Fig. 10.1
Induction of stem cells or transdifferentiation requires developmentally important factors that regulate process of differentiation, migration, proliferation, and apoptosis
10.2 Pluripotent Stem Cells and Development
Induction of pluripotent stem cells has been used as an efficient strategy to achieve regeneration of several organs. However, induction protocols are based on results from previous studies of developmental biology. During development, cells choose which germ layer fate to acquire (ectoderm, mesoderm, or endoderm) after fertilization, and they are then specifically induced into the desired cell type. The factors used to induce pluripotent stem cells into desired cells include those that determine early-stage dorsoventral or anteroposterior axis in development: the transforming growth factor-β (TGF-β) superfamily (Activin, Nodal, and bone morphologic protein), Wnt signaling proteins, and fibroblast growth factors (FGFs) [5] and their inhibitors. Currently, multiple types of organs and/or their tissue stem cells from all three germ layers can be induced from pluripotent stem cells.
10.2.1 Mesodermal Organs
Various mesodermal organs, such as those of the hematopoietic system and cardiomyocytes, can be successfully induced. Hematopoietic stem cells are induced from pluripotent stem cells by overexpression of a single transcription factor, HoxB4, and co-culture with OP9, a stromal cell line from mouse bone marrow [6, 7]. Transplantation of induced hematopoietic stem cells has also been shown to rescue a phenotype of sickle cell anemia in mouse models [6]. HoxB4 was selected for induction because it is specifically expressed in definitive hematopoietic stem cells but not in the developing, early-stage organs or the primitive hematopoietic organ, yolk sac [7]. In case of cardiomyocytes, they were first generated from embryonic stem cells (ESCs) by spontaneous differentiation [8]. Since then, numerous protocols based on discoveries in developmental biology have been optimized. To begin with, markers of early cardiac induction were selected based on the study showing that platelet-derived growth factor receptor alpha (Pdgfr-α) and fetal liver kinase 1 (Flk-1) are co-expressed in the cardiac crescent of embryos at embryonic day (E) 7.0–7.75 and in the linear heart tube at E8.5 [9]. Next, the protocol to direct pluripotent stem cells toward the mesodermal lineage [10, 11] by adding Activin A [12–14], bone morphogenetic protein 4 (BMP-4) [15], and FGF-2 [16] that mimic the signaling environment of the primitive streak in a post-gastrulation embryo was determined. Cardiac differentiation was subsequently induced by inhibiting Wnt [17] and TGF-β signaling [18]. Cardiomyocytes thus derived from pluripotent stem cells have been integrated into infarcted guinea pig hearts to improve cardiac function [19].
10.2.2 Endodermal Organs
The induction of pancreatic cells as model of endodermal organs is well characterized. The protocol for pancreatic cell induction is divided in two stages: induction of definitive endoderm followed by the induction of a pancreatic progenitor. The study of vertebrate development demonstrates that Nodal induces embryonic differentiation into the endoderm [20]. Based on this result, an efficient protocol for generating definitive endoderm in vitro from pluripotent stem cells was developed [21]. In this protocol, Activin A, TGF-β family member, was used since it can be easily produced as a recombinant protein, and they can signal via similar downstream pathways with those of Nodal. The development of pancreatic endoderm from definitive endoderm is inhibited by sonic hedgehog (Shh) signaling [22], which in turn is inhibited by the signal from the notochord. This repression of the Shh signaling is essential for the development of pancreatic mesoderm [23]. In addition, retinoic acid signaling is necessary for the specification of the pancreatic and liver endoderm from the endodermal germ layer [24], and FGF10 is required for the expansion and branching of the pancreatic epithelium [25]. The combination of these three factors, namely, retinoic acid, the Shh inhibitor cyclopamine, and Fgf10, was found to successfully induce pancreatic epithelium in vitro from the definitive endoderm derived from pluripotent stem cells [26].
10.2.3 Ectodermal Organs
Several ectodermal organs such as the skin regenerate without treatment, while neurons or retinal tissues were considered to be incapable of regeneration. Currently, even these organs can be induced from pluripotent stem cells. To this end, iPSCs are propagated in media supplemented with FGF-2 (basic fibroblast growth factor) to obtain neural precursors. The transplantation of these neural precursors has been shown to facilitate functional recovery in Parkinson’s disease model and spinal cord injury [27, 28]. These studies are based on published research, which demonstrated that FGF-2-responsive progenitor cells can be isolated and cultured from the adult rat hippocampus [3]. Originally, FGF-2 was used to stimulate the proliferation of neuronal precursors from embryonic brain [29] or embryonic spinal cord [30]. Among the cell populations in the retina, retinal progenitor cells [31] and both photoreceptor cells as well as the retinal pigment epithelium (RPE) that support photoreceptor cells [32, 33] were induced from pluripotent stem cells by mimicking retinal developmental processes in a step-wise fashion. For the induction of retinal progenitors, ESCs were treated with Wnt and Nodal antagonists (Dkk1 and Lefty A) and Activin A [31]. These experiments utilized a plethora of transcription factors expressed at various developmental stages to define the cell populations obtained in the induction process.
10.3 Transdifferentiation and Development
In contrast to the induction of pluripotent stem cells, the transdifferentiation of mature cells is still less efficient. However, since pluripotent stem cells are induced from mature differentiated cells by overexpressing several factors that are specifically expressed in pluripotent stem cells [34], reprogramming has been tested in multiple types of organs. Even in cases of reprogramming of differentiated cells into other types of differentiated cells (direct conversion, or transdifferentiation), developmentally important factors have been utilized and some kinds of mature cells were successfully induced (see Chap. 21) [35–39]. The proteins that govern transdifferentiation are transcription factors that are specifically expressed in embryonic or matured target cells or their progenitor cells and that are important for the development, maintenance, or both of the desired cell type.
10.4 Future Direction
As described in this chapter, regeneration of organs recapitulates embryonic development in numerous aspects. Both processes involve the differentiation, migration, proliferation, and apoptosis of various cell types. Many of the same key signaling pathways that are activated during embryonic development are also activated during the regeneration process. Despite these similarities, there are a number of important differences between the molecular mechanisms that regulate regeneration and embryonic development, and these may be partly responsible for the inability of regeneration of organs into their original uninjured state.
References
1.
Zhao T, Zhang ZN, Rong Z, Xu Y. Immunogenicity of induced pluripotent stem cells. Nature. 2011;474(7350):212–5. doi:10.1038/nature10135.PubMedCrossRef
2.
Araki R, Uda M, Hoki Y, Sunayama M, Nakamura M, Ando S, et al. Negligible immunogenicity of terminally differentiated cells derived from induced pluripotent or embryonic stem cells. Nature. 2013;494(7435):100–4. doi:10.1038/nature11807.PubMedCrossRef
3.
Gage FH, Coates PW, Palmer TD, Kuhn HG, Fisher LJ, Suhonen JO, et al. Survival and differentiation of adult neuronal progenitor cells transplanted to the adult brain. Proc Natl Acad Sci U S A. 1995;92(25):11879–83.PubMedCentralPubMedCrossRef
4.
Svendsen CN, Clarke DJ, Rosser AE, Dunnett SB. Survival and differentiation of rat and human epidermal growth factor-responsive precursor cells following grafting into the lesioned adult central nervous system. Exp Neurol. 1996;137(2):376–88. doi:10.1006/exnr.1996.0039.PubMedCrossRef
5.
Andoniadou CL, Martinez-Barbera JP. Developmental mechanisms directing early anterior forebrain specification in vertebrates. Cell Mol Life Sci. 2013;70(20):3739–52. doi:10.1007/s00018-013-1269-5.PubMedCentralPubMedCrossRef
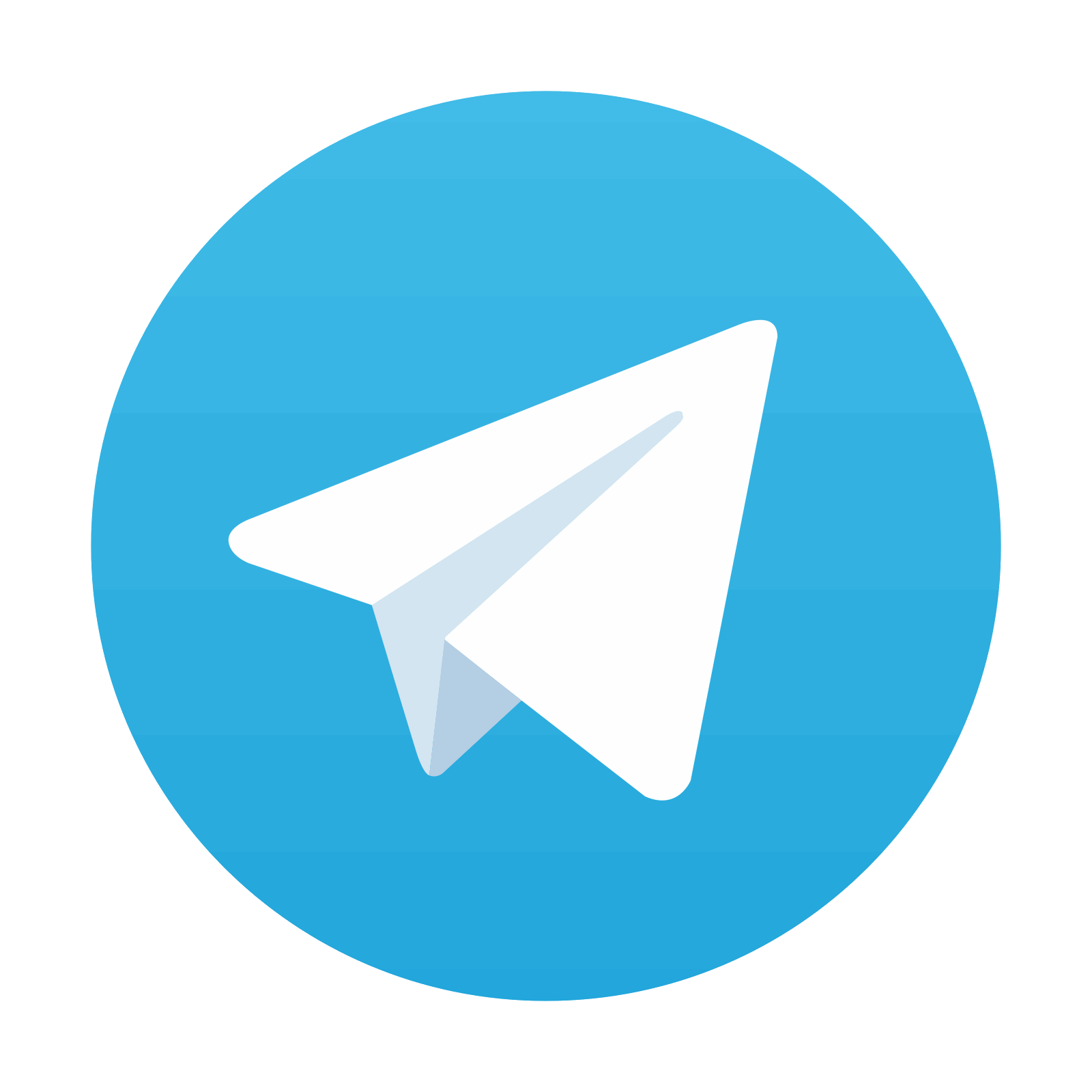
Stay updated, free articles. Join our Telegram channel

Full access? Get Clinical Tree
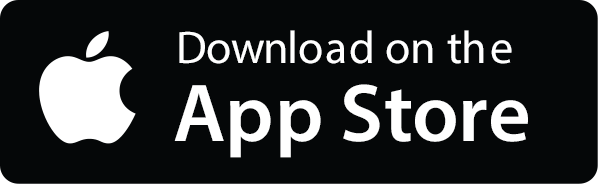
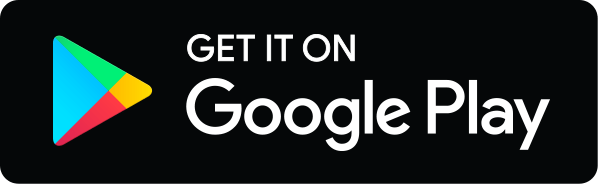