Fig. 22.1
Overview of dedifferentiation-mediated hair cell regeneration. Dedifferentiation-mediated hair cell regeneration involves a dedifferentiation step (dashed arrow) and a subsequent redifferentiation step (arrow). iPS transcription factors (TFs) and epigenetic modifiers would facilitate dedifferentiation of otherwise mature cochlear supporting cells
The cyclin-dependent kinase inhibitor, p27 kip1 , is an early marker of presumptive sensory region that will generate hair cells and supporting cells [15]. Deletion of p27 kip1 led to an extension in the normal developmental limit in proliferation of cells in the cochlea [16]. More recently, Ono et al. demonstrated that knockdown of p27 kip1 with short hairpin RNA-expressing vectors resulted in the cell-cycle reentry of post-mitotic supporting cells in the postnatal mouse cochleae ex vivo [17]. However, in parallel with the proliferation of supporting cells by knockdown of p27 kip1 , there was an activation of the apoptotic pathway in proliferating supporting cells, suggesting that additional manipulations are required to achieve hair cell regeneration [17].
The MYC gene family members, which in mammals include c-Myc, L-Myc, and N-Myc, are basic helix-loop-helix leucine zipper transcription factors that play a prominent role in regulating cell proliferation and differentiation [18]. T58A variant of c-Myc initiated cell cycle reentry of postmitotic supporting cells of adult mouse utricles and a portion of the cells that reentered the cell cycle expressed the hair cell marker myosin VIIa [7]. If myosin VIIa-positive cells that reentered the cell cycle originated from the supporting cell population, ectopic c-Myc expression may be capable of dedifferentiating supporting cells into otic progenitor cells [7]. However, c-Myc gene therapy is not a realistic approach for stimulating regeneration in humans due to its potential oncogenic characteristics [19].
Multipotent otospheres, which are sphere-forming cells derived from dissociated cochlear supporting cells of neonatal mice, are similar to cochlear epithelial cells, in that both of them have the same methylation status of Sox2 enhancers and the silenced Nanog and Oct3/4 promoters [20]. Lou et al. demonstrated that Oct3/4, Klf4, Sox2, and c-Myc reprogrammed otospheres into iPS cells [21], suggesting the possibility of dedifferentiation of cochlear supporting cells at postnatal stages. What remains to be performed are detailed analyses of the process of reprogramming otospheres, which contributes to insights into dedifferentiation of cochlear epithelial cells in tissue, characterizing cochlear progenitor cells, and identification of key molecules for otic induction. Needless to say, we need to take into account the difference between the artificially-produced otospheres and the original cochlear epithelial cells, as otospheres possess a proliferative capacity unlike postnatal cochlear epithelial cells in situ.
Several experiments have demonstrated that dedifferentiation of mature mammalian cells occurred in vivo: mature B cells were reverted to uncommitted hematopoietic progenitors by conditional Pax5 deletion in mice [22]; mature airway epithelial cells were dedifferentiated into stable and functional multipotent stem cells after the ablation of the airway stem cells [23]; and astrocytes were dedifferentiated into proliferative neuroblasts in the adult brain by overexpression of Sox2 [24]. More recently, Abad et al. demonstrated that transitory induction of iPS cell TFs in mice resulted in teratomas emerging from multiple organs, suggesting that even ultimate dedifferentiation can occur in vivo [25]. These precedents give us a proof of principle that cochlear supporting cells are dedifferentiated into otic progenitor cells in situ, which is an important step toward functional hair cell regeneration in the mammalian inner ear.
22.2 Epigenetics of Cochlear Sensory Epithelium
Epigenetics is a study of heritable changes in gene expression that occur without a change in DNA sequence [26]. Epigenetic regulation plays a pivotal role in cell differentiation and dedifferentiation. The two most well-known mechanisms of epigenetic alternations are DNA methylation and histone modifications. DNA methyltransferase-mediated methylation of cytosines in CpG islands recruits methyl CpG-binding proteins, histone deacetylases, and other associated proteins that change chromatin structure and prevent appropriate transcription factors from accessing the DNA, therefore silencing the gene expression as a result [27]. Mutai et al. demonstrated that an epigenetic regulatory mechanism, represented by DNA methylation and expression of Dnmt3a and 3b, occurred in the mammalian auditory epithelium during postnatal development, suggesting a role of epigenetic regulation during postnatal inner ear development [28]. Two class I histone deacetylases were expressed in the sensory epithelium of the avian utricle and treatment with histone deacetylases inhibitors decreased in supporting cell proliferation in the avian utricle [29]. Thus, histone deacetylation is a positive regulator of regenerative proliferation in the avian uticle. Waldhaus et al. demonstrated that the degree of methylation of the otic Sox2 enhancers NOP1 and NOP2 was correlated with the dedifferentiation potential of postmitotic supporting cells into otic stem cells, concluding that the stemness in the organ of Corti is partly dependent on the epigenetic status of NOP1 and NOP2 [20]. More recently, Wanda et al. reported that epigenetic factors involved in histone modification such as NuRD and PRC2 were present in the neonatal organ of Corti [30]. Cellular reprogramming by epigenetic modification in other system was demonstrated that 5-azacytidine, an inhibitor of DNA methylation, caused fibroblasts to differentiate into muscle and fat cell [31]. Therefore, epigenetic factors expressed in cochlear sensory epithelium are targets for drug intervention to induce dedifferentiation of a supporting cell into a hair cell, by reactivating the cell’s endogenous regenerative potential.
22.3 Concluding Remarks
Here we reviewed recent advances of research in cellular dedifferentiation and its potential application to hair cell regeneration. Although its impact on hair cell regeneration will be tremendous, further studies are mandatory to transfer basic findings gleaned from animal experiments to clinics as this field is still in its infancy.
References
2.
Poss KD, Wilson LG, Keating MT. Heart regeneration in zebrafish. Science. 2002;298(5601):2188–90. doi:10.1126/science.1077857.PubMedCrossRef
3.
Takahashi K, Yamanaka S. Induction of pluripotent stem cells from mouse embryonic and adult fibroblast cultures by defined factors. Cell. 2006;126(4):663–76. doi:10.1016/j.cell.2006.07.024.PubMedCrossRef
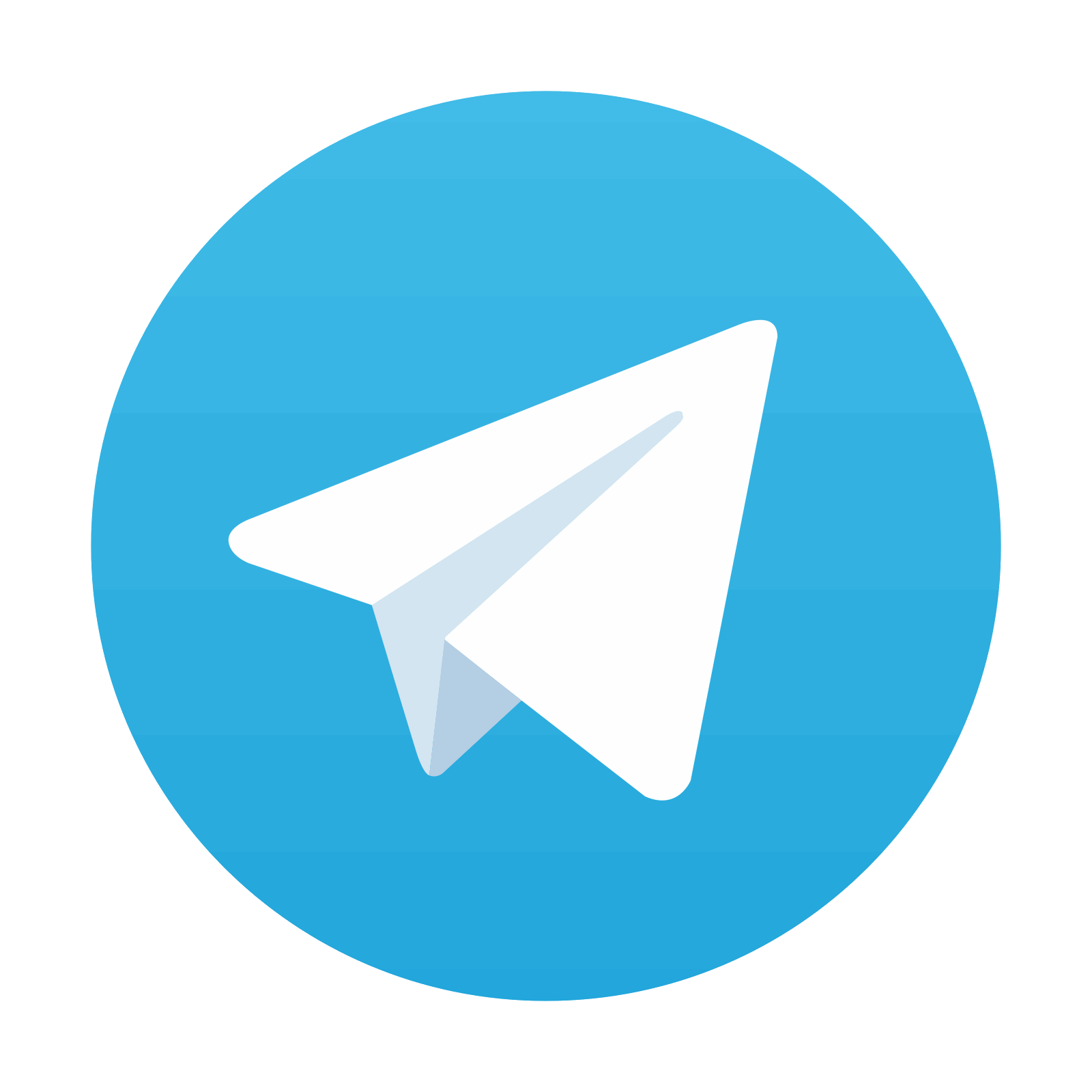
Stay updated, free articles. Join our Telegram channel

Full access? Get Clinical Tree
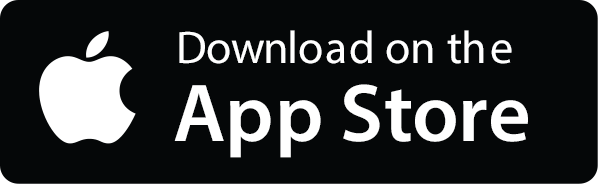
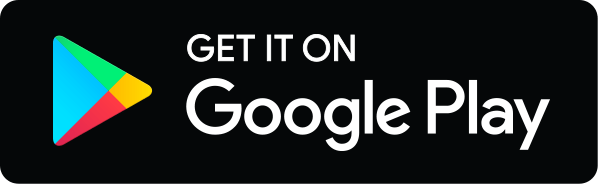