Fig. 24.1
Challenges to be overcome in cell therapy for hair cell regeneration. In left hand, requirements for preparation and transplantation of stem/progenitor cells are listed. In the middle, survival, proliferation, and differentiation should be tightly controlled after cell transplantation into the cochlea. In right hand, transplants-derived hair cells should be integrated into the organ of Corti and recapitulate native hair cells in terms of planar cell polarity, tonotopy, and innervation from the spiral ganglion neurons
24.3.1 How to Apply Exogenous Cells and Possibility for Tissue Implantation into the Cochlea
On cell-based medicine generally, including in cochlea and other organs or tissues, cells are multiplied in culture or used with minimal manipulation after harvesting and then delivered by one of the two major strategies. In one hand, cells are suspended in medium and directly injected into the defective tissues. The transplanted cells are expected to home to the damaged area or the site of interest. In any cases, little guidance is provided to the cells by the transplant system before and after transplantation. Alternatively, cells may be allowed to adhere to a material carrier in vitro, be allowed to proliferate and differentiate within a bioreactor, and subsequently be implanted on the material including functional cells to a specific anatomical site. The material serves as a template to guide tissue formation and typically is designed to degrade in concert with the deposition of new extracellular matrix and cell proliferation [19]. However, our knowledge is still limited at this moment about when and how much amount of stem cells should be transplanted into the inner ear. It should be also clarified how frequently stem cells should be administered into the inner ear. Regarding cell transplantation into the cochlea, the strategy to perform single injection of exogenous cells has been solely adopted in the studies reported to date. In the previous works, direct transplantation to the cochlea is most commonly used, however, the site of cell injection varies among studies. Cochleostomy in the lateral wall [14, 20], the round window [21], the lateral semicircular canal injection [12], the internal auditory meatus [22], and utriculostomy [23] have been tested.
Transvascular delivery of stem cells would be another option to have transplanted cells reach the cochlea, although efficiency would be very small without any manipulation after transplantation since the inner ear is quite a small organ in the body in terms of weight, cell number, and blood flow, so that most part of transplanted cells could escape to other organs or tissues in the body. The concept for organ-specific “ZIP code” would solve these issues on transvascular stem cell delivery. The general idea is that each organ and pathology has a unique vascular “ZIP code” that can be targeted with affinity ligands [24]. Organ- or pathology-specific delivery of drugs or nanoparticles containing small nucleotides has been explored for treatment of cancer, congenital enzyme defects, or regenerative medicine [25–27]. These strategies employ carrier molecules that bind to receptors exposed on the cell surface in target tissues or pathology. If “ZIP codes” in the cochlea would be identified, cell delivery based on tissue-specific “ZIP code” would become available, and transvascular delivery of stem cells to the cochlea could be powerful tools for regenerative therapy for hearing loss because no surgical approaches are required to have stem cells reach to the cochlea.
Finally, although there would be many challenges to transplant new tissues into the cochlea, it seems to be worth to try tissue implantation into the cochlea. Cell sheet formation with cellular mosaic of hair cells and supporting cells has not been achieved even in in vitro stem cell experiments. Hair cell-like cells have already been induced from ES cells or iPS cells [28]; however, the formation of a cell sheet of inner ear sensory epithelium has never been accomplished in vitro. If cell sheet formation that mimics organ of Corti would be accomplished, then next target would be the three-dimensional culture of stem cell-induced sensory cells. Three-dimensional culture from stem cells to cochlear spiral seems to be quite attractive. Indeed, Koehler et al. demonstrated a stepwise method for the differentiation of mouse embryonic stem cells to inner ear sensory epithelia [29]. In this study, clusters of embryonic stem cells recapitulate in vivo development with precise temporal control of signaling pathway and form sensory epithelia that look like the vestibular end organs in three-dimensional culture system.
24.3.2 Stem Cell-Based Regenerative Medicine and Artificial Organ
Artificial organs versus cell-based regenerative medicine would be another major issue in debate when cell-based medicine would be clinically applied. Various artificial organs have been subjects of intensive research and development and now widely used in patients. Cochlear implants are one of the most successful artificial organs, and approximately 324,200 people worldwide have received cochlear implants according to the Food and Drug Administration in the United States [30].
Artificial organs are roughly categorized into three groups as follows: (1) mechanically engineered artificial organ, (2) in situ tissue engineering, and (3) organ designed by stem cell-based regenerative medicine. In addition, organ transplantation from the brain dead would be the fourth in a broad sense. As for mechanically engineered artificial organs, there are two subgroups of artificial organs, ones are for life support while awaiting an organ transplantation or regeneration process and the others are for improvement of the patient’s ability. Artificial lung and heart are sometimes used for patients while awaiting organ transplantation. In addition, artificial dialysis is also widely used in clinic to replace the function of kidney in chronic renal failure patients. However, devices that release patients from continuously tethering to stationary resources or power supply have not become available in these artificial organs. On the contrary, some of artificial organs have prevailed these days for the improvement of the patient’s ability. Artificial lens after cataract removal, or artificial joint prosthesis, are clinically used in common while these artificial organs work without any power supply. In contrast, cardiac pacemaker and cochlear implant are the most successful mechanically engineered artificial organs to date since these are working for more than ten years without replacing power supply. Clinical indication and characteristics of cochlear implants are discussed in the Chap. 27 by Yamazaki in this book.
In situ tissue engineering is applied for tissues or organs that possess abilities to regenerate themselves, such as skin, bone, trachea, or blood vessels. A typical approach uses biodegradable scaffolds that attract endogenous stem cells and growth factors to regenerate tissue of interest. It has been also accepted that inflammation is not only a detrimental response to biomaterials, but, when harnessed properly, it can also be exploited to induce a natural regenerative response. To the best of our knowledge, in situ tissue engineering for cochlear regeneration has not been achieved yet to date. Alternatively, transplantation of organs designed by stem cell-based regenerative medicine appears to be the third option for regenerative medicine using artificial organs. Indeed, in vitro studies by Oshima et al. showed that both mouse embryonic stem cells and iPS cells can be induced to commit ectodermal cell fate and differentiate into hair cell-like cells in mammalian inner ear [28]. Moreover, Koeler et al. demonstrated a three-dimensional culture system that subjects embryonic stem cells to form otocyst-like cell clusters, which finally form sensory epithelia that resemble the vestibular end organs [29]. These findings indicate that the formation of artificial cochlea designed by stem cell-based regenerative medicine would be a promising strategy for regeneration of cochlear sensory epithelium. Accurate innervation could be a next mark to accomplish once transplantation of artificial cochlea would be realized in vivo.
Organ transplantation from donors of brain dead is widely performed in the cases with chronic organ failure, including kidney, lung, heart, and liver. However, it has not been the case in the cochlea or inner ear to date, since excision and transplantation of the inner ear are technically challenging.
These categories listed above are not independent, but overlap in part one another and mutually interact to develop and improve function in the organ of interest. It does not matter whether these tissues are cultivated inside or outside the patient’s body in order to regenerate functional organs or tissues. Therefore, it would be very important to clarify what situation would be suitable for cell-based regenerative medicine and what would be the contraindication in the future.
24.3.3 In Vivo Tracking of Stem Cells After Transplantation
In the last, but not least, in vivo tracking of behavior of transplanted stem cells should be of interest even though it is a challenge under currently available technology. Tracking the dynamic behaviors of transplanted stem cells has been a long-standing research goal of biologists, medical scientists, and engineers. As mentioned above, the cochlear duct is surrounded by the bony wall that prevents target tissues or cells from being directly observed by optical microscope. The development of the way to trace transplanted cells in intact cochlea would be a major advance in the area of stem cell research in the inner ear. The ideal tracer molecule should fulfill a number of criteria: biocompatibility, absence of genetic modification to stem cells, single-cell detection at any atomic location, quantification of cell number, minimal dilution with cell division, minimal transfer of contrast agent to non-stem cells, noninvasive imaging in the living subject over months to years, and absence of requirement for ejectable contrast agent [31]. Radionuclide labels possess a number of characteristics that account for their preferential use in clinical practice. First they are relatively safe, and second, radionuclide methods are highly sensitive and quantifiable. However, the tracking of labeled cells is limited by temporal loss of radio tracer. Moreover, physiological accumulation and excretion of free radio tracer may interfere with the activity in labeled stem cells. In the study by Bos et al., rat mesenchymal stem cells were labeled with superparamagnetic iron oxide (SPIO), administered intravenously, and tracked with magnetic resonance (MR) imaging [32]. MR imaging of SPIO-labeled stem cells offers a noninvasive evaluation of stem cell engraftment in host organs; however, excessive iron load from SPIO labeling impairs differentiation of stem cells. Some of the recent studies, including one by Nejadnik et al., have explored the way to reduce iron exposure and improve efficacy for the differentiation of stem cells [33]. Real-time imaging and longitudinal tracking of stem cells in in vivo three-dimensional tissue environments have been explored with an integrated optical microscope. The integrated microscope combines multiple imaging functions derived from optical coherence tomography (OCT) and multiphoton microscopy (MPM), including optical coherence microscopy (OCM), microvasculature imaging, two-photon excited fluorescence (TPEF), and second harmonic generation (SHG) microscopy [34]. Stem cells labeled with fluorescence protein would be traced using these techniques with smallest surgical invasion to make a pinhole for observation inside the cochlear duct.
24.4 Concluding Remarks
In this chapter, we briefly presented the anatomy of the cochlea and hair cells and discussed the current status and future directions of cell-based therapy for hair cell regeneration. Despite recent advances in the area of regenerative medicine in the inner ear, the exploration of replacement of damaged hair cells by cell transplantation has just started. Clearly, there are still many concerns and challenges in biological, clinical, and ethical aspects to overcome before clinical application of cell-based regenerative medicine in the inner ear. Indeed, recent progress of stem cell biology provides a variety of source for transplanted cells [35, 36]. Delivering cells directly to the organ of Corti would be ideal, but its small size and relative inaccessibility should be major obstacles. Techniques to detect survival and differentiation of transplanted cells in vivo should be developed. Although there will be much work to be done, we believe the future of cell-based regenerative medicine for cochlear disorder would be bright.
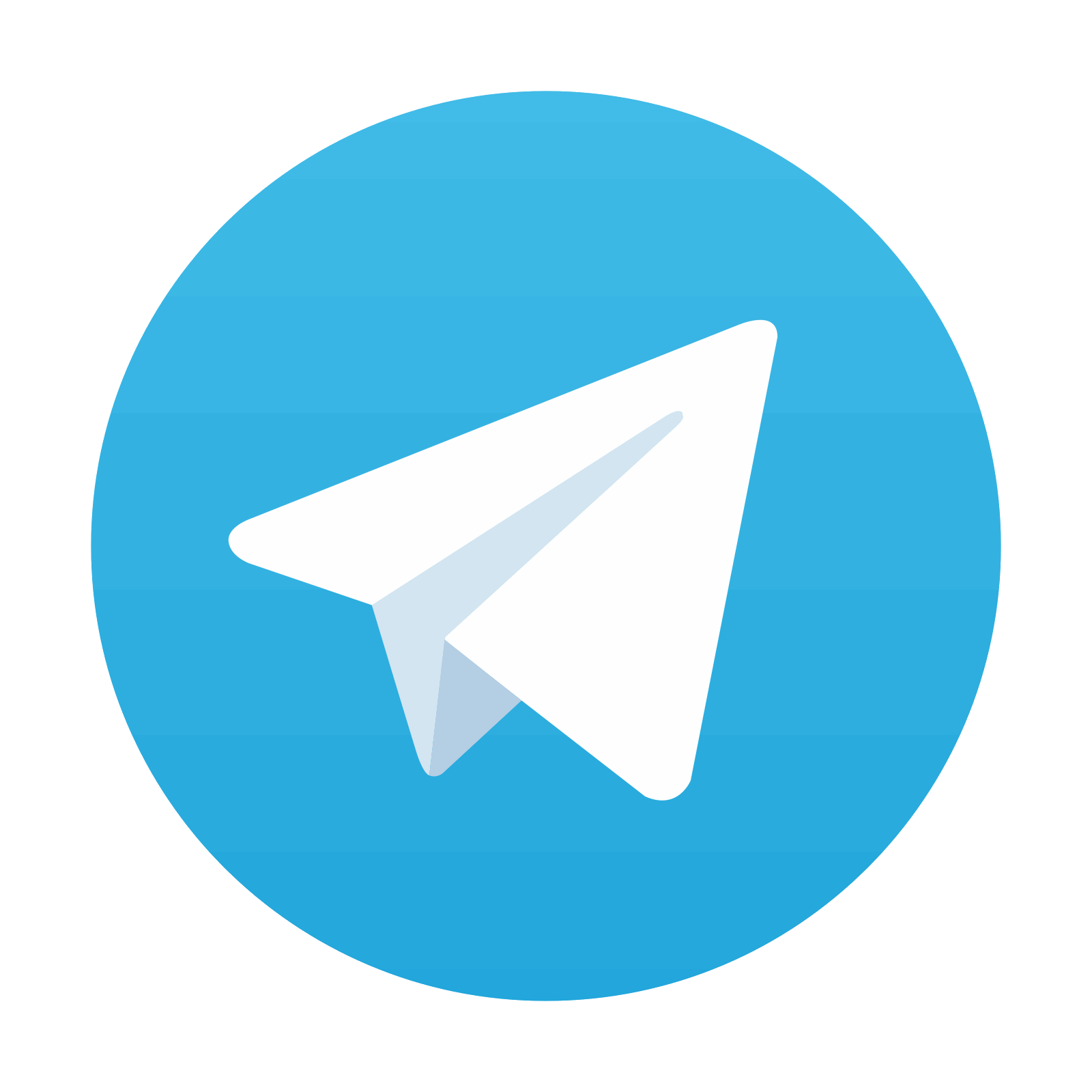
Stay updated, free articles. Join our Telegram channel

Full access? Get Clinical Tree
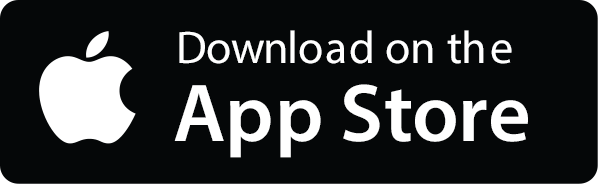
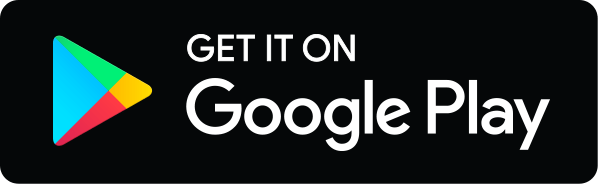