31 Core Messages • Virtual surgical planning is useful as an educational and teaching instrument by allowing three-dimensional analysis of the clinical problem and visualization of idealized digital manipulations. Without the ability to transfer the virtual plan into reality, however, it remains just that: an educational tool. • The incorporation of computer-aided design and computer-aided modeling technology into the head and neck workflow process facilitates transfer of the virtual plan into reality and theoretically has a positive effect on functional and esthetic treatment outcomes, reduced operating times, and improved quality. • Computer planning and intraoperative navigation (computer-aided surgical simulation) may add safety and predictability during extirpative and reconstructive surgery of the head and neck. The age of virtual surgery made its clinical debut in the early 1990s with the use of frameless stereotaxy, or intraoperative navigation. Intraoperative navigation was initially developed for medical purposes as an aid to performing safe neurosurgical and endoscopic sinus surgical procedures.1–5 Recently, its use has been expanded to a variety of anatomic regions and it is now used to improve accuracy and predictability in reconstructive head and neck/craniomaxillofacial surgery, including complex orbital repair,6 head and neck oncologic surgery,7–9 and craniofacial surgery.10 Intraoperative navigation offers the advantage of three dimensional (3D) visualization of the patient’s anatomy and access to areas of the facial skeleton that once required larger incisions and increased hard tissue exposure. Modern navigation systems allow precise location of anatomic landmarks or implants with a margin of error of 1 to 2 mm when compared with the projected computer tomography (CT) in the operating room.11,12 Computer-aided design (CAD) and computer-aided modeling (CAM) software and the easy acquisition and transfer of Digital Imaging and Communications in Medicine (DICOM) data have facilitated the development of various proprietary software programs for use in planning and implementing surgical procedures in the head and neck region/craniomaxillofacial skeleton. These software systems have been incorporated into previously developed navigation systems to create a computer-aided surgical simulation (CASS) system that may be applied in head and neck ablative and reconstructive surgery. Contemporary software allows the surgeon to analyze the patient with head and neck cancer by performing three-dimensional (3D) measurements of tumor volume and skeletal relationships and to manipulate deformed or missing anatomy by mirror imaging, segmentation, or insertion of unaltered or ideal skeletal constructs (Fig. 31.1). The virtual reconstruction may be transferred to reality (the patient) by using custom stereolithographic models (SLMs), implants, and/or cutting jigs that are constructed by using a CAD/CAM process or through image-guided surgery in the form of intraoperative navigation performed to the idealized virtual image (Fig. 31.2). The accuracy of the surgical procedure may then be confirmed by using modern portable intraoperative CT scanners (Fig. 31.3). Third-party service providers have made this software and rapid prototyping technology available and accessible to most surgeons. Surgery of the anterior and lateral skull base has traditionally been characterized by lengthy and extensive transcranial operations, often performed with suboptimal visualization and with a modest degree of unpredictability in terms of obtaining negative resection margins and minimizing complications. Frameless stereotaxy has been advocated as a method to facilitate subcranial approaches to the anterior skull base, to improve the surgeon’s ability to achieve safe, clear resection margins, and to minimize complications.13–16 Virtual approaches using intraoperative navigation can be incorporated into existing anterior skull base protocols, such as that defined by Dubin et al, to facilitate both subcranial ablation and reconstructive surgery when indicated.17 Figure 31.1 Workflow diagram of the initial clinical and radiographic analysis. Virtual two-dimensional and three-dimensional measurements of surgical resection are made. Fibula is virtually osteotomized and adapted to native mandibular margins. Virtual splints are made and then fabricated to replicate virtual cuts during surgery. A reconstruction plate is then adapted to a stereolithographic model. Intraoperative navigation has also been advocated as a method to increase safety in mandibular ankylosis release or resection of lateral skull base tumors or other lesions involving the temporomandibular joint (TMJ), pterygomaxillary fossa, and/or infratemporal fossa.14,18 The senior author has combined these techniques into a protocol for skull base tumors that uses navigation-assisted tumor resection to aid in safely achieving negative en block resection margins, followed by custom CAM as a guide for accurate fibular reconstruction of the mandible. Schmelzeisen et al first described the use of navigation for improved safety in lateral skull base surgery in general and ankylosis release in particular.14 Malis et al later described a single-stage approach for ankylosis release combined with total TMJ replacement by using navigation as a guide to accurately place osteotomies and inset custom prostheses.19 Zhang et al recently reported the outcome of a case series by using digital preoperative segmentation, osteotomies, and preoperative shaping of a reconstruction plate on an SLM derived from patients undergoing costochondral graft reconstruction of the TMJ.20 Compared with a control group, computer-assisted TMJ reconstruction was found to have significantly shorter operating time; however, it was found to have no significant difference in accuracy when compared with those subjects who underwent conventional TMJ reconstruction. Similar methodology in a patient with TMJ bony ankylosis was used by Chandran et al,21 who built on the previous application of navigation protocols.22,23 Preoperative digital resection of the TMJ fossa and condylar head was performed; cutting guides to duplicate the resection were fabricated; and an appropriate alloplastic stock prosthesis was adapted to an SLM model, rendering successful total joint reconstruction. Figure 31.2 Splints and cutting guides for ablative and donor sites are fabricated in the SLA machine to replicate osteotomies made during virtual surgery. SLM, stereolithographic model. Perhaps the most significant advancement in reconstruction of the midface and mandible during the past 30 years has been the development of microvascular osteocutaneous flaps, particularly the free fibular osteocutaneous free flap (FFOF) and its ability to achieve dental implant-supported prosthetic rehabilitation.24–30 SLMs developed from 3D preoperative imaging have been used to assist in overcoming some of the limitations of the FFOF as it relates to ideal positioning of the neomandibular constructs and to allow the surgeon to assess the contour of the maxillomandibular complex by removal of the external deformation left by the tumor, creating “ideal” anatomic surfaces.31 In addition to transverse assessment of the jaws, sagittal relationships of the jaws may be assessed for proper anteroposterior positioning to create ideal orthogonal relationships. Inaccurate insertion of the neomandibular (fibular) construct will render dental implant-supported prosthetic rehabilitation difficult or impossible because of over- or underprojection of the reconstructed mandible. SLMs developed from 3D imaging allow for precise plate bending and improved maxillomandibular relationship, which facilitates ideal dental implant placement. Figure 31.3 Intraoperative computed tomography can be used before the patient leaves the operating room to confirm surgical resection and implant and graft placement. Figure 31.4 A 37-year-old woman with recurrent, previously resected squamous cell carcinoma of the tongue, invading the mandible and previous fibular reconstruction. (A) Preoperative appearance. (B) Preoperative computed tomography (CT) image demonstrating recurrent tumor with the erosion of the mandible. (C) CT images in the Digital Imaging and Communications in Medicine format imported into Surgicase CMF software (Materialise, Ann Arbor, Michigan, United States) for segmentation and insertion. The resection is planned, and the defect is analyzed for fibular reconstruction. Cutting paths are created and virtual cutting guides are planned. (D) By using custom CT fibular data, the virtual fibula is imported into the mandible to create ideal position of the neomanidbular construct. (E) Virtual fibular cutting guides are constructed based on the planned fibular reconstruction to provide cutting guides for the closing wedge osteotomies at the time of surgery. (F) Virtually altered stereolithographic model demonstrating the neomandible. The model is used to prebend a stock locking reconstruction plate. (G) Neomandible, mandibular cutting guides, and fibular cutting guides. (H) Surgical approach. (I) Mandibular resection using cutting guide. (J) Resection specimen. (K) Composite tissue defect following composite resection of the tongue, floor of mouth, and mandible. (L) Fibular construct inset into defect with prebent reconstruction plate. (M) Postoperative appearance following adjuvant chemoradiation therapy. (N) Postoperative intraoral appearance of patient prosthesis in place. Leiggener et al32 and Hirsch et al33 described the use of CAD/CAM technology to produce orthognathically ideal surgical outcomes for patients with segmental mandibular defects undergoing reconstruction with fibular free flaps. Using the surgical simulation software, surgery is simulated on a computer workstation. The virtual fibular and mandibular osteotomies are transferred to a rapid prototyping instrument and a guide stent is constructed to allow for accurate placement of osteotomies (Medical Modeling, Inc., Golden, Colorado, United States). The guide stent is sterilized and used intraoperatively for mandibular osteotomies at the time of resection and fibular closing-wedge osteotomies. In this fashion, the vascularized composite tissue is transferred to the appropriate anteroposterior, vertical, and transverse position (Fig. 31.4). Custom guide stents may also be used to aid the accurate dental implant placement to facilitate prosthetic rehabilitation.34 Roser et al, in 2010, compared their ability to reproduce the computer plan in a series of 11 patients who underwent segmental mandibulectomy with FFOF.35 3D computer models of the final reconstruction were obtained for comparison with the preoperative virtual plan. To make the desired comparisons, the 3D objects representing the postoperative surgical outcome were superimposed onto the preoperative virtual plan by using manual alignment techniques. These objects were then compared by 1-to-1 magnification for measurements of fibular bone volume, location of mandibular osteotomies, location of fibular osteotomies, plate contour, plate position on the fibula, and plate position on the mandible. Comparison was made between the virtual and final plates with regard to contour and position through superimposition overlays of the 3D models that are registered in the same coordinate system. The authors noted that the mean distance of the actual mandibular osteotomy compared with the virtual mandibular osteotomy was 2.00 ± 1.12 mm. The mean volume determined by the software program of the 11 virtual fibulas was 13,669.45 ± 3874.15 mm3 (range 9568 to 22,860 mm3), and the mean volume of the 11 actual postoperative fibulas was 12,361.09 ± 4161.80 mm3 (range 7142 to 22,294 mm3). The mean percentage volumes of the actual postoperative fibula compared with the planned fibula were 90.93% ± 18.03%. Katikaneni et al36 performed a study similar to that of Roser et al. In 16 patients and 104 osteotomies, the average linear variance from the preoperative plan in all tested locations was 1.49 to 4.91 mm, with the transverse dimension being the most unpredictable location (mean 4.91 mm difference) compared with the accuracy of the mandibular osteotomies (1.49 mm difference from planned) and length of fibular segments (2.27 mm difference). Additional studies that will evaluate the cost relative to a similar group of patients treated without computer planning are underway. Bell et al described the use of intraoperative navigation combined with custom cutting guides and guide stents to implement the virtual plan for patients undergoing maxillectomy or midface resection reconstruction using fibular free flaps.7–9 The planned reconstruction data from patients undergoing maxillary ablation were “back converted” from their proprietary language to the standard DICOM format, so that digital reconstruction may then be imported into a surgical navigation system (Intellect Cranial Navigation System, Stryker, Freiburg, Germany). The resection is performed under navigation guidance so that the ablative defect matches the planned fibular reconstruction. Custom guide stents are then used to recreate the fibular closing wedge osteotomies, so that the neomaxillary construct fits the ablative defect. The entire neomaxillary construct is then inserted under navigation guidance (Fig. 31.5). Hanasono et al also described favorable results in a single patient who underwent reconstruction of a total maxillectomy defect.37 Besides providing a chance for preoperative planning, the authors noted that computer-assisted preoperative planning also limits the number of incisions that has to be made on the face. Lethaus et al reported a similar method of reconstruction of midface defects by using computer-assisted free-fibula graft reconstruction and found favorable results.38 Despite the use of traditional reconstructive principles, lasting functional and esthetic complications, such as enophthalmos, may persist following orbital reconstruction of postablative defects.39 This is especially true following tumor resection with margins spanning multiple orbital walls. In these defects it is especially difficult to obtain premorbid orbital dimensions because of the lack of reliable bony landmarks to guide the placement of bony plates and implants. Specific areas of difficulty are reestablishing proper orbital volume, considering the need to reestablish the critical ethmoidal and antral bulges of the medial and inferior floors, respectively, as well as plate adaptation around the orbital apex. 3D virtual planning combined with intraoperative navigation has been shown to assist the surgeon in achieving ideal orbital volume and globe projection when compared to unoperated controls.39–41 Gellrich et al used 3D imaging in a study on subjects with increased enophthalmos and decreased globe projection secondary to posttraumatic orbital deformities.39 In their landmark study, the investigators mirrored the unaffected orbit to the affected, traumatized orbit by using 3D preoperative image software. They used this as their “goal” orbital dimensions, and the subjects then underwent orbital reconstruction by using intraoperative navigation. Navigation guidance was used to confirm plate placement of grafts at the “goal” dimensions set preoperatively. The investigators found a significant decrease in orbital volume and increase in globe projection in their study cohort. Markiewicz et al performed a similar study, using the imaging software Analyze (Mayo Clinic Biomedical Imaging Resource, Rochester, Minnesota, United States) to assess the change in orbital volume and globe projection in a cohort of subjects with defects secondary not only to trauma but to tumor ablation as well.42,43 In addition, they used SLMs for preoperative graft contouring as well as confirming graft placement by using intraoperative navigation. They found results similar those of the study by Gellrich et al, with a significant decrease in orbital volume, an increase in globe projection, a linear correlation between goal and actual orbital volume, and globe projection measurements following orbital reconstruction. CASS can be divided into four phases: (1) data acquisition phase, in which all clinical information, anthropometric measurements, and bite registrations are obtained; (2) planning phase, in which CT data are imported into a proprietary software program for the purposes of virtual planning before surgery; (3) surgical phase, which is performed by using CAD/CAM-derived SLMs, guide stents, occlusal splints, and/or intraoperative navigation; and (4) assessment phase, in which the accuracy of the treatment plan transfer is evaluated using intraoperative (or postoperative CT imaging).
Computer Planning and Intraoperative Navigation for Head and Neck Cancer
Site-Specific Applications for Computer Planning and Intraoperative Navigation
Skull Base
Mandibular Reconstruction
Palatomaxillary Reconstruction
Orbital Reconstruction
Computer-Aided Surgical Simulation
< div class='tao-gold-member'>
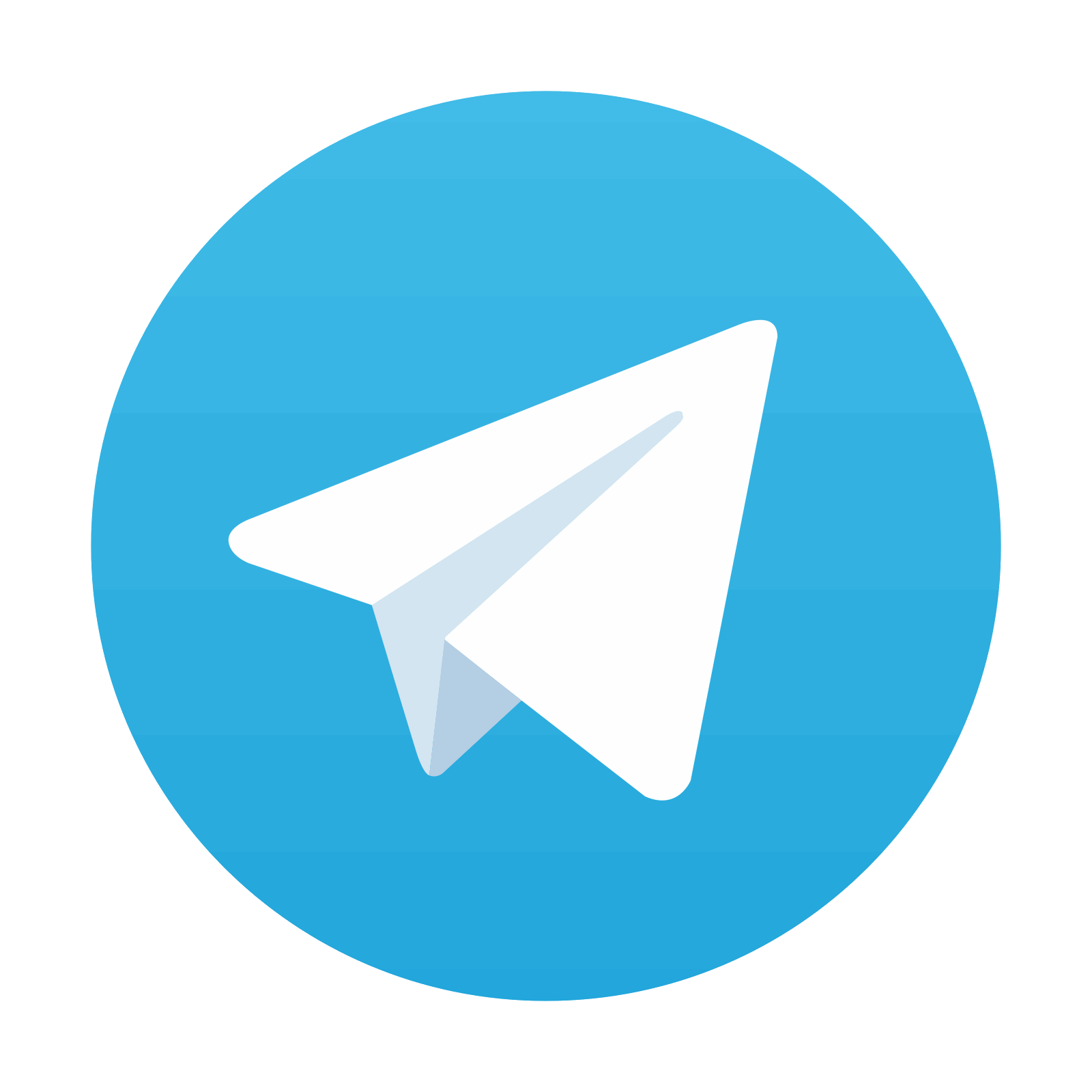