9
Cochlear Implant Electrode History, Choices, and Insertion Techniques
J. Thomas Roland Jr., Tina C. Huang, and Andrew J. Fishman
♦ History of Cochlear Implantation
The history of cochlear implantation begins with the use of electricity to stimulate the ear in an attempt to produce a sensation of sound. Volta, in 1790, was the first to publish his results. Using his recently invented voltaic cell, he connected 30 or 40 cells, the equivalent of ˜50 V, to two metal rods, which he then inserted into his ears. When he closed the circuit, he received a sensation akin to being hit in the head and then described a sound like thickly boiling paste. Luckily, he chose not to repeat his experiment. Ritter reported his attempt to repeat Volta’s experiment using a battery with 100 to 200 cells in 1801. He also experienced disagreeable cerebral effects. Not until 1855 did another experimenter stimulate the ear with electricity. Duchenne used alternating current to produce a crackling sound. Brenner, in the 1860s, was able to use short current pulses to produce a sensation of sound, and in the 1930s several other researchers were able to use alternating currents in the ear to produce sound.1,2
Stevens at Harvard described the effect of auditory sensation due to alternating current passed through the head as the electrophonic phenomenon. He did several experiments using subjects with normal hearing and intact tympanic membranes and middle ears as well as patients who had prior surgery and no tympanic membranes. His first published report in 1937 showed that, in people with normal hearing and intact tympanic membranes, alternating current passed through the ears could produce sound of different frequencies. In addition, the subjects could recognize speech and music, but the quality was extremely poor and no word discrimination was possible. This led him to propose three different mechanisms for the production of sound: (1) direct stimulation of the auditory nerve, (2) cochlear receptors electrically tuned to different frequencies, and (3) mechanical vibrations produced by the alternating currents that stimulate the auditory organs in the same manner as sound vibrations. Further experiments led him to refine his three hypotheses. He proposed that in patients with normal ears, alternating current displaces the tympanic membrane, leading to mechanical vibration. Patients lacking a tympanic membrane either had the hair cells of the organ of Corti set into motion by the electrical current or had direct stimulation of the auditory nerve.3 Some subjects also experienced vertigo with stimulation, presumably from stimulation of the vestibular nerves.
The year 1957 ushered in the modern era of cochlear implants with the implantation of an electrode into a human patient by Djourno and Eyriès. A patient with large bilateral cholesteatomas had undergone bilateral temporal resections and was left with bilateral facial nerve paralysis and deafness. During the surgery for facial nerve grafting, an electrode was implanted into the stump of the remaining cochlear nerve. The patient was able to hear sound and differentiate frequency and intensity, but not speech. Unfortunately, the electrode broke twice and was not replaced a third time. Interestingly, Djourno is also the first person to describe using promontory stimulation with a transtympanic needle to verify a functioning cochlear nerve.4 Chouard, who had worked in Eyriès laboratory, carried on the work and developed one of the early multichannel implants.5,6
In the 1960s, Simmons at Stanford, Michelson at University of California–San Francisco, and House in Los Angeles all began implanting human subjects with various types of implants. Simmons, in 1964 and 1966, placed multiple electrodes into human subjects with no adverse effects. Both patients had direct auditory nerve stimulation and both patients were able to distinguish different frequencies, but no speech.7 He also performed several histologic studies in cats that did not show significant damage to auditory structures from prolonged electrical stimulation and damage limited to the most distal portions of the basal turn of the cochlear from electrode insertion.8 He, too, encountered what continues to plague implant makers today: the fragility of the electrical wires leading to the electrodes.9 In 1977, two patients were implanted with a four-electrode system into the auditory nerve. Their initial results showed that the patients were able to differentiate various frequencies and loudness levels.10
Michelson had also published experiments on cats in the late 1960s that showed no adverse effects from long-term stimulation with multiple electrodes placed within the cochlea. He then published his results on four patients with a bipolar electrode placed through the round window into the cochlea. Two patients perceived only noise with activation, but the other two patients were able to identify pure tones and had limited speech recognition.7 Again, technical failures due to breakage of the wires were a major impediment. This was corrected using methyl methacrylate cement to fix the receiver and wires to the temporal bone.11
House first implanted two patients with a single electrode system into the scala tympani in 1961. However, one was quickly explanted secondary to an increase in current requirements. The second patient had the single electrode replaced with a five-wire electrode system several months after his first implantation. This system, too, was explanted secondary to an inflammatory reaction.12 He teamed up with Urban and, in 1969, implanted another patient with their five-electrode system. Two more were then implanted in 1970. The third patient moved away from the area and the second patient had a device failure, but their first patient was able not only to differentiate sounds but also to carry on simple telephone conversations.12,13
Clark developed an early multichannel implant using biphasic current stimulation. He also began using a banded electrode array, instead of a two-dimensional electrode array, placed within the cochlea and driven with a transcutaneous data and power link. This was the precursor of the Nucleus cochlear implant.5,14 In Austria, Hochmair and Hochmair-Desoyer were also experimenting with multichannel bipolar electrode arrays.5,14
Once clinical trials began, cochlear implantation advanced rapidly in terms of device design as well as device placement. Clark’s first patient, implanted in 1978, was able to perceive pitch and gained some open set speech discrimination. Several other patients were implanted in 1979 and this experience led to the formation of Cochlear Ltd. by a collaboration between the University of Melbourne, Nucleus Ltd., and the Australian Government. The Nucleus 22 device (Cochlear Corporation, Sydney, Australia) was developed in 1981 and clinical trials began in 1982. The Nucleus 22 then evolved into the Mini 22 receiver/stimulator (Cochlear Corporation, Sydney, Australia). The Mini 22 improved on the original Nucleus 22 in terms of its smaller size as well as the incorporation of a magnet to hold the transmitting coil against the device instead of the previously used headset. The electrode array contained 22 evenly spaced platinum band electrodes supported within a straight silicone rubber molding and an additional 10 platinum bands to provide extra stiffness to facilitate insertion. The array had a maximal insertion depth of 25 mm into the scala tympani.15 The Mini 22 was then replaced with the Nucleus CI24M/SP5 cochlear implant system (Cochlear Corporation, Sydney, Australia). The Nucleus 24 device consisted of a titanium-encapsulated receiver-stimulator connected to 22 intracochlear electrodes and two extracochlear electrodes with the ability to deliver biphasic pulses in either bipolar or monopolar configuration.16
In 1987, the Clarion multichannel cochlear implant was developed by MiniMed Technologies, now the Advanced Bionics Corporation (Valencia, CA), in collaboration with the Department of Otolaryngology of the University of California–San Francisco and the Neuroscience Program Office of the Research Triangle Institute (Research Triangle Park, NC). The Clarion used 16 platinum-iridium (90:10) wire contacts encased within a curved silicone rubber carrier. Each wire ends in a 0.3-mm-diameter ball with the balls arranged in eight near-radial pairs with 2 mm between pairs. The 16 wires are stacked in a double-rib configuration and the total length of the electrode array was 25 mm. The external antenna connected to the internal components via a magnet.17 Because the electrode was precurved, the device required a special insertion tool to straighten the electrode prior to insertion.18 The electrode was inserted into a Teflon tube, which is then mated to the insertion tool, which contained a retractable slide. The tube containing the electrode was then placed into the cochleostomy and the slide advanced. As the slide advanced, the electrode was advanced into the cochlea. The original device, version 1.0, was introduced in 1991. Version 1.2, with a smaller case, was introduced in 1995.19 The Clarion was approved for use in adults in 1996 and for children in 1997.
The last company currently manufacturing cochlear implants is Med-El (Innsbruck, Austria). Its original device, the Med-El Combi 40, was a multichannel fast-stimulator device with eight intracochlear electrodes housed in a straight soft silicone carrier. The 16 electrode contacts, two interconnected contacts per electrode, were arranged in diametrically opposite pairs. The electrodes were driven in monopolar mode. The electrodes were spaced over 20.6 mm and had an average insertion depth of 30 mm. The device became available in the mid-1990s and, at the time, was the fastest implant available.20,21 The company’s next device, the Combi 40+ implant, was a 12-channel system with a thinner receiver/stimulator housing.22 The newest device is the Pulsar CI100, which continues to use the same design for the electrode array, but has improved processing capability.
As research into device design progressed, the potential advantages of perimodiolar placement of the electrode array came to light. With this goal in mind, several implant companies produced implants that brought the electrodes closer to the modiolus. Though the original Clarion array was already precurved, the array assumed an intermediate position within the scala tympani. The Advanced Bionics Corp. developed an intracochlear Silastic positioner that was inserted adjacent to the electrode to bring the array even closer to the medial wall. The positioner was a curved piece of Silastic thinner at the distal tip and thicker at the proximal end. The positioner was inserted using the insertion tool after the electrode was in position.23 In addition, the company developed a new electrode, the HiFocus electrode, to be used specifically with the electrode positioning system. It still contained 16 electrode contacts, but these contacts were arranged longitudinally along the carrier and had a large rectangular surface area. Dielectric partitions were located between each contact to reduce the spread of current. The HiFocus device was approved for use in adults in 2000.24 Unfortunately, the positioner was associated with an increased risk of meningitis and was taken off the market. The company’s current device, the HiResolution 90K, uses the “J” electrode, which maintains the precurved shape of the carrier and has a thinner case housed in Silastic for the receiver/stimulator. A perimodiolar Helix electrode is also currently under development and refinement.
Cochlear Ltd. developed the perimodiolar Contour electrode. The Nucleus CI-22 and CI-24 systems were both straight electrodes and assumed a position along the lateral wall of the scala tympani. The Contour electrode was precurved and tapered from 0.8 mm at the proximal end to 0.5 mm at the tip to minimize its volume. A platinum stylet within the lumen of the array holds the electrode straight before insertion. Removal of the stylet either after or during insertion allows the array to assume its perimodiolar shape and position. The array contains 22 platinum half-band electrodes positioned on the modiolar side of the array along the proximal 15.5 mm of the carrier. The array also has a silicone marker rib at 22 mm measured from the tip to guide the depth of insertion.25 It was approved for use in adults in 2000.26 The Contour electrode has recently been replaced with the Contour Advance electrode, which continues to utilize the perimodiolar design with 22 electrodes, but a softer tip to guide insertion and minimize insertion trauma. This device is deployed with the Advance Off Stylet (AOS) technique.
The initial debate between single versus multiple electrode systems has produced modern devices that all utilize multichannel systems. The most current challenge for cochlear implant manufacturers is to design an electrode that assumes the closest position to the modiolus without causing excessive insertional trauma and perhaps preserve residual cochlear elements and hearing.
♦ Intracochlear Trauma Due to Cochlear Implantation
Intracochlear trauma caused by cochlear implantation has been extensively reported in the literature. The short-and long-term structural and functional detrimental effects of trauma have been documented in animals but not in humans. The long-term functional effects of trauma are unknown in humans. In 1981 Leake-Jones et al27 showed that basilar membrane perforation caused focal spiral ganglion cell degeneration in animals. In a later report, Leake-Jones and Rebscher28 comment that neuronal elements can withstand chronic implantation if two prerequisites are met. The shape and mechanical properties of the array must be precisely controlled such that insertional trauma is minimized and that the materials and fabrication must be highly biocompatible. Miller et al29,30 in 1983 showed that fracture of the osseous spiral lamina in monkeys was associated with a significant decrease in spiral ganglion cell population and that degeneration might continue along the auditory neural pathways to the inferior colliculus. In a large postmortem human study, four separate studies reported no correlation between spiral ganglion cell counts and speech perception performance.31–34 Significant medial osseous trauma and neo-ossification was reported. Spiral ganglion cell counts, however, are not the only variable related to performance with a cochlear implant. Nadol35 suggests that there is no firm evidence that intracochlear trauma, found in several human postmortem temporal bones, caused further spiral ganglion cell degeneration. In 1999, Ketten36 performed computed tomographic (CT) radiographic analysis of living postimplantation patients showing cochlear trauma and ossification related to electrode trajectory and final position. Animal studies, human temporal bone studies, and radiologic studies document intracochlear trauma following cochlear implantation. Despite the discrepancy between findings in animal studies and human clinical results and postmortem temporal bone histopathology, few would argue against preservation of cochlear structures during cochlear implantation. Perhaps the subtle detrimental outcomes from cochlear trauma will become more apparent as patients with better hearing are implanted and more complicated signal processing and neuronal stimulation strategies are utilized. One might also argue that, within a single subject, performance would certainly be better with more surviving neurons to stimulate.
Currently, there are three cochlear implant electrode array designs: straight, precurved, and perimodiolar. As mentioned earlier, the recent designs have attempted to position the electrode array closer to the modiolus in an effort to decrease power consumption and prolong battery life as well as provide more specific neuronal subpopulation stimulation. Among the perimodiolar devices, there are three conceptual models: straight electrode design with a lateral tension band; precurved electrode with a straightening stylet; and space-occupying positioner lateral to the electrode array. The nature of observed intracochlear trauma differs with each of these designs.37–39
Other than the design characteristics of the aforementioned electrodes, surgical technique is a variable in cochlear trauma during cochlear implantation. Cochleostomy position, electrode insertion angle in two dimensions, insertional forces, kinking of electrodes, and the use of various tools and lubricants all affect the potential degree of trauma resulting from cochlear implantation.
Observations and conclusions in this chapter are based on the cumulative experience of more than 10 years of work developing and refining a cadaver temporal bone model for the study of cochlear implant electrode insertion dynamics, position analysis, and trauma reporting.40
The Model for Electrode Evaluation
Temporal bones are harvested from human cadavers, and the otic capsule is removed with limited surrounding bone to minimize bone density and allow optimal fluoroscopic imaging. A basal cochleostomy is performed and a lubricant (surgical grade glycerin diluted to 50% with saline) is infused into the cochlea to facilitate insertion and for bone dust removal, as is performed in all our live patient implantations. Under real-time fluoroscopic guidance, using standard microsurgical instrumentation and technique, electrodes are inserted through the cochleostomy. Multiplanar real-time images are captured to videotape, so that detailed analyses may be performed. Additionally, hydraulic pressure measurements can be performed by inserting a catheter into the superior semicircular canal ampulla and connecting to a pressure transducer and monitor (Fig. 9–1). Pressure fluctuations can be detected during lubricant infusion, and during electrode insertion and withdrawal.
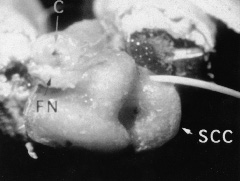
Figure 9–1 Thinned otic capsule with catheter placed in superior semicircular canal (SCC) ampulla. FN, facial nerve; C, cochleostomy. Fluoroscopic measurement evaluation, histologic sections, and hydraulic pressure measurements are obtainable from these specimens.
The temporal bones are next dehydrated and fixed in resin. Radial sections allow observation and imaging of the pars inferior, pars ascendens, and pars descendens in a plane perpendicular to the scalar lumen. This technique, which has been previously described, facilitates measurements of electrode position relative to cochlear structures and the evaluation of trauma.40,41 Additional sections at the cochleostomy and the cochlear apex can also be made (Fig. 9–2). Alternatively, serial sections can be performed as with standard thick section temporal bone histologic techniques.
Anatomic Regions of Potential Trauma
The anatomic sites and the potential mechanisms of cochlear implant–related trauma are categorized in Table 9–1. Damage to the spiral ligament and the outer scalar wall endosteum undoubtedly occurs with every cochlear implantation. Straight electrodes strike the outer wall at the mid pars ascendens in most cases.40–42 This outer wall contact is well seen on both fluoroscopic evaluations and in histologic preparations, and the trauma has been shown to cause neo-ossification and fibrosis that may affect electrode impedance characteristics and will also affect cochlear reimplantation. No evidence exists in human studies that injuries to the outer scalar wall and the spiral ligament affect spiral ganglion cell survival.
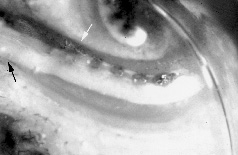
Figure 9–2 Longitudinal section through the basal turn of the cochlea. Black arrow cochleostomy; white arrow modiolar wall. Note the tip of the electrode in the second turn of the cochlea.
Damage to the basilar membrane also occurs in many cochlear implantations. Tears and disruptions have the potential to destroy the organ of Corti and the terminal dendritic processes that may result in spiral ganglion cell degeneration in humans.
Osseous spiral lamina fractures are a rare occurrence, but trauma to this structure disrupts the dendrites of the spiral ganglion cells, which might lead to further spiral ganglion cell degeneration.
Modiolar wall trauma and fracture represents the most severe form of cochlear implant injury. The immediately adjacent spiral ganglion cells would be directly injured or exposed to ongoing inflammatory and fibrotic processes.
Cochlear and vestibular neuroepithelial damage may occur secondary to perilymphatic and endolymphatic flow and composition alterations as well as by hydraulic forces.
Anatomic Region of Injury | Mechanism |
Lateral wall/spiral ligament | High cochleostomy Inappropriate angle of insertion Electrode friction/design Outer wall forces |
Basilar membrane | High cochleostomy Inappropriate angle of insertion Outer wall forces Electrode size |
Osseus spiral lamina | Electrode pressure on medial wall Medial movement after basilar membrane perforation |
Modiolar wall | Electrode pressure of perimodiolar designs Direct insertion trauma Chronic electrode pressure |
Neuroepithelium | Hydraulic forces Adjacent structural violations |
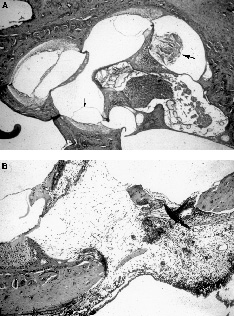
Mechanisms of Trauma
The surgical cochleostomy represents the first step in the traumatic process of cochlear implantation. Direct injury to the spiral ligament, basilar membrane, and the osseus spiral lamina may occur with a cochleostomy that is made in a superior position closer to the oval window. Blood and bone dust created by the drilling process might enter the cochlea, initiating an irritative or inflammatory process leading to fibrosis and ossification. Fibrosis and neo-ossification were found in nearly every control and test guinea pig cochlea, adjacent to the cochleostomy and more distally in the scala tympani, during a lubricant safety study43 (Fig. 9–3). Bone dust introduced during cochleostomy can be pushed further into the scala by the electrode insertion.
Electrode introduction and insertion occurs after cochleostomy. An electrode will directly elevate or penetrate the basilar membrane if inserted at an inappropriate angle. Damage from this type of trauma is seen as either focal or diffuse elevation, focal penetration, or diffuse disruption (Fig. 9–4). An electrode that penetrates focally will occupy the scala vestibuli or scala media in the upper cochlea. Diffuse disruption represents the most severe form of basilar membrane trauma. All electrode designs have been found to potentially disturb or penetrate the basilar membrane to varying degrees.