Purpose
To compare pathologic changes in the photoreceptors of eyes with idiopathic macular telangiectasia types 1 and 2 using adaptive optics scanning laser ophthalmoscopy (AO-SLO).
Design
Prospective cross-sectional study.
Methods
Eleven eyes with idiopathic macular telangiectasia type 1, 14 eyes with type 2, and 10 normal eyes underwent a full ophthalmologic examination, spectral-domain optical coherence tomography (SD-OCT), and imaging with an original prototype AO-SLO system. All eyes with idiopathic macular telangiectasia were examined with fluorescein angiography (FA), confocal blue reflectance, and microperimetry.
Results
Compared with normal eyes (29 017 ± 5507 cones/mm 2 ), those with idiopathic macular telangiectasia type 1 had significantly lower cone density on the temporal side (18 427 ± 4908 cones/mm 2 , P = .010). The FA leakage area (5.90 ± 3.23 mm 2 ) was larger than the dark regions (2.45 ± 2.40 mm 2 ) on AO-SLO ( P = .003)—which were observed only in the FA leakage area. Compared with normal eyes, those with idiopathic macular telangiectasia type 2 had significantly lower cone density in all areas ( P < .05 for all). Patchy dark regions were also seen in areas without FA abnormalities in 12 eyes. Higher mean retinal sensitivity correlated with greater mean cone density 0.5 mm from the center of the fovea (type 1: P = .010; type 2: P < .001).
Conclusions
Idiopathic macular telangiectasia type-specific differences in the distribution of photoreceptor abnormalities were shown in the AO-SLO images. For both idiopathic macular telangiectasia types 1 and 2, visual impairment was associated with cone damage.
Idiopathic macular telangiectasia is a rare condition, usually diagnosed in patients’ fifth or sixth decade, that causes a slow decline in visual acuity. Gass first described this condition in 1968 as “idiopathic juxtafoveolar retinal telangiectasis,” and later suggested multiple different classifications for distinguishing between different forms of the disease. In 2006, Yannuzzi and associates proposed the current name, along with a simplified classification scheme identifying 2 types of idiopathic macular telangiectasia: type 1, aneurysmal telangiectasia; and type 2, perifoveal telangiectasia. Typically, patients with idiopathic macular telangiectasia type 1 are men whose mean age at disease onset is 40 years. The telangiectasis is generally confined unilaterally to the temporal half of the macula, involving equivalent areas superior and inferior to the horizontal raphe. Idiopathic macular telangiectasia type 2 is equally common in both sexes and typically manifests bilaterally; however, disease severity may be asymmetric. Functional impairments typically include parafoveal scotomas, reading difficulties, and metamorphopsia; full-thickness macular holes may also occur.
Studies of optical coherence tomography (OCT) images from patients with both types of idiopathic macular telangiectasia have revealed macular structural abnormalities in the outer retina, including disruption of the line representing the junction of the photoreceptor inner and outer segments (IS/OS). However, OCT and other imaging modalities such as scanning laser ophthalmoscopy (SLO) fail to provide sufficiently detailed images of photoreceptor microstructure, primarily because of aberrations in ocular optics. These aberrations can be compensated for by using imaging systems that incorporate adaptive optics (AO), including a wavefront sensor to measure aberrations in the eye and a deformable mirror or a spatial light modulator to compensate for these aberrations in living eyes. Adding AO to imaging systems such as flood-illuminated ophthalmoscopes, SLO equipment, or OCT has allowed researchers to identify abnormalities in individual cone photoreceptors in patients with various retinal diseases.
We recently used AO-SLO to show the photoreceptor abnormalities that are associated with decreased visual function in idiopathic macular telangiectasia type 2. Using spectral-domain OCT (SD-OCT), we have also shown that retinal sensitivity is influenced by IS/OS disruptions in idiopathic macular telangiectasia type 1. However, there is still a need to understand how pathologic changes in photoreceptor patterns vary between these 2 types. In the current study, we used our prototype AO-SLO system to compare the photoreceptor changes observed in eyes with idiopathic macular telangiectasia types 1 and 2. We also compared these pathologic changes with abnormalities shown on images obtained by other modalities, and with abnormalities in the patients’ visual functioning.
Methods
All study investigations adhered to the tenets of the Declaration of Helsinki, and this prospective cross-sectional study using a prototype AO-SLO system was approved by the Institutional Review Board and the Ethics Committee of Kyoto University Graduate School of Medicine. The nature of the study, participation in the research study, and its possible consequences were explained to the study candidates, after which written informed consent was obtained from all participants.
Participants
Participants in this study included 11 eyes from 11 idiopathic macular telangiectasia type 1 patients (10 men and 1 woman; mean age: 60.4 years, range: 45-78 years), and 14 eyes from 7 idiopathic macular telangiectasia type 2 patients (3 men and 4 women; mean age: 66.7 years, range: 58-75 years). All individuals were recruited after they visited the Kyoto University Hospital (Kyoto, Japan) between September 4, 2008 and April 26, 2012, and none displayed any other macular abnormality or inherited color blindness. We also examined 10 eyes of 10 healthy volunteers (5 men and 5 women; mean age: 62.9 years, range: 36-69 years) with no eye diseases. Specific exclusion criteria for eyes with idiopathic macular telangiectasia included neovascular maculopathy (ie, age-related macular degeneration, polypoidal choroidal vasculopathy, retinal angiomatous proliferation, idiopathic choroidal neovascularization, angioid streaks), pathologic myopia, other causes of secondary macular telangiectasia (ie, retinal vein occlusion and radiation retinopathy), diabetic retinopathy, and any history or signs of retinal surgery, including laser treatment. We used the criteria proposed by Yannuzzi and associates to perform diagnosis and classification of idiopathic macular telangiectasia using slit-lamp biomicroscopy findings, fundus photographs, and fluorescein angiography (FA) findings. We excluded patients with diabetes from the diagnosis of idiopathic macular telangiectasia type 1.
All patients and volunteers in this study underwent a comprehensive ophthalmologic examination, including measurements of best-corrected visual acuity (BCVA) and intraocular pressure, axial length assessed using an IOL Master (Carl Zeiss Meditec, Dublin, California, USA), indirect ophthalmoscopy, slit-lamp fundus observation with a Goldmann 3-mirror contact lens, color fundus photography, SD-OCT, and AO-SLO. All patients with idiopathic macular telangiectasia also underwent simultaneous FA and indocyanine green angiography (IA), confocal blue reflectance imaging, infrared reflectance (IR) imaging, and fundus-monitoring microperimetry (MP). FA, IA, confocal blue reflectance, and IR images were acquired using a confocal SLO (Spectralis HRA+OCT; Heidelberg Engineering, Heidelberg, Germany). Confocal blue reflectance images were obtained using a 488-nm light stimulus, whereas IR images were obtained using an 820-nm light stimulus. Each study participant underwent all examinations within the same week.
The Adaptive Optics Scanning Laser Ophthalmoscopy System
The AO-SLO system used here was a simplified version designed and constructed in our laboratory based on previous reports detailing the usefulness of incorporating a wide-field SLO with an AO-SLO. The AO-SLO system is confocal, enabling creation of high-contrast “en-face” images in any plane; these images show individual cone photoreceptor cells. The system is composed of 4 primary optical subsystems: the AO subsystem, which includes the wavefront sensor and the spatial light modulator; the high-resolution confocal SLO imaging subsystem; the wide-field imaging subsystem; and the pupil observation subsystem, which facilitates the initial alignment of the subject’s pupil with respect to the optical axis of the AO-SLO system through adjustment of the chin rest position. The wavefront sensor measures aberrations in the whole eye, and the spatial light modulator compensates for these aberrations. Further details of our AO-SLO system are described in the Supplemental Material (available at AJO.com ).
Adaptive Optics Scanning Laser Ophthalmoscopy Imaging of Cone Mosaic Features
The AO-SLO images were obtained to cover a 3 × 3-mm-square area around the fovea. AO-SLO imaging was performed by shifting the focus from the retinal nerve fiber layer to the retinal pigment epithelium, then recording images that showed the cone mosaic. Offline, a montage of AO-SLO images was created by selecting the area of interest and generating each image to be included in the montage from a single frame, without averaging. Comparisons between AO-SLO images and wide-field images for the same eye were used to ensure that each image corresponded to the area of interest. The montage of AO-SLO images was used for the registration with images of other imaging modalities by matching the shape of the vessels.
We next applied the automated cone labeling process of Li and Roorda, which uses an algorithm implemented in MATLAB (Mathworks Inc, Natick, Massachusetts, USA) and a function from the MATLAB Image Processing Toolbox. We manually corrected the results of automated cone labeling on any images for which the algorithm failed to identify the cones, as follows: an experienced observer who was masked to the classification and other clinical findings examined each image after automated cone labeling; the observer manually labeled the areas where cones were visible but had not been identified, then entered this area into the computer software.
We selected areas without shadows of vascular components, and estimated cone density in areas 0.5 mm and 1.0 mm from the center of the fovea by instructing the computer software program to divide the number of cones in each area by the size of the area ( Figure 1 ). These distances from the center of the fovea were selected because the system does not always clearly show individual cones within the central fovea (a limitation that has been reported for similar systems ) but does clearly show cones >0.2 mm from the center. The center of the fovea, defined here as the center of the foveal avascular zone, was determined from the montage of AO-SLO images. To obtain accurate lengths of scans, we corrected the magnification effect in each eye using the adjusted axial length method devised by Bennett and associates.
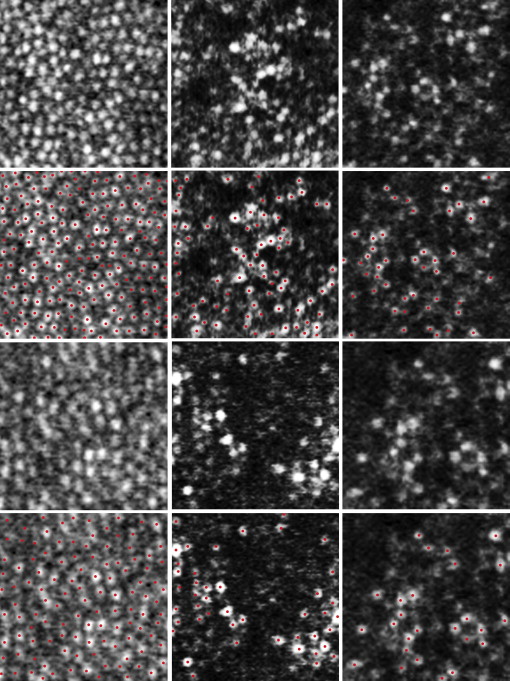
To quantify the extent of the leakage area in FA images (within areas scanned by AO-SLO) and the dark regions in AO-SLO images, each image was examined by 2 independent experienced observers (S.O. and K.T.) who used an image processing software (Image J) available from the National Institutes of Health (Bethesda, Maryland, USA) to manually identify any region of abnormality and to measure their respective areas. The area in each eye was taken as the mean of the areas measured by the 2 observers.
Spectral-domain Optical Coherence Tomography Evaluation of Photoreceptor Layer Features
SD-OCT examinations were performed in all eyes using a Spectralis HRA+OCT (Heidelberg Engineering). Horizontal and vertical line scans through the center of the fovea were obtained at a 30-degree angle, after which we performed 12 radial scans centered on the fovea. We then obtained volume scans (19 horizontal B-scans at 15 × 20 degrees; the distance between each B-scan was 237 μm). At each location of interest on the retina, 50 SD-OCT images were acquired and averaged to reduce speckle noise.
We manually marked each area of interest (ie, the area with intraretinal cystoid spaces in type 1 and retinal lamellar cavities in type 2) by drawing a line in each B-scan image taken by volume scan, then projecting it onto the IR image ( Figure 2 ). Each projected image was then merged into 1 en-face image; thus, the SD-OCT images were registered with images obtained by other imaging modalities ( Figures 3-6 ).
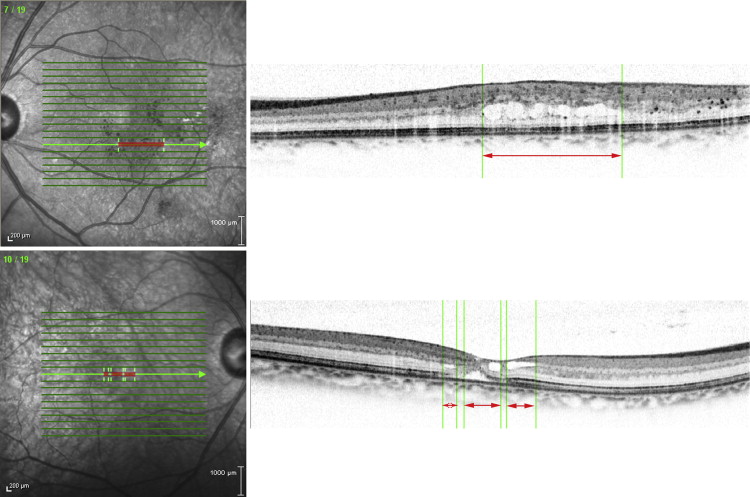
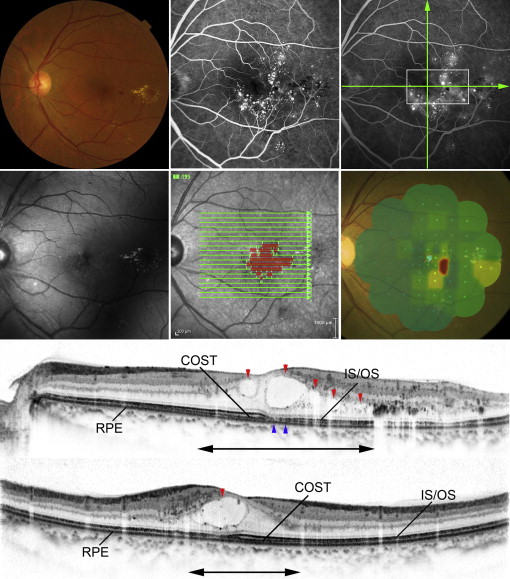
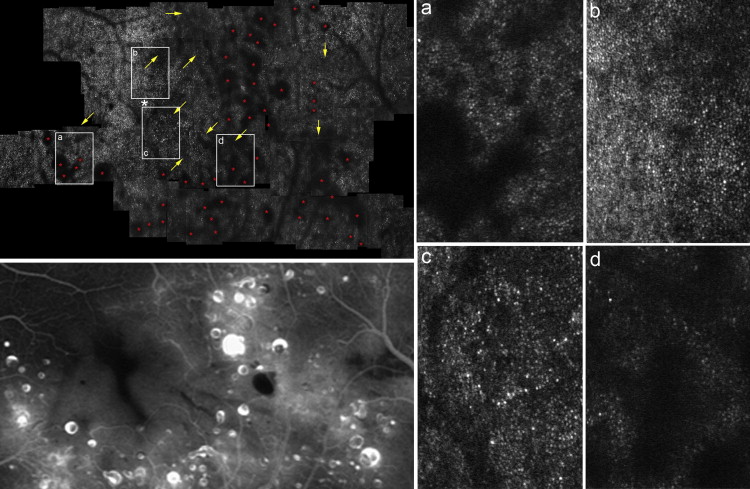
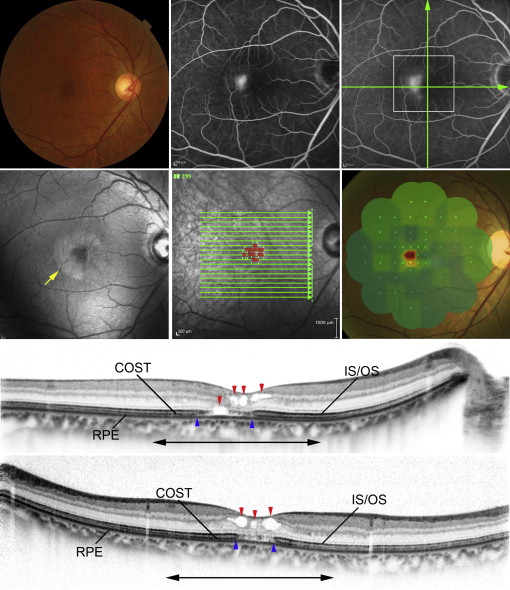
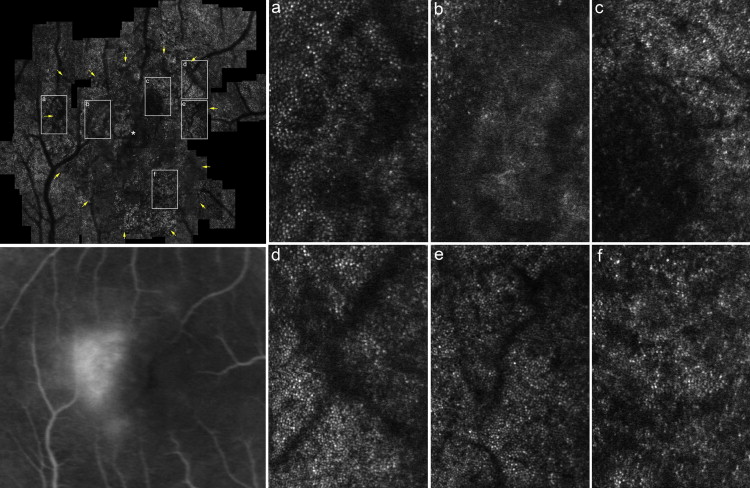
Microperimetry: Retinal Sensitivity Measurements
We used fundus-monitoring MP (MP-1; NIDEK, Padova, Italy) to measure retinal sensitivity. The MP-1 software can be set to automatically track fundus movements and evaluate every acquired frame for shifts in the directions of the x- and y-axes of the fundus with respect to a reference image obtained by an infrared camera at the beginning of the examination.
We used a 4-2-staircase strategy with Goldmann size III stimulus against a white background with illumination of 1.27 cd/m 2 to examine 55 stimulus locations covering the central 20 degrees. The differential luminance, defined as the difference between stimulus and background luminance, was 127 cd/m 2 at 0 dB stimulation; the maximum stimulus attenuation was 20 dB. The duration of the stimulus was 200 ms.
Statistical Analyses
BCVA measured using the Landolt chart was expressed as the Snellen equivalent or the logarithm of minimal angle of resolution (logMAR). We used Tukey-Kramer tests to evaluate differences in patient age, refractive error, and cone density for eyes with and without idiopathic macular telangiectasia. Student t tests were used to compare logMAR visual acuity between type 1 and type 2 and to evaluate the relationships between cone density and tomographic or angiographic changes. For interobserver measurements, 2-way mixed, average-measure intraclass correlation coefficients (ICC [3, K]) were obtained. We calculated the Pearson product moment correlation coefficient to determine associations between mean cone density and both logMAR visual acuity and retinal sensitivity. All statistical evaluations were performed in SPSS v.17 (SPSS Inc, Chicago, Illinois, USA). Significance was defined as P < .05.
Results
There were no statistical differences in age or spherical equivalent of refractive error between the patient and volunteer groups ( Table 1 ). The mean logMAR visual acuity was 0.16 in eyes with idiopathic macular telangiectasia type 1 and 0.12 in eyes with idiopathic macular telangiectasia type 2 ( P = .712).
Normal (n = 10) | Type 1 (n = 11) | Type 2 (n = 14) | P b | P c | P d | |
---|---|---|---|---|---|---|
Patient age (y) | 62.9 ± 9.8 | 60.4 ± 8.9 | 66.7 ± 6.4 | .539 | .627 | .155 |
Refractive error (diopters) | 0.6 ± 1.4 | −1.0 ± 1.7 | 0.4 ± 1.4 | .137 | .959 | .169 |
a Values are represented as mean ± standard deviation. All P values were obtained with Tukey-Kramer tests.
b Comparison between normal eyes and those with idiopathic macular telangiectasia type 1.
c Comparison between normal eyes and those with idiopathic macular telangiectasia type 2.
d Comparison between eyes with idiopathic macular telangiectasia types 1 and 2.
AO-SLO images revealed a regular cone mosaic pattern in normal eyes ( Figure 1 ). By contrast, we observed scattered dark patches in the cone mosaic in AO-SLO images of eyes with idiopathic macular telangiectasia type 1 ( Figure 4 ; Supplemental Figure 1 , available at AJO.com ). In eyes with idiopathic macular telangiectasia type 2, AO-SLO images showed ring-like (n = 5) or oval (n = 9) patchy dark regions with surrounding small dark patches in the cone mosaic ( Figure 6 ; Supplemental Figure 2 , available at AJO.com ).
In both normal eyes and those with idiopathic macular telangiectasia, cone density decreased with increasing distance from the center of the fovea ( Table 2 ). Compared with normal eyes, those with idiopathic macular telangiectasia type 1 had significantly lower cone density on the temporal side, 0.5 mm from the center of the fovea, and on the superior, inferior, and temporal sides, 1.0 mm from the center of the fovea; eyes with idiopathic macular telangiectasia type 2, on the other hand, had significantly lower cone density in all areas 0.5 mm from the center of the fovea. Cone densities were also significantly lower 1.0 mm from the center of the fovea on the superior and temporal sides. Compared with eyes with idiopathic macular telangiectasia type 1, those with idiopathic macular telangiectasia type 2 had significantly lower cone density on the temporal side, 0.5 mm from the center of the fovea.
Normal (n = 10) | Type 1 (n = 11) | Type 2 (n = 14) | P b | P c | P d | |
---|---|---|---|---|---|---|
0.5 mm | ||||||
Superior | 29 988 ± 6395 (20 910 – 40 245) | 22 095 ± 6107 (12 546 – 31 096) | 16 781 ± 9653 (0 – 37 103) | .068 | .001 | .225 |
Nasal | 29 655 ± 5563 (21 541 – 37 000) | 23 944 ± 6684 (13 959 – 33 991) | 20 261 ± 11 168 (0 – 35 981) | .292 | .032 | .541 |
Inferior | 28 080 ± 6167 (21 186 – 36 426) | 20 088 ± 6893 (13 467 – 34 862) | 18 875 ± 10 036 (0 – 34 425) | .079 | .027 | .928 |
Temporal | 29 017 ± 5507 (21 575 – 35 439) | 18 427 ± 4908 (12 343 – 29 810) | 9854 ± 10 394 (0 – 29 879) | .010 | <.001 | .026 |
1.0 mm | ||||||
Superior | 14 873 ± 2118 (10 255 – 16 836) | 12 002 ± 2145 (9697 – 16 580) | 11 817 ± 2929 (6246 – 16 865) | .033 | .015 | .982 |
Nasal | 14 767 ± 2044 (10 202 – 17 213) | 13 073 ± 1560 (10 252 – 15 061) | 13 621 ± 1796 (11 425 – 16 569) | .095 | .287 | .734 |
Inferior | 13 812 ± 2390 (9412 – 16 950) | 10 497 ± 2519 (8600 – 15 168) | 12 694 ± 2952 (3918 – 16 172) | .021 | .575 | .118 |
Temporal | 14 692 ± 2659 (9483 – 19 379) | 9824 ± 2784 (5689 – 12 965) | 6994 ± 6154 (0 – 16 065) | .045 | .001 | .269 |
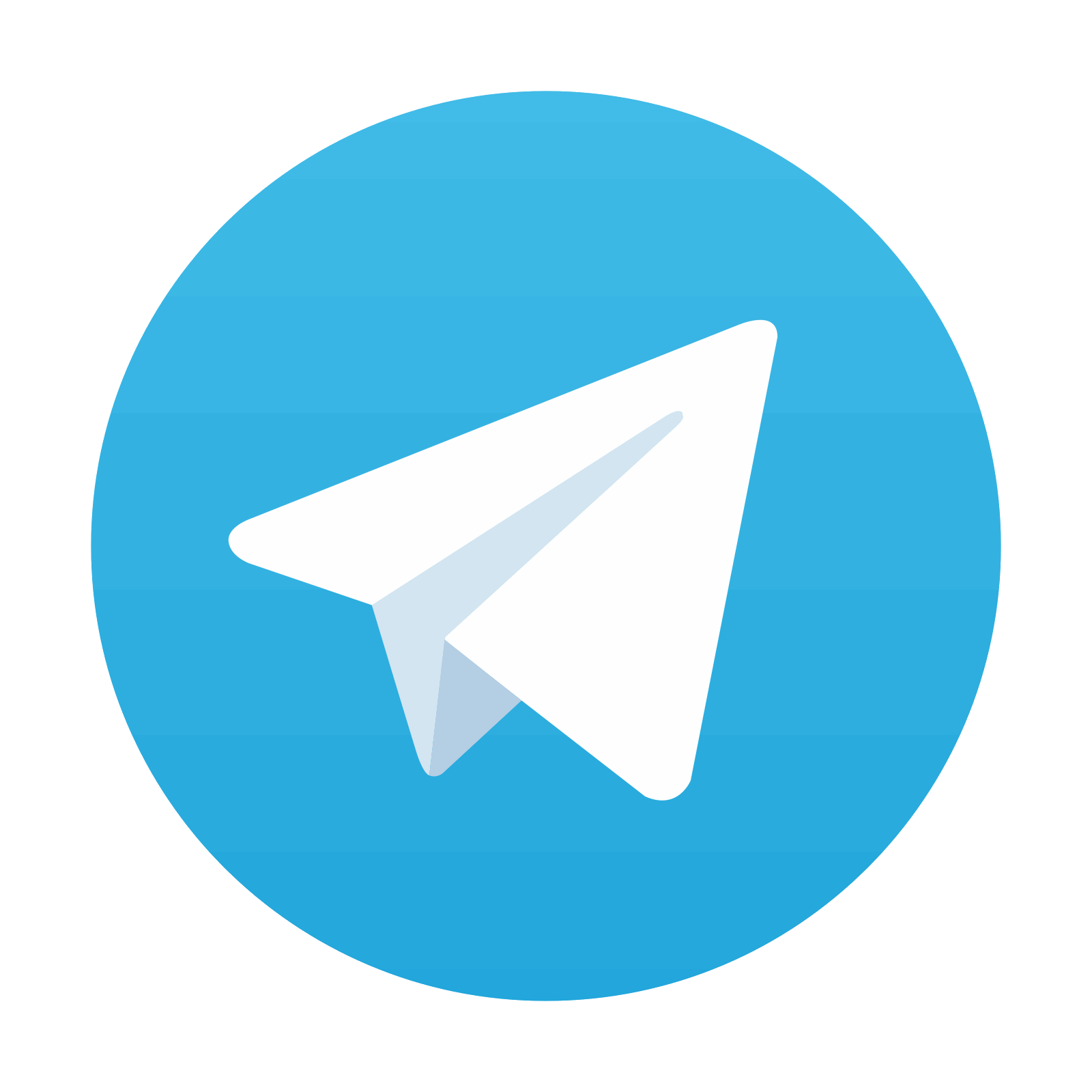
Stay updated, free articles. Join our Telegram channel

Full access? Get Clinical Tree
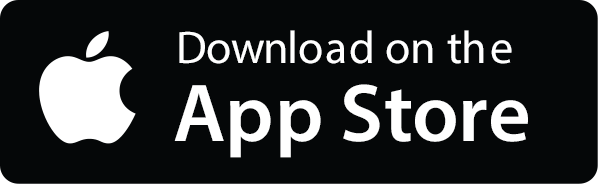
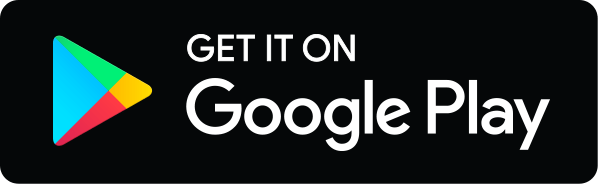
