Cerebellopontine Angle Tumors
Matthew L. Bush
Bradley D. Welling
INTRODUCTION
The cerebellopontine angle (CPA) is a complex and wellguarded anatomic region of the cranial base that contains an intricate interface of the central and peripheral nervous systems. Lesions occurring within this area are diverse in etiology and may lead to significant symptoms. The otolaryngologist frequently encounters pathology of the CPA that may present a diagnostic and therapeutic dilemma. A thorough knowledge of the anatomy of the region along with a clear understanding of diagnostic and therapeutic options for tumors in this region is vital for successful management. A variety of lesions may occur in this location and the differential diagnosis is discussed.
History
Tumors occurring within the CPA were identified as early as the late 1700s (1); however, little was known about the anatomy of this region and even less was known about the physiology of the nervous system. During the 1800s, Sir Charles Bell, a surgeon and skilled anatomist, carefully documented the intricate anatomy of the brainstem and cranial nerves, and he is credited with publication of the first clinical case of a CPA tumor in 1833 (2), which was identified as a vestibular schwannoma. The first successful removal of CPA vestibular schwannoma was performed by Sir Charles Ballance in 1894; however, surgical intervention for CPA tumors carried a mortality rate near 80% (3).
Significant advances in diagnostic evaluation of brain tumors occurred during the late 1800s and early 1900s, which allowed further correlation of clinical symptoms with specific lesions (4). Subsequently, the term cerebellopontine angle tumor was introduced in 1902 (5) to describe posterior fossa tumors. With the aid of radiographic imaging and astute clinical observation, Harvey Cushing carefully described the CPA syndrome in his 1917 monograph, Tumors of the Nervus Acusticus and the Syndrome of the Cerebellopontine Angle (6). Furthermore, Cushing advocated surgical intervention for CPA tumors to prevent brainstem compression and death via a suboccipital craniotomy approach to facilitate subtotal tumor removal. Mortality rates for surgical intervention dropped below 30%. Walter Dandy (7) advocated complete resection of CPA tumors through a modified suboccipital approach that improved exposure through resection of the lateral third of the cerebellum.
The latter half of the 20th century led to further advances in cranial base surgery including improved imaging modalities, high-speed surgical drills, and the microscope. The translabyrinthine approach to the CPA, although previously described (8,9), was popularized by William House, who reported improved facial nerve function, tumor exposure, and reduced morbidity and mortality. In an attempt to preserve hearing, House also advocated and demonstrated the utility of the middle fossa approach in small intracanalicular tumors (10,11). Although surgical approaches to the CPA have changed little over the past several decades, technologic advances in microsurgery, stereotactic radiation, diagnostic evaluation, and perioperative care have continued to reduce morbidity and mortality.
Anatomy
The CPA is a subarachnoid space filled with cerebrospinal fluid (CSF) and contains tortuous brainstem vessels and cranial nerves (Fig. 159.1). This inverted triangular-shaped space within the lateral posterior fossa is bound superiorly by the cerebellar tentorium and inferiorly by the cerebellar tonsil. The ipsilateral cerebellar hemisphere and the brainstem form the posterior and medial borders, respectively. The posterior face of the petrous temporal bone, with its dural reflection, forms the anterior border. The CPA cistern is the space between the pons, medulla oblongata, and the
cerebellum medially and the temporal bone anteriorly. It contains blood vessels, cranial nerves, and the cerebellum flocculus and choroid plexus, which are surrounded by CSF. This cistern extends into the medial aspect of the internal auditory canal (IAC) of the petrous bone, which is known as the porus acusticus. Within the superior portion of the cistern, the trigeminal nerve travels from the lateral pons to the Meckel cave. The seventh and eighth cranial nerves exit the brainstem at the lateral pontomedullary junction adjacent to the foramen of Luschka and travel prominently within the CPA and then enter the porus acusticus. Inferiorly, the ninth, tenth, and eleventh cranial nerves extend from the medulla across the inferior lateral aspect of the CPA and enter the pars nervosa of the jugular foramen. The basilar artery lies near the midline anterior to the brainstem and gives off the anterior inferior cerebellar artery (AICA), which takes a variable course through the CPA and can loop into the porus acusticus. Adjacent to the IAC, the AICA forms a branch, the labyrinthine artery, which travels laterally in the canal to supply the cochlea and labyrinth.
cerebellum medially and the temporal bone anteriorly. It contains blood vessels, cranial nerves, and the cerebellum flocculus and choroid plexus, which are surrounded by CSF. This cistern extends into the medial aspect of the internal auditory canal (IAC) of the petrous bone, which is known as the porus acusticus. Within the superior portion of the cistern, the trigeminal nerve travels from the lateral pons to the Meckel cave. The seventh and eighth cranial nerves exit the brainstem at the lateral pontomedullary junction adjacent to the foramen of Luschka and travel prominently within the CPA and then enter the porus acusticus. Inferiorly, the ninth, tenth, and eleventh cranial nerves extend from the medulla across the inferior lateral aspect of the CPA and enter the pars nervosa of the jugular foramen. The basilar artery lies near the midline anterior to the brainstem and gives off the anterior inferior cerebellar artery (AICA), which takes a variable course through the CPA and can loop into the porus acusticus. Adjacent to the IAC, the AICA forms a branch, the labyrinthine artery, which travels laterally in the canal to supply the cochlea and labyrinth.
Within the cistern, the seventh and eighth cranial nerves lack an epineurium and are covered by pia mater and glial tissue. The facial nerve lies inferior and then anterior to
the vestibulocochlear nerve complex within the cistern but rotates 90 degrees as it travels within the IAC (Fig. 159.1C). Within the canal the eighth nerve divides into its main branches, and the superior and inferior vestibular nerves fill the posterior half of the canal. The cochlear nerve resides within the anteroinferior quadrant of the canal, with the facial nerve occupying the quadrant immediately superior to the cochlear nerve and anterior to the superior vestibular nerve. The lateral aspect of the IAC, known as the fundus, possesses bony partitions of the seventh and eighth cranial nerves. The vertical crest, also known as the Bill bar, separates the facial nerve from the superior vestibular nerve, while the transverse crest separates these two superiorly located nerves from the cochlear nerve and inferior vestibular nerve.
the vestibulocochlear nerve complex within the cistern but rotates 90 degrees as it travels within the IAC (Fig. 159.1C). Within the canal the eighth nerve divides into its main branches, and the superior and inferior vestibular nerves fill the posterior half of the canal. The cochlear nerve resides within the anteroinferior quadrant of the canal, with the facial nerve occupying the quadrant immediately superior to the cochlear nerve and anterior to the superior vestibular nerve. The lateral aspect of the IAC, known as the fundus, possesses bony partitions of the seventh and eighth cranial nerves. The vertical crest, also known as the Bill bar, separates the facial nerve from the superior vestibular nerve, while the transverse crest separates these two superiorly located nerves from the cochlear nerve and inferior vestibular nerve.
EXTRAAXIAL LESIONS
The differential diagnosis of CPA lesions can be categorized in multiple ways based on site of origin, imaging characteristics, or histopathology. Bonneville et al. (12,13) outlined a differential diagnosis strategy for CPA tumors based on site of origin. Under this classification, lesions are categorized as extraaxial, intraaxial, or skull base in origin. Extraaxial lesions are distinct from the brain parenchyma, displace surrounding structures, and may be neural, dural, vascular, inflammatory, or infectious in nature.
Vestibular Schwannoma
Epidemiology
Vestibular schwannomas are encapsulated benign tumors that arise from the Schwann cells of the vestibular nerve. Historically, these tumors have been referred to as acoustic neuromas; however, vestibular schwannoma represents a more accurate name. Vestibular schwannomas account for approximately 6% of all intracranial tumors and 85% of all CPA tumors (14). The incidence rate is variable globally but has been increasing over the past few decades and is reported to be 1.9 tumors per 100,000 people per year (15).
Histopathology
Vestibular schwannomas may occur on either the superior or inferior nerve; however, they more commonly originate from the inferior vestibular nerve (16,17). A commonly held misconception is that tumors most often develop at the glial-Schwann cell junction known as the Obersteiner-Redlich zone. Xenellis and Linthicum (18) point out that this is a much less common site of origin than laterally near Scarpa ganglion. Tumors typically grow slowly by expanding the porus and extending into the CPA (19). Grossly, the tumors appear yellow to gray; however, larger or cystic tumors may vary in appearance due to hemorrhage or necrosis. Approximately 5% to 20% of vestibular schwannomas are cystic in nature and tend to have poorer outcomes for facial nerve preservation (20,21). Vestibular schwannomas typically slowly expand within the IAC where they invade the vestibular nerves and eventually compress the cochlear and facial nerves, as well as the labyrinthine artery. Without intervention, this eventually leads to cochlear and vestibular dysfunction. The facial nerve is exceptionally resilient to dysfunction, in spite of compression and thinning of the nerve; nevertheless, facial weakness can be present in large tumors. Extension into the CPA can lead to compression of the cerebellum and brainstem and can cause cranial neuropathy, hydrocephalus, and even death.
Histologic examination of vestibular schwannomas reveals two discrete cell arrangements. Regions of compact spindle cells with bland cytoplasm and lack of nuclear atypia along with whorls of palisading nuclei aligned in rows (Verocay bodies) are referred to as Antoni type A arrangement. Antoni type B, however, possesses a less dense and more loosely arranged cellular histology. Areas of necrosis and fibrosis may also be seen within these tumors. Due to the neural crest cell origin of Schwann cells, schwannoma cells display strong S100 immunohistochemical staining.
Molecular Biology
Vestibular schwannomas most commonly occur sporadically; however, they can also occur as part of the genetic syndrome, neurofibromatosis type 2 (NF2). This highly penetrant autosomal dominant disorder with variable expressivity presents with multiple nervous system tumors. Diagnostic criteria have been defined for NF2 (22). The presence of bilateral vestibular schwannomas establishes a diagnosis of NF2. However, NF2 patients may also develop multiple spinal and cranial nerve schwannomas, meningiomas, and ependymomas. NF2 is caused by a germ-line mutation within a tumor suppressor gene, known as NF2, located on chromosome 22q12. This gene, identified by both Trofatter (23) and Rouleau (24), was found to encode a cytoskeletal protein known as merlin or schwannomin that modulates cellular adhesion, proliferation, and motility (25). A variety of mutations have been identified within the NF2 gene, and disease severity appears to be at least somewhat related to the type of mutation present (26), although marked phenotypic variation can occurs within NF2 families.
The absence of merlin results in alterations in cellular signaling and promotes cellular proliferation and tumorigenesis. Merlin typically interacts with tyrosine kinase receptors, such as the ErbB receptor family (27,28,29,30,31), to prevent growth; therefore, these receptors have been identified as potential targets for inhibition of proliferation in merlindeficient vestibular schwannoma cells (32,33,34,35,36). Merlin also prevents the pro-growth effects of the AKT/PI3 kinase signaling pathway, and AKT activation has been demonstrated in vestibular schwannomas and may be another potential target for vestibular schwannoma drug development (37,38). Vascular endothelial growth factor (VEGF) and associated receptors have been implicated in vestibular schwannoma tumorigenesis, and NF2 patients have undergone clinical trials to inhibit tumor growth with anti-VEGF medications (39,40,41,42). Although translational research has advanced our knowledge of the mechanisms of tumor growth and has provided potential treatment targets, effective medical treatments have not yet been clearly demonstrated.
Clinical Presentation
Vestibular schwannomas, when small or when they arise in the CPA rather than the confines of the IAC, may be asymptomatic. More are being diagnosed incidentally on imaging studies performed for separate indications. The majority of tumors, however, present with unilateral auditory or vestibular dysfunction. Approximately 75% of patients with vestibular schwannomas experience unilateral tinnitus as an early symptom (43). This may be severe enough to affect quality of life (44,45). Unilateral hearing loss develops in approximately 95% of vestibular schwannoma patients (46) and typically is progressive in nature (47,48,49). Only 5% of patients with vestibular schwannomas present with sudden sensorineural hearing loss; however, 20% to 30% of vestibular schwannoma patients may experience a sudden deterioration in their hearing during their clinical course (50,51). This asymmetric hearing loss may respond to steroid treatment; however, further evaluation is indicated to rule out retrocochlear pathology. Vestibular schwannomas gradually ablate the function of the ipsilateral vestibular system; thus patients may experience disequilibrium, although this is the presenting chief complaint in only 10% of patients (52). Tumor extension into the labyrinth may result in episodic vertigo, similar to Meniere disease. A family history of vestibular schwannomas or NF2 should be determined. A thorough neurotologic examination is warranted for any patient with suspected cranial base pathology and should include a microscopic otologic examination, evaluation of cranial nerve function, and cerebellar examination. Decreased sensation of the ear canal and conchal bowl is referred to as Hitselberger sign and is due to dysfunction of the sensory component of the facial nerve by an expanding vestibular schwannoma. Expansion of the tumor within the CPA may lead to brainstem compression and cranial nerve dysfunction and present with diplopia, facial hypesthesia, facial paralysis, dysphagia, hoarseness, hydrocephalus, and, potentially, death (45,52).
Diagnostic Evaluation
Audiologic Behavioral and Electrophysiologic Evaluation
Audiometric evaluation of hearing loss is fundamental to the evaluation of patients with vestibular schwannomas and includes, at a minimum, pure-tone audiometry and word discrimination evaluation. Patients with vestibular schwannomas typically present with unilateral sensorineural hearing loss with disproportionate asymmetric word recognition. Even when hearing loss is mild, vestibular schwannoma patients typically experience a significant degree of word recognition dysfunction. In order to report outcomes consistently, auditory function should be documented using the American Academy of Otolaryngology— Head and Neck Surgery classification (53). Acoustic reflex threshold and acoustic reflex decay have been used as a screening tool for retrocochlear pathology, but these tests are not commonly employed currently. Auditory brainstem response (ABR) may be useful for electrophysiologic assessment of auditory function. An interaural wave
V latency difference of greater than 0.2 ms, or prolonged interpeak latencies greater than 4.4 ms for I-V, 2.3 ms for I-III, or 2.1 ms for III-V raise the suspicion of a vestibular schwannoma. ABR usage is limited by the fact that a reliable waveform is absent in patients with moderately severe hearing loss. The sensitivity of ABR is greater than 90% in medium and large tumors; however, the sensitivity ranges between 60% and 80% in tumors less than 1 cm (54,55,56). The sensitivity of ABR has been increased to 95% with the use of a stacked ABR technique as reported by Don et al. (57).
V latency difference of greater than 0.2 ms, or prolonged interpeak latencies greater than 4.4 ms for I-V, 2.3 ms for I-III, or 2.1 ms for III-V raise the suspicion of a vestibular schwannoma. ABR usage is limited by the fact that a reliable waveform is absent in patients with moderately severe hearing loss. The sensitivity of ABR is greater than 90% in medium and large tumors; however, the sensitivity ranges between 60% and 80% in tumors less than 1 cm (54,55,56). The sensitivity of ABR has been increased to 95% with the use of a stacked ABR technique as reported by Don et al. (57).
Vestibular Assessment
Progression of vestibular schwannomas leads to eventual loss of ipsilateral vestibular function, which is most often accompanied by central compensation. Videonystagmography (VNG) may document the degree of central and peripheral vestibular dysfunction. Caloric testing is the most useful component of VNG testing in vestibular schwannoma patients, and it may identify a unilateral vestibular weakness in tumors that have affected or originate from the superior vestibular nerve. A small schwannoma of the inferior vestibular nerve may have a normal caloric response and VNG entirely. It has been suggested that VNG may add prognostic information by identifying the nerve of origin when a hearing preservation operation is considered.
Evaluation of the inferior vestibular nerve can be performed with vestibular evoked myogenic potential (VEMP) testing, which may further assist in determining the nerve of origin. A VEMP is elicited by stimulating the saccule through a high-intensity sound stimulus. Excitation through the vestibulospinal central tract leads to a biphasic reflex potential from the ipsilateral sternocleidomastoid muscle. Patients with inferior vestibular nerve tumor commonly have an absent or reduced VEMP response when the caloric testing may be normal (58,59); however, VEMP responses may be normal in the presence of an inferior vestibular nerve tumor. It is likely that the presence of a VEMP response is more closely related to the size and ensuing neural compression of a tumor within the IAC than by the nerve of origin (60).
Imaging Studies
Advancements in radiographic imaging modalities continue to improve the diagnosis of CPA tumors. Small tumors can be diagnosed with high sensitivity. Magnetic resonance imaging (MRI) is the gold standard in evaluation of patients with unilateral auditory and vestibular dysfunction. Noncontrasted T1-weighted images typically display vestibular schwannomas as hyperintense relative to CSF and iso- to hypointense to gray matter, while T2-weighted images show these tumors as hypointense to CSF and iso- to hyperintense to gray matter. These lesions typically have a component filling the IAC and may extend medially into the CPA having an “ice cream cone” or “mushroom” appearance (Fig. 159.2). NF2 patients may develop large vestibular schwannomas that can compress the brainstem and cerebellum and lead to obstruction of the fourth ventricle (Fig. 159.3). Volumetric high-resolution T2 sequence can reveal the fluid-filled inner ear and tumor invasion into the labyrinth can be seen as a filling defect. T2 images can be useful in identifying hyperintense cystic regions within the tumor, while the solid portion of the tumor appears as a hypointense filling-defect within the CPA and/or the IAC. Additionally, the T2 images provide vital information regarding the lateral relationship of the tumor with the fundus of the IAC. CSF within the fundus of the IAC, adjacent and lateral to the tumor, is known as a “fundal cap,” (Fig. 159.4) and its presence has been demonstrated to positively affect hearing preservation outcomes in middle fossa tumor resection (61).
![]() Figure 159.2 Axial T1-weighted contrast-enhanced MRI image at the level of the IAC demonstrating an enhancing right vestibular schwannoma with an “ice cream cone” or “mushroom” appearance. |
![]() Figure 159.3 Axial T1-weighted contrast-enhanced MRI image at the level of the IAC demonstrating bilateral enhancing vestibular schwannomas with brainstem compression. |
![]() Figure 159.4 T2 fast spin-echo magnetic resonance image of a left intracanalicular vestibular schwannoma. Note the presence of CSF in the fundus lateral to the tumor. |
The use of gadolinium-based contrast is standard in imaging of the CPA and the contrast-enhanced T1-weighted images of a vestibular schwannoma reveal marked tumor enhancement and can result in the detection of tumors as small as 1 to 2 mm. To reduce the expense of enhanced MRI some have advocated the use of noncontrast heavily T2-weighted fast spin echo (FSE) techniques. Comparisons of gadolinium-enhanced T1-weighted MRI with T2-weighted FSE sequences have demonstrated the reliability of T2 FSE sequences to detect mass lesions within the IAC and CPA and may be used as a screening tool for patients with sensorineural hearing loss or for those with contraindications to contrast administration (62,63).
Postimaging analysis and interpretation is vital in predicting outcomes and guiding clinical management. The standard documentation of tumor size is to record linear measurements in three dimensions; however, volumetric analysis of tumor size appears to be more sensitive in monitoring small changes of tumor growth over time (64). Prediction of hearing preservation following tumor resection has been shown to correlate with tumor volume (65).
Although MRI is the standard for diagnosis of vestibular schwannomas, patient movement causing motion artifact can limit the study resolution. Patients may not be able to tolerate the close confines of the MRI; however, mild sedatives or open MRI units may improve patient compliance. Due to the strong magnetic field, the presence of certain metallic foreign bodies or implants is a contraindication of MRI. The safety of MRI in patients with otologic or cochlear implants has been debated and concern has been raised for implant heating, malfunction, and/or displacement. Some early stapes prostheses were made with ferromagnetic materials that would, potentially, be unsafe in an MRI. Currently, most stapes prostheses are considered safe for 1.5-Tesla magnet of MRI (66,67). NF2 patients face bilateral hearing loss and may undergo placement of cochlear or auditory brainstem implantation for rehabilitation; yet, they have a lifelong need for serial imaging. If implant magnets are removed, these patients can undergo MRI safely. Although not FDA approved, Crane et al. (68) suggest that cochlear implant patients can safely undergo MRI with the receiver magnet left in place, as long as the device is bound tightly by head wrapping prior to scanning in a low field strength magnet.
Computed tomography (CT) scanning is not ideal for CPA examination but may be used in patients who cannot undergo MRI. One-millimeter CT scanning in both the axial and coronal planes provides excellent detail of the temporal bone and IACs. Expanding vestibular schwannomas may lead to expansion of the IAC, which may be demonstrated on CT. The soft tissue resolution of CT scan, even with contrast administration, is inferior to MRI and tumors less than 1 cm can be missed on CT (69). Tumors that cause erosion of the otic capsule or large tumors that extend into the CPA and compress the brainstem are readily visible on CT. Historically, air-contrast was administered through a lumbar puncture to increase the sensitivity of CT to localize intracanalicular tumors.
Treatment Options
When considering treatment options for patients with vestibular schwannomas, the utmost priority is preservation of the patient’s life, which is then followed in priority by preservation of brainstem and facial nerve function. Hearing preservation is an important objective; however, patients may be rehabilitated following the loss of hearing with contralateral routing of signals (CROS) type of hearing aids or bone-anchored devices.
Patients with vestibular schwannomas are faced with three primary treatment options: observation with serial imaging, stereotactic radiation, and microsurgical excision. Factors that affect treatment decision include patient preference, patient age/medical health, tumor size and location, auditory and vestibular function on the tumor and contralateral side, and tumor progression. The skill and experience of the treating team should also be considered. Because of the dearth of high-quality outcomes studies, patients must choose from these several options when deciding on how to manage their tumor.
Observation
Because vestibular schwannomas are most often slowgrowing tumors, observation with serial imaging is recommended in 25% to 30% of our patients. At the time of diagnosis, the rate of tumor growth is unknown and somewhat unpredictable. Rates of tumor growth range widely in the literature, but meta-analyses indicate that at least 50% of vestibular schwannomas exhibit growth and growth
ranges from 0.25 to 3.2 mm/y (70,71,72). In our patients, 66% demonstrated volumetric growth over an average follow-up of 3 years. Interestingly, NF2-associated vestibular schwannomas had an independent protective effect against deterioration of the pure tone average (73). NF2-associated vestibular schwannomas have been shown to exhibit a growth rate of 1.3 mm/y (74). Identification of predictors of tumor growth has been limited; however, patients that are younger, experience tinnitus initially, have large tumors at the time of presentation, have cystic tumors, or experience significant growth during the first year after diagnosis are more likely to have persistent tumor growth (75,76,77). If observation of the vestibular schwannoma is selected as the clinical path, the first follow-up MRI is recommended at 6 months and yearly thereafter if no growth is detected with volumetric measurements. Tumors may exhibit significant variability in growth and may experience precipitous increases in size with significant morbidity and even mortality (78,79); therefore, observation carries inherent risk.
ranges from 0.25 to 3.2 mm/y (70,71,72). In our patients, 66% demonstrated volumetric growth over an average follow-up of 3 years. Interestingly, NF2-associated vestibular schwannomas had an independent protective effect against deterioration of the pure tone average (73). NF2-associated vestibular schwannomas have been shown to exhibit a growth rate of 1.3 mm/y (74). Identification of predictors of tumor growth has been limited; however, patients that are younger, experience tinnitus initially, have large tumors at the time of presentation, have cystic tumors, or experience significant growth during the first year after diagnosis are more likely to have persistent tumor growth (75,76,77). If observation of the vestibular schwannoma is selected as the clinical path, the first follow-up MRI is recommended at 6 months and yearly thereafter if no growth is detected with volumetric measurements. Tumors may exhibit significant variability in growth and may experience precipitous increases in size with significant morbidity and even mortality (78,79); therefore, observation carries inherent risk.
Observation may allow enough time to determine the tumor growth characteristics, and if no growth is demonstrated, then no treatment may ever be necessary. If symptoms change or tumor growth is seen, then patients may undergo intervention when appropriate. If hearing is present at the time of tumor discovery, the most likely complication of observation is a decline in auditory function during the observation period. The opportunity for hearing-preservation approach may be lost. Observation is a reasonable option for patients who do not have significant brainstem compression or when the tumor affects an only-hearing ear. We are inclined to recommend observation when the patient is over 65 years of age, or if they are in poor medical health. Patients’ preference certainly plays a role. In spite of the unpredictability of tumor and associated symptoms, observation may afford years of residual auditory function without the risk of radiation or surgery.
Stereotactic Radiation
Pioneered by the Swedish neurosurgeon, Lars Leksell, in the 1950s, stereotactic radiation therapy has become a vital tool in the management of a wide variety of intracranial pathology. Intracranial stereotactic radiation therapy involves the administration of radiation to a precise location so as to induce radiation damage to the target area and minimize the effect to the adjacent structures. Current modalities for radiation for vestibular schwannoma include single fraction gamma radiation therapy from an active cobalt-60 source (Gamma Knife), fractionated radiation, hypofractionated linear accelerator photon radiation therapy (XKnife, CyberKnife, Trilogy, Novalis, and SyngergyS), and proton beam. The term “radiosurgery” is commonly employed to describe the precision of such radiation; however, it is a misnomer since it involves no surgery and the radiation is delivered to a closed intracranial lesion. Vestibular schwannomas are treated with a highly conformal prescribed dose of radiation under the direct supervision of a radiation treatment team, which typically consists of a surgeon (neurosurgeon or neurotologist), a radiation oncologist, and a physicist.
The goals of stereotactic radiation therapy are to provide the lowest effective dose of radiation that induces longterm tumor growth control without subjecting patients to the risk of surgical intervention and to prevent acute loss of neurologic function. The technique for stereotactic radiotherapy varies depending on the modality used. For gamma knife, patients undergo head frame placement with pretreatment MRI and/or CT imaging, which are then uploaded to the radiation dose-planning computer where the images can be examined for inaccuracies and appearance of the pathology. Both CT and MRI studies can be fused to accentuate the lesion and the surrounding structures. The margins of the tumor are outlined and then the treatment team proceeds with dose planning to contour the treatment dose to the tumor with a sharp radiation dose drop-off at the margins of the tumor (Fig. 159.5). Once all members of the team confirm the treatment plan and dose, patients are placed in the treatment suite and the head frame is immobilized on the table and advanced into the unit for treatment. The gamma knife dose used to treat vestibular schwannomas is, generally, between 12 and 13 Gray (Gy) to 50% isodose line at the periphery of the tumor to maximize tumor effect and minimize complications (80,81,82). The computer software calculates the time and exact positioning of the patient within the unit to achieve the treatment dose. Linear accelerators differ, in that patients do not require head frame placement or rigid fixation and they are fitted with a mask that provides immobilization. A series of real-time radiographic images are obtained during treatment to confirm correct positioning and to provide guidance during the treatment. CyberKnife treatment doses vary significantly but, typically, a total of 18 Gy is delivered over three fractions and has been reported to be safe and effective (83). Following stereotactic radiotherapy,
the head frame is removed, patients are observed for a few hours and then they are discharged home. Serial MRI images are obtained over the patient’s lifespan to monitor for persistent tumor growth.
the head frame is removed, patients are observed for a few hours and then they are discharged home. Serial MRI images are obtained over the patient’s lifespan to monitor for persistent tumor growth.
Outcomes for tumor growth control and neurologic preservation vary greatly in the literature and lack uniform reporting (84). Additionally, many vestibular schwannomas do not grow over the course of time, which makes the assessment of the true efficacy of treatment difficult to determine. Nevertheless, patients who have had stereotactic radiotherapy have reported growth control rates of 90% to 100% (85,86,87,88,89,90,91,92,93,94,95,96,97,98,99,100,101,102) for gamma knife and 98% for CyberKnife (83). NF2 patients treated with gamma knife have reported actuarial tumor control rates of 71% to 85%, within declining rates with longer follow-up (103,104,105), while data on CyberKnife treatment of NF2 patients is limited, but has been reported to have over 90% tumor control rate in a small group of patients with short follow-up (less than 25 months) (106).
Stereotactic radiotherapy is an attractive option for many patients and has the benefit of no hospitalization and the rapid return to normal activity and work almost immediately after treatment. This treatment is indicated for those who have demonstrated tumor growth, are elderly, or have medical contraindications to microsurgery. Radiation lacks the microsurgical risks of infection and CSF leak. A wide variety of protocols have been reported that generally recommend treatment of tumors that measure less than 2.5 to 3 cm. Stereotactic radiation also is a valuable tool to treat tumor recurrence following microsurgery (107). Patients who have radiation treatment of their vestibular schwannoma rarely experience complete resolution to the mass; therefore, they are faced with a need for prolonged surveillance with repeat MRI during the course of their life.
Preservation of neurologic function is a primary goal of stereotactic radiotherapy; however, the development of adverse side effects is directly related to radiation dose. Complications of stereotactic radiotherapy are similar to microsurgical resection. Hearing loss may occur acutely following radiation, but, typically, hearing loss occurs gradually months to years later. Hearing preservation outcome reporting lacks uniformity (84); but is reported to range from 50% to 86% (108,109). This hearing loss has been linked to radiation of the cochlea and maintaining a cochlear dose less than 6.9 Gy is important in preserving residual hearing (110,111). The actuarial serviceable hearing preservation rates of NF2 patients has been reported at 73%, 59%, and 48% for 1, 2, and 5 years, respectively (105).
Facial and trigeminal cranial neuropathies due to radiation treatment are similar in incidence and are reported around 0% to 5% in sporadic vestibular schwannomas and 8% in NF2 patients; however, these rates increase with doses greater than 13 Gy (112,113). Hemifacial spasm may also occur in 2% to 4% and may be related to a delayed insult to the facial nerve (114,115). Hydrocephalus without evidence of tumor growth may occur in up to 5% of patients and is more common in patients with larger tumors that are treated with radiotherapy and may be related to proteinaceous debris obstruction of CSF flow (116,117). Chronic vestibular dysfunction may be present in 13% to 26% of patients following stereotactic radiotherapy (114,118). Radiation has been reported to cause fibrosis of the facial nerve leading to poorer facial nerve outcomes if microsurgical resection is necessary following radiotherapy (119,120). Others, however, have reported no significant changes in facial nerve histology or functional outcomes (121). Malignant transformation and delayed oncogenesis, which is uniformly lethal, has been documented with stereotactic intracranial radiotherapy, and treatment with radiotherapy carries a 1:1,000 risk over a 5- to 30-year period (122,123,124,125,126,127,128,129,130,131).
Stereotactic radiotherapy remains a viable treatment option for patients with vestibular schwannoma. Irregularities in reporting outcomes have made it difficult to fully compare radiotherapy with microsurgical intervention. The long-term effect of radiation on tumor control and neurologic functions is not fully known and longitudinal follow-up will prove beneficial in informing patients and directing clinical management of vestibular schwannomas.
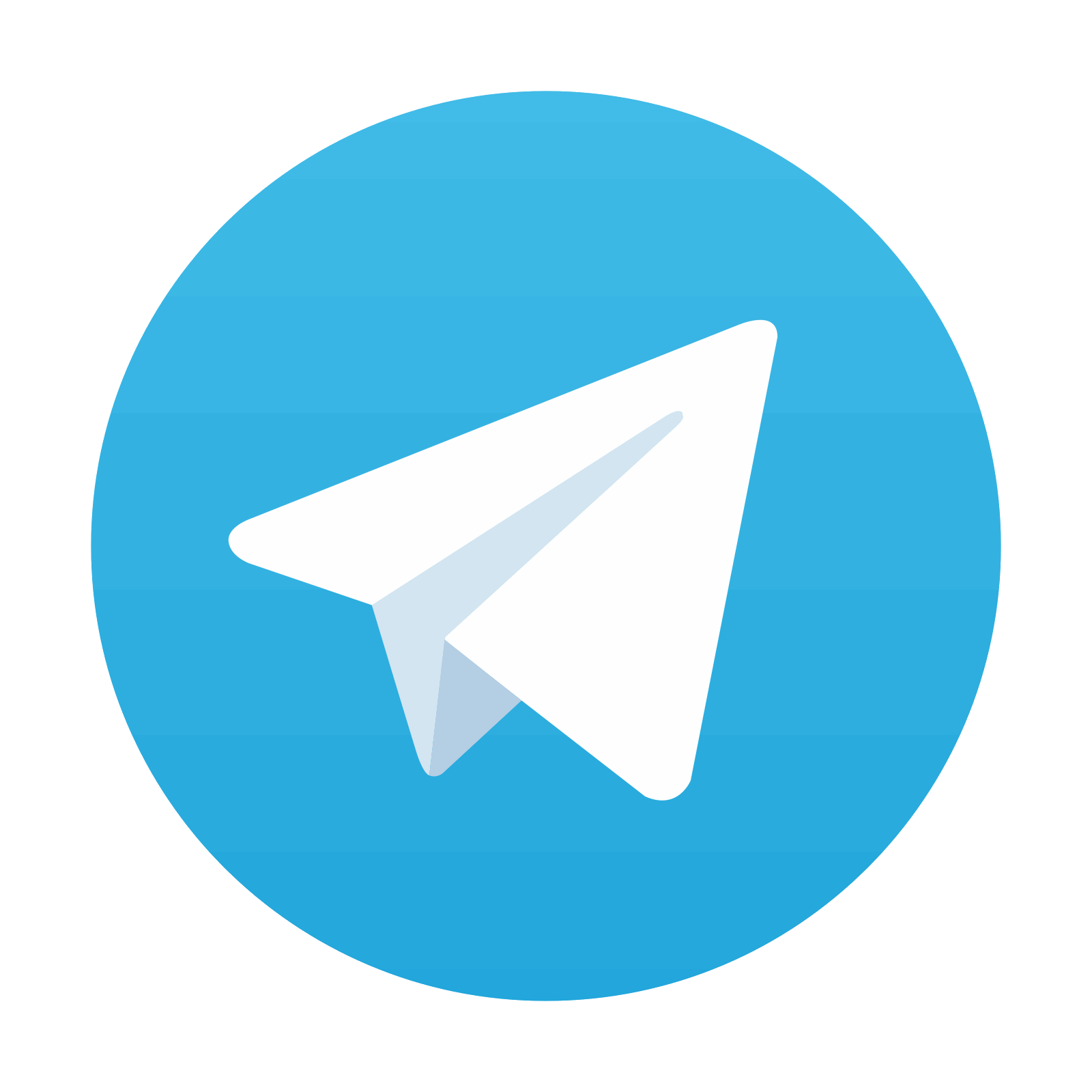
Stay updated, free articles. Join our Telegram channel

Full access? Get Clinical Tree
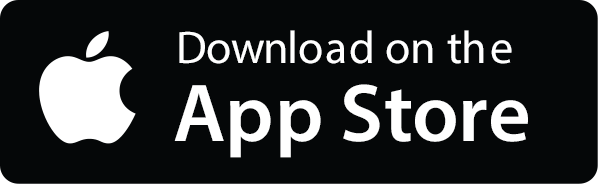
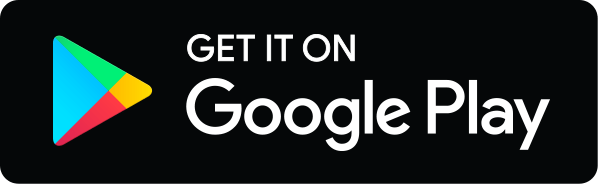