Introduction
Dry eye disease (DED) is a multifactorial disease for which the exact pathophysiological mechanism remains unknown. Nevertheless, a combination of a compromised tear film, ocular surface inflammation, and neurosensory abnormalities in the presence of signs and/or symptoms is considered the main pillars of DED. , This chapter aims to address evaluation of neurosensory abnormalities through assessment of nerve health, as well as the impact the other pillars, the tear film and ocular surface inflammation, have on the nerves and vice versa.
Nerves of the ocular surface have both a sensory function and a physiological function. The first function involves the nociceptive, or sensory, neural pathway system of the body. Our body is in direct contact with the surrounding environment, and the nociceptive neural pathway system provides awareness of the surrounding environment, as well as internal stimuli to the central nervous system, so that if needed, action can be initiated to prevent harm by and/or protect against noxious stimuli that could cause tissue damage. , However, ocular surface nerves do more than provide sensation. They have a large impact on the tear film, and nerve health can impact both the progression of DED and DED treatment. This chapter will discuss the role that the nerves play in DED and how we can assess these nerves clinically.
Anatomy of the Nerves
To fully understand any aspect that nerves play in DED (sensations, pain, corneal reflex actions, or neuroimmune crosstalk), an understanding of the anatomy of the corneal nerves, their pathways to the brain, and efferent nerves to target organs is necessary. The afferent nerves that innervate the cornea and ocular surface are pseudounipolar neurons, which have their cell bodies within the trigeminal ganglion. The axons innervating the ocular surface travel via the nasociliary branch of the ophthalmic division of the trigeminal nerve (cranial nerve V 1 ). While conjunctival innervation does contribute to corneal innervation at the limbus and far periphery, the cornea has its own very dense innervation network. Nerve trunks enter the corneal stroma in a radial fashion before branching to form a midstromal plexus, which is most dense in the corneal periphery. In addition to the midstromal plexus, branches from the limbal stromal plexus and collaterals of the stromal nerve trunks contribute to a subbasal plexus. , After penetrating Bowman’s membrane, nerve bundle branches and the nerve fibers ultimately converge to form the subbasal plexus. As the branches innervate the corneal epithelium, they form terminals throughout all corneal epithelial cell layers ( Fig. 3.1 ).

The nociceptors of the ocular surface are most often characterized by the stimulus or stimuli to which they respond. The three major types of receptors have been identified as mechanical, thermal, and polymodal. Mechanical receptors are stimulated by mechanical stimuli only, especially stimuli with motion. However, it has been shown that the most prominent nociceptors are polymodal receptors, which respond to a variety of stimuli. They respond strongly to changes/initiation of mechanical stimuli and to noxious, but not necessarily painful, mechanical stimuli. These receptors also respond to heat stimuli above approximately 39°C, noxious cold stimuli, and changes to pH levels. Unlike mechanical and polymodal receptors, studies have shown that cold receptors are constantly active even without stimulation. , These receptors also show increased activity to cold stimuli, decreased activity to warm stimuli, and increased activity to acidic stimuli, hypertonic stimuli, and certain chemical stimuli. , Due to the ongoing activity of cold receptors and their increased activity with small reductions in ocular surface temperature, which could be experienced between blinks, it is theorized that these receptors are important in basal tearing. Evidence in mice supports that the transient receptor potential cation channel subfamily M member 8 (TRPM8) channel, which is associated with cold receptors, specifically is important for this basal tearing.
The first-order neurons of the ocular surface have a second axon branch reaching toward the brainstem. This branch of the axon enters the brainstem at the level of the pons and terminates to form a synapse with the second-order neuron cell bodies within the trigeminal nucleus. The trigeminal nucleus is divided, from rostral to caudal, into the oralis (V o ), the interpolaris (V i ), and the caudalis (V c ). The corneal afferent terminations exist within (1) the caudal region of V i and the rostral region of V c (the V i /V c complex) and (2) the caudal region of V c and rostral region of the dorsal horn of the first cervical vertebrae (the V c /C1 complex). Here we will focus on the responses within these complexes that are most closely related to DED. When comparing these two regions, one can broadly state that tearing can be altered by V i /V c complex stimulation and inhibition, which is not the case for the V c /C1 complex. Further, the V c /C1 complex does not respond to drying or wetting of the cornea, while some neurons within the V i /V c complex do respond to corneal dryness. These neurons, which are sometimes called type II V i /V c complex neurons, also show reduced activity when the cornea is moistened with artificial tears. , These type II neurons also respond to hyperosmolarity and cold temperatures. Preclinical studies suggest that nerves in the V i /V c complex and V c /C1 complex become sensitized when the cornea is exposed to hyperosmotic conditions in DED. Responses in the V c /C1 complex are consistently inhibited by analgesics, which suggests that this area is involved in pain sensation; however, this response to analgesics is not present within the V i /V c complex. , ,
Processing of the information transmitted to these complexes contributes to several reflex responses: (1) blinking; (2) corneal-lacrimal reflex tearing; and (3) goblet cell secretion. Studies investigating the contribution of the trigeminal nucleus processing to blinking have shown that the V i /V c complex neurons initiate reflex blinking and control their amplitude. Dry eye conditions enhance the typical reflex blinks caused by hypertonic solution. In addition, chemical suppression of the V i /V c complex neurons or the V c /C1 complex neurons reduced the blink reflex. Contribution to the second and third reflex responses, corneal-lacrimal reflex tearing and goblet cell secretion, are supported by the neuron projections from the trigeminal nucleus. Projections from both complexes of the trigeminal nucleus travel to the superior salivatory and lacrimal nucleus, which is known to project to the pterygopalatine ganglion and then the tear producing glands. Compared to the V c /C1 complex, there does appear to be a stronger association between the superior salivatory and lacrimal nucleus and the V i /V c complex, which responds to drying/wetting of the cornea, hyperosmolarity, and cold temperatures. , Both complexes also project to the thalamus, which is often a relay station for further processing within the brain, such as the processing of sensory information within the primary somatosensory cortex. However, the thalamus appears to have a stronger association with the V c /C1 complex, which responds to analgesics in a way expected for neurons involved in pain processing. , While the presence of projections to and from the superior salivatory and lacrimal nucleus explains the contribution of the trigeminal nucleus to the reflex responses of corneal-lacrimal reflex tearing and goblet cell secretion, the apparent organization of the information carried specifically from the V i /V c complex to the superior salivatory and lacrimal nucleus suggests that the V i /V C complex’s contribution is of grave importance to these reflexes.
Nerves from the superior salivatory and lacrimal nucleus follow the greater petrosal branch of the facial nerve to synapse in the pterygopalatine ganglion. The postganglionic nerves then join with the zygomatic branch of the trigeminal nerve, and terminate at the tear producing glands, including the lacrimal gland. In addition to parasympathetic innervation from the pterygopalatine ganglion, the lacrimal gland also has sympathetic and sensory innervation from the superior cervical and trigeminal ganglions, respectively. However, the parasympathetic system appears to be most closely associated with secretion and tearing. ,
Like the lacrimal gland, the goblet cells also have a diverse innervation, although it should be noted that not all goblet cell clusters are associated with nerves. , , Like the lacrimal gland, current evidence suggests that the parasympathetic innervation affects secretion. While the response of the lacrimal gland to ocular surface stimuli is well known, evidence suggests that goblet cells also secrete their mucins in response to ocular surface stimulation. The proximal association of nerves with the Meibomian glands , , and accessory lacrimal glands , is also well established; however, there is limited evidence to support the hypothesis that these nerves influence the production or secretion of tear contributing substances, although recent evidence demonstrates that neurostimulation does result in release of meibum.
The presence of a variety of receptors at the ocular surface and the organization of information at the level of the trigeminal nucleus suggest that this pathway may also be linked to tear production even at times of homeostasis. While the importance of nerves and their pathways in the pathogenesis of DED is still fully being discovered, it is clear that understanding and assessing nerve health in DED evaluations is critical.
Manifestations of Compromised Nerve Health
The nociceptive neurons are composed of thinly myelinated Aα-fibers (proprioception), Aβ-fibers (mechanoreception), AΩ-fibers (nociception), and unmyelinated C-fibers (polymodal, including nociception). , The nerve terminals of the peripheral tissues, such as the eye, contain high-threshold receptors, ergo nociceptors, responsible for depolarizing cellular ion channels, and initiating communication of the presence of a noxious stimulus from the peripheral to the central nervous system through the nociceptive pathways. In other words, a stimulus, whether thermal, mechanical, or chemical, that exceeds the threshold of nociceptors, is required to generate a noxious stimulus message and reach the brain. ,
When pain is experienced from a noxious stimulus, it is considered nociceptive. There are several classifications for pain, such as acute (when the pain lasts 3 months or less) or chronic (when the pain lasts greater than 3 months). There are also different types of pain: , ,
- (a)
Nociceptive pain : physiologic response to direct noxious stimuli, albeit external (i.e., mechanical, thermal and chemical) or internal (i.e., tissue ischemia or cellular hypoxia), that activates high-threshold nociceptor neurons. , ,
- (b)
Inflammatory pain : pain hypersensitivity due to detection of active inflammation in peripheral tissue by nociceptors or cytokine receptors of the peripheral nervous system. Local inflammation often triggers both neuronal depolarization and other immune cell depolarization, generating a self-reinforced stimulus called neurogenic inflammation. ,
- (c)
Neuropathic pain : maladaptive response, caused by a neuronal lesion or disease that initially affects either the peripheral or central nervous system, of the somatosensory system that generates a painful response in the absence of a stimulus or an amplified response to innocuous and noxious stimuli. , ,
- (d)
Dysfunctional pain/noninflammatory/nonneuropathic pain : amplification of the nociceptive signaling in the absence of inflammation or neural lesions. ,
It is important to note that these classifications can sometimes overlap, meaning a chronic pain can have an inflammatory component or a neuropathic component, or both.
Both environmental stimuli and tear film hyperosmolarity can trigger the corneal nociceptors resulting in DED symptoms, such as burning sensation, foreign body sensation, light sensitivity, dryness, and ocular pain. The activated nociceptors also generate reflex responses, such as corneal-lacrimal reflex tearing, goblet cell secretion, and blink reflex. These sensations and reflexes can be augmented in the presence of inflammation often associated with DED. Corneal inflammation can cause a nociceptive hypersensitivity enhancing the nociceptive pathways and exacerbating the noxious stimuli and even innocuous stimuli, such as wind, light (photoallodynia), and palpebral mechanical contact. , , Thus, inflammation can cause changes to the ocular surface nerves, which in turn can change the sensations and reflexes experienced by a patient; however, changes to peripheral nerves can also initiate or perpetuate inflammation. Corneal nerve damage, which has been shown in DED, activates inflammation to aid healing and repair. The inflamed tissue area promotes a heightened sensitivity that can be activated by low-threshold innocuous stimuli and noxious stimuli that are both prolonged and exaggerated. It is hypothesized that these continuous stimuli, either from untreated or persistent inflammation, can cause neural sensitization and neuroplasticity. If the stimuli continue within the peripheral nerves and are not addressed, this can result in central sensitization. This can then result in the development of neuropathic pain, where DED symptoms persist despite appropriate treatment and resolution of ocular surface inflammation and tear film stability. , , Importantly, both neuropathic and chronic pain are complex and poorly understood neurological phenomena, specifically when involving corneal nerves. , However, there is a growing consensus within the research and clinical community that neuropathic pain is likely the explanation for patients who present with DED symptoms, but lack signs of DED or those with symptoms that are out of proportion to signs. Nevertheless, neuropathic pain can also occur with severe surface disease such as in early stages of neurotrophic keratopathy. Both neurogenic inflammation and neuropathic pain are examples of the neuroimmune crosstalk that can occur in the cornea, which is discussed next.
Neuroimmune Crosstalk
An additional key aspect of nerve health involves the impact of inflammation associated with DED on these nerves and vice versa. Corneal inflammation and nerve dysfunction are two important pillars in the pathophysiology of DED. , Evidence for inflammation in patients has been demonstrated in various types of DED, i.e., evaporative, MGD or aqueous-deficient, and in different stages of severity. It has been well-established that desiccation leads to a proinflammatory microenvironment, thus stimulating dendritic cells (DCs), which mature and migrate to draining lymph nodes, subsequently activating T cells that further amplify the inflammatory cascade. , This process is commonly referred to as the “inflammatory vicious cycle” in DED, affecting the tear film stability, tear osmolarity, ocular surface cytokine activation, cell cycle function (apoptosis), and corneal nerves. ,
Imaging studies have shown that increased density of corneal DCs are correlated with clinical parameters of DED, lacrimal gland lymphocytic infiltration, and increased tear film cytokines levels. , Thus, corneal DCs have been proposed as an imaging biomarker surrogate for treatment response in DED. In addition, greater corneal DC density has shown a correlation with decreased corneal nerve density. , Corneal nerve alterations have been reported previously in patients with DED, such as decreased density and increased tortuosity, branching and beading, , , and the close association of corneal DCs and nerves suggest a neuroimmune crosstalk that may occur in various ocular surface diseases, including DED.
The exact mechanism of how corneal nerves can affect local immune responses in different diseases is not fully understood. Nevertheless, there is growing evidence of the critical role the peripheral nervous system plays in maintaining corneal immune privilege and immune homeostasis. Antigen-presenting cells, including DCs and macrophages, as well as T-lymphocytes can directly activate neurons, Schwann cells, and glial cells via cytokines and chemokines, such as interleukin (IL)-1β, tumor necrosis factor (TNF)-α, bradykinin, and nerve growth factor. , , Conversely, neuropeptides can modulate or suppress proinflammatory cytokine secretion of leukocytes. Thus, dysfunction of corneal nerves can trigger a maladaptive proinflammatory innate immune response, called neurogenic inflammation. , ,
Preclinical studies have demonstrated that sensory nerve denervation results in a significant increase in activation and migration of DCs into the cornea, increased expression of vascular adhesion molecules followed by an increase of proinflammatory cytokine levels (IL-1β, IL-6, and IL-17), corneal angiogenesis, and lymphangiogenesis. In clinical studies, there was an inverse correlation of corneal nerve density and DC density on in vivo confocal microscopy (IVCM) in patients with infectious keratitis, neurotrophic keratitis, and DED. , , , , Moreover, it has been shown that corneal nerves regenerate 6 months after infection and resolution of inflammation, although they do not fully recover to normal levels. Furthermore, the literature suggests that chronic peripheral nerve injury and inflammation that occur in DED can drive peripheral sensitization of the nociceptive pathways. , This phenomenon may contribute to many of the DED symptoms, such as photophobia (photoallodynia), burning, foreign body sensation, and pain. , , When peripheral sensitization remains present for long periods of time, central sensitization can occur.
Taken together, recent studies suggest that dysfunction or damage to corneal nerves result in an immediate disruption of immune homeostasis and may be an underlying intrinsic part of the pathogenesis of many ocular surface diseases.
Clinical Assessment of Corneal Nerve Health
An assessment of nerve health in DED should involve two major aspects: anatomy and function.
Anatomy
While nerves beyond the ocular surface are out of reach for anatomical assessment, anatomical assessment of corneal nerves can be conducted via IVCM imaging of the subbasal nerve plexus. , IVCM is a noninvasive tool that allows for real-time, layer-by-layer, and near-histological resolution cellular imaging of the cornea. The main principles consist of utilizing a white or laser light through a pinhole aperture, the light is focused point-by-point and the scattered light is deflected through a second confocal aperture. , The most common IVCM devices use either a slit-scanning or a laser scanning design. The latter has the advantage of scanning multiple points at a faster rate and higher axial resolution with en face visualization and subsequent volumetric imaging of corneal structures. ,
The specifications vary among the different devices commercially available: lateral resolution 1–2 μm, axial resolution 4–27 μm, and field of view 300 × 300 μm or 400 × 400 μm. , The laser scanning device uses a 670 nm diode laser that generates a 1 μm lateral resolution; however, it requires contact and minor corneal applanation during image acquisition. Thus, utilization of anesthetic eye drops, a disposable contact cap, and gel are recommended.
The different indices of refraction of the various corneal structures allow for visualization of subbasal nerve plexus, epithelial cells, keratocytes, endothelial cells, and immune cells, i.e., DCs. , , Quantification of corneal subbasal nerves through IVCM images have been well-established in the literature in DED. Their characteristics and clinical application will be discussed in the following sections ( Fig. 3.2 ).

One common quantitative nerve assessment performed on these IVCM images is total nerve density, which is calculated by summing the length of all the nerves within one image and dividing the sum by the area of the image. Studies have reported group mean total nerve densities of 15,956 μm/mm 2 to 24,461 μm/mm 2 for control groups , , and 9,426 μm/mm 2 to 18,300 μm/mm 2 in DED. , , While there is some overlap in these ranges, most studies report reduced total nerve fiber density in DED subjects compared to controls. , , , , , Historically, nerve counts were used, in which the number of nerves were counted within an image and divided by the image area. However, this measure is not commonly used today.
Another anatomical measure assessed via IVCM imaging is nerve tortuosity. This measure is typically graded on a scale of 0–4 where 0 is no tortuosity and 4 is extensive tortuosity. The average normal value for tortuosity is reported as 1.1 grade (grade range 0–4). , , In DED, increased tortuosity is reported consistently in numerous studies. Previous studies reported grade 2.63–3.18 nerve tortuosity in Sjögren’s-related dry eye and grade 2.30–3.01 in non-Sjögren’s dry eye, which was significantly different when compared to controls.
A third measure that can be made via IVCM is beading of nerves. Subbasal nerve beading frequency varied from 90 to 198 beads/mm in healthy individuals. , , Many studies report this is increased in DED. Reported values for DED include 332–387/mm in Sjögren’s-related DED and 186–323/mm and in non-Sjögren’s-related DED. , ,
A new interest for IVCM assessment is the presence of microneuromas. Microneuromas are known to be the source of ectopic spontaneous excitations in sensory fibers in patients with postsurgical pain. In 2015, Aggarwal et al. first described microneuromas as stumps of severed nerves and abrupt endings of nerve fibers on IVCM and reported their presence in patients with photoallodynia. Recently, the presence of microneuromas has been suggested as a biomarker for neuropathic pain. These microneuromas were found to be more numerous in patients with peripheral pain compared to those with centralized pain, which suggests that the anatomical appearance of nerves supports the symptomology of patients and the importance of these microneuromas in the pathophysiology of the neuropathic corneal pain. , As we continue to investigate microneuromas, it is important to ensure thorough corneal mapping, given that microneuromas are not equality distributed throughout the cornea, and that investigations utilize appropriately trained and experienced observes, since research has noted that other corneal structures, such as entry points of stromal nerve fiber bundles through the Bowman’s membrane or immune cells associated with nerves can be mistaken as microneuromas ( Table 3.1 ). ,
Study | Device Used | Sample | Nerve Density | Beading | Tortuosity | Correlations |
---|---|---|---|---|---|---|
Labbe et al. | Heidelberg Retinal Tomograph/Rostock Cornea Module | 10 control eyes 12 DED eyes |
|
|
| |
Levy et al. | Heidelberg Retinal Tomograph/Rostock Cornea Module | 15 controls 30 SS DED treated with cyclosporine |
|
|
| |
Tepelus et al. | Heidelberg Retinal Tomograph/Rostock Cornea Module | 24 eyes with NSS DED 44 eyes with SS DED 10 control eyes |
|
|
| |
Tepelus et al. | Heidelberg Retinal Tomograph/Rostock Cornea Module | 30 eyes of GVHD 20 eyes of DED 16 control eyes |
|
| ||
Kheirkhah et al. | Heidelberg Retinal Tomograph/Rostock Cornea Module | 40 eyes with DED 13 control eyes |
| |||
John et al. | Heidelberg Retinal Tomograph/Rostock Cornea Module | 8 DED placebo treated 9 DED treated with amniotic membrane |
| |||
Kheirkhah et al. | Heidelberg Retinal Tomograph/Rostock Cornea Module | 33 DED with GVHD 21 DED without GVHD |
|
| ||
Labbe et al. | Heidelberg Retinal Tomograph/Rostock Cornea Module | 14 control pts 43 NSS DED |
|
|
| |
Benitez del Castillo et al. | Confoscan | 11 controls <60yoa 10 controls ≥60yoa 11 SS DED 10 NSS DED |
|
|
|
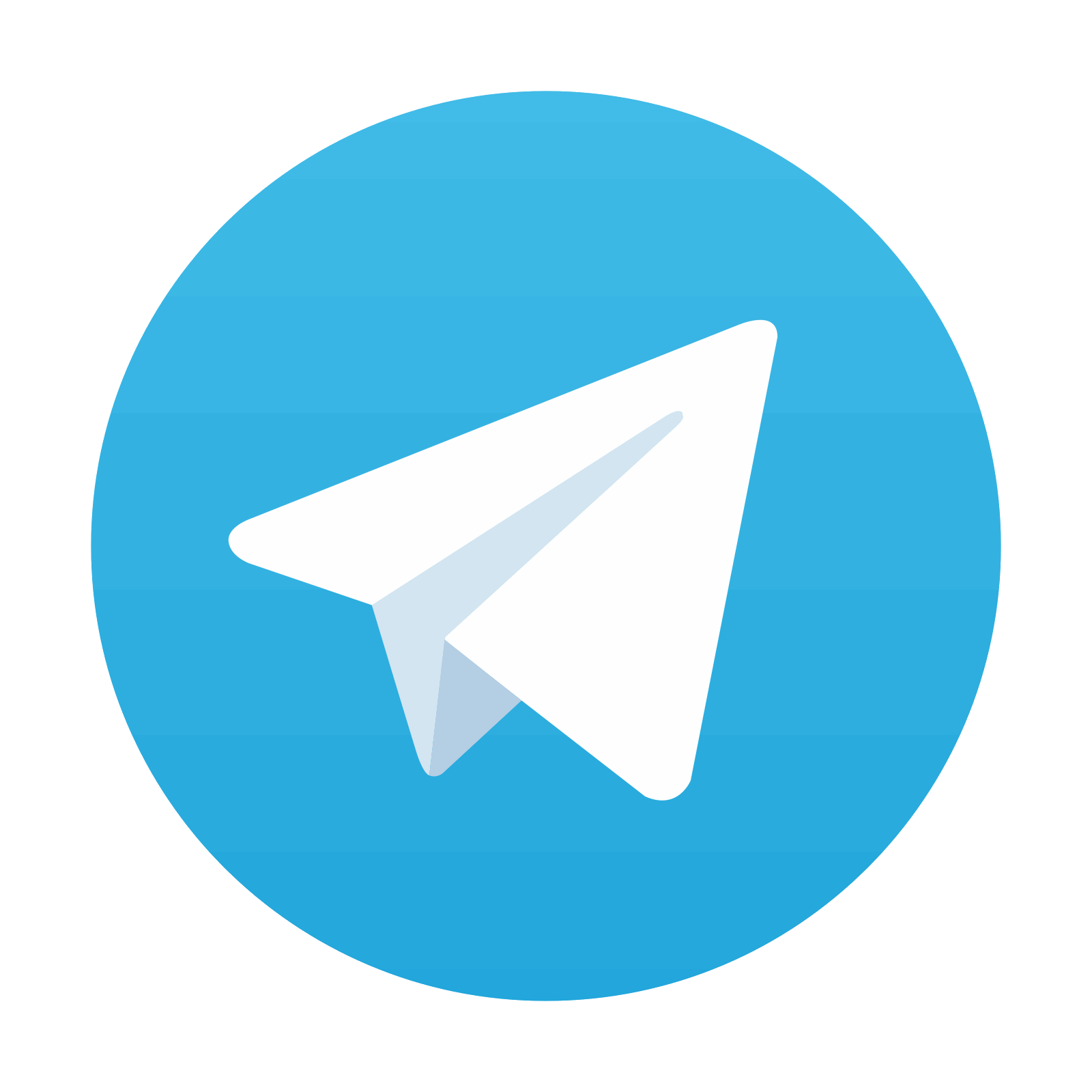
Stay updated, free articles. Join our Telegram channel

Full access? Get Clinical Tree
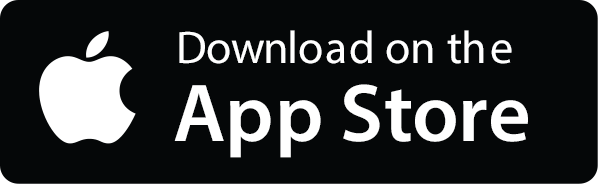
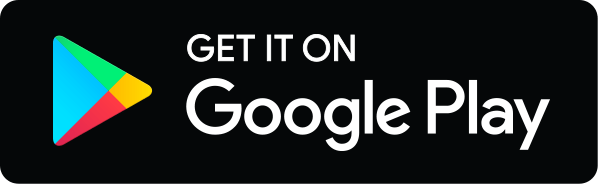
