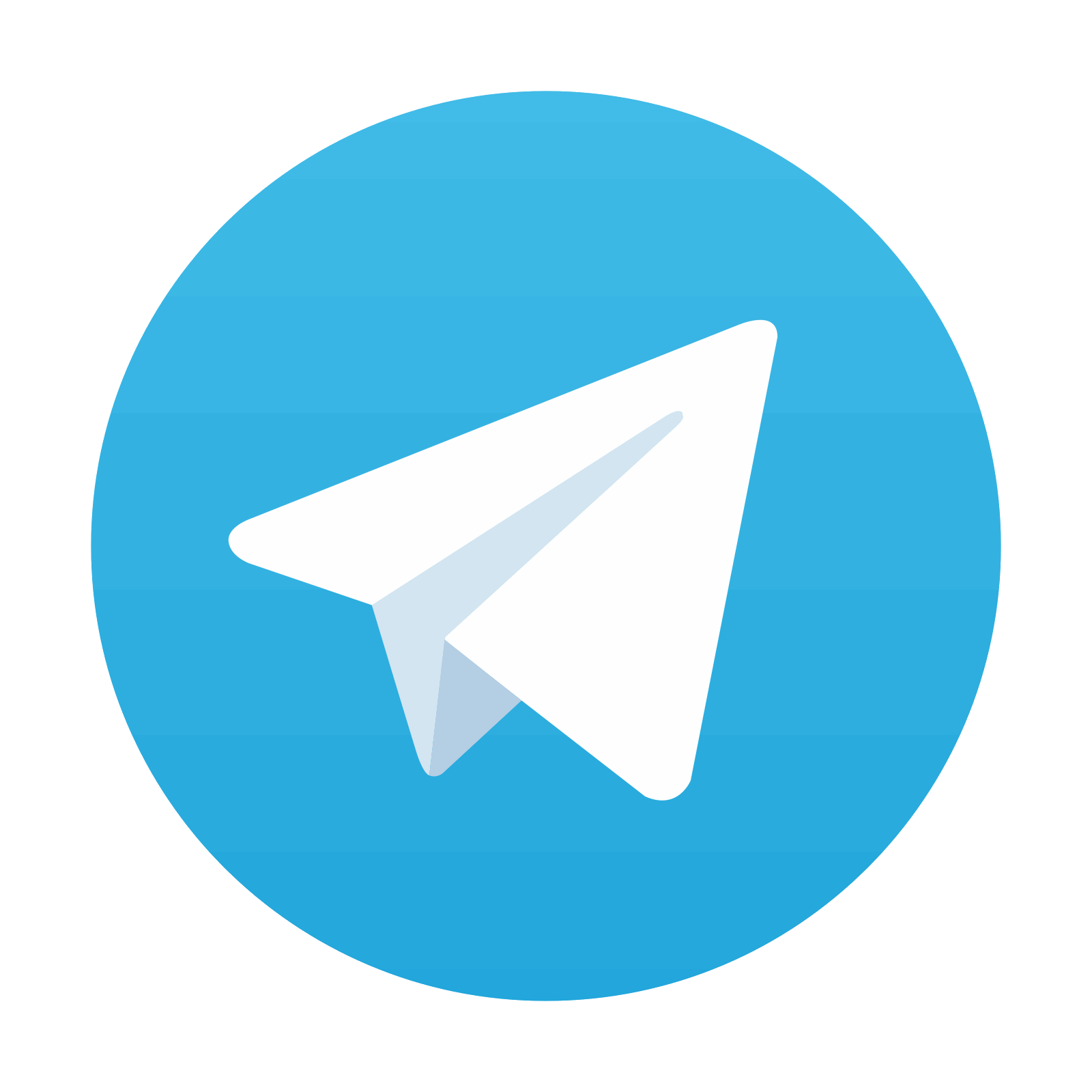
Stay updated, free articles. Join our Telegram channel

Full access? Get Clinical Tree
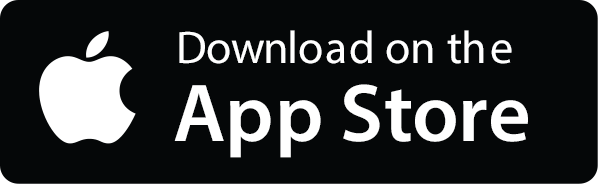
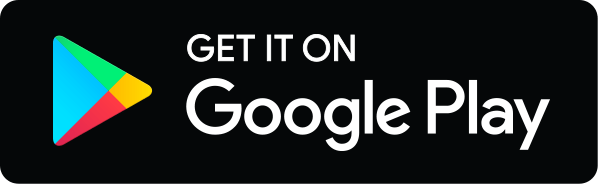