Bacterial biofilms are 3-dimensional aggregates of bacteria that have been shown to play a major role in many chronic infections. Evidence is growing that bacterial biofilms may play a role in certain cases of recalcitrant chronic sinusitis that do not respond to traditional medical and surgical therapies. Novel therapies may have clinical applications to prevent and destabilize biofilms. Future research will determine if topical antimicrobials, surfactants, and other adjuvant therapies can be used to treat biofilm-associated chronic rhinosinusitis.
Chronic rhinosinusitis (CRS) is one of the most common chronic medical conditions, affecting between 14% and 16% of the US population. Direct health care costs are significant and are estimated to be more than $5.8 billion per year. According to the most recent data from the National Health Interview Survey (2007), rhinosinusitis continues to be one of the top 10 leading diagnoses of office visits in the United States. Patients with CRS demonstrate lower quality-of-life scores than those suffering from chronic obstructive pulmonary disease, congestive heart failure, back pain, or angina. However, some patients with CRS suffer from persistent and recurrent infections despite maximal medical management and surgery. Since the initial description of biofilms on the sinonasal mucosa of patients with CRS, there has been a growing body of evidence supporting the contributory role of biofilms in poor disease progression and persistent sinonasal inflammation.
Biofilms overview
It is currently estimated that at least 65% of all human bacterial infections may involve biofilm formation. These include a diverse range of infectious processes, including dental caries, periodontitis, musculoskeletal infections, osteomyelitis, bacterial prostatitis, endocarditis, and cystic fibrosis pneumonia. Biofilms have also been implicated in several conditions seen in an otolaryngology practice, including otitis media, chronic sinusitis, chronic tonsillitis, adenoiditis, and device infections (such as in cochlear implants, tympanostomy tubes, and tracheostomy tubes). Bacterial biofilms are described as surface-associated communities of microorganisms encased in a protective extracellular matrix. The life cycle of bacterial biofilms can be divided into 5 parts ( Fig. 1 ). Biofilms are initiated when free-floating, planktonic bacteria anchor to biologic or inert surfaces. The attached bacteria multiply and progress from a state of monolayer to a microcolony and then to a critical mass, in which interbacterial crosstalk occurs, triggering a phenomenon known as quorum sensing that leads to the biofilm phenotype. The bacteria respond collectively to express factors that are specific to the biofilm phenotype, which lead to the secretion of an exopolysaccharide matrix. This biofilm phenotype is characterized morphologically by the formation of microbial towers, which are composed of layers of embedded, live bacteria with intervening water channels. Under the right environmental conditions, free-floating bacteria are released from the biofilms, and the cycle is continued at other surfaces. Approximately 80% of the world’s microbial biomass resides in the biofilm state, and the National Institutes of Health estimates that more than 75% of microbial infections that occur in the human body are underpinned by the formation and persistence of biofilms.
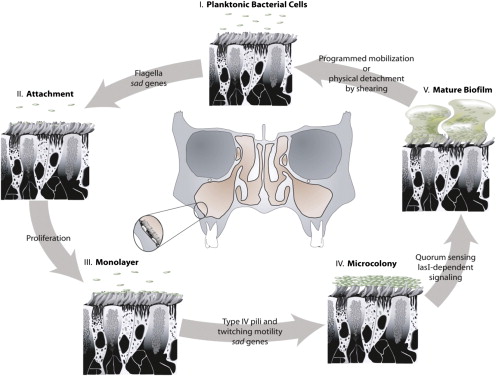
Biofilm formation is thought to provide a mechanism for enhanced bacterial survival. Bacteria in biofilms lack the antibiotic susceptibility of planktonic bacteria and can be up to 1000 times more resistant to antibiotic treatment. Bacteria in biofilms are also more resistant to host defenses, because the extracellular matrix that makes up most of the biofilm serves to protect the bacteria against antibodies, immune-system phagocytosis, antibiotic penetration, and complement binding. There is also a decreased need for oxygen and nutrients when bacteria exist in the biofilm state, further reducing susceptibility to certain antimicrobials ( Box 1 ). This is especially true for bacteria within the core of the biofilm mass. In addition, biofilms are environments where bacteria can share their DNA by transfer of genetic information via plasmids to encourage variability and adaptive mutations, such as antibiotic resistance. All of these properties encourage persistence of bacteria for extensive periods despite antibiotic treatment, resulting in chronic disease with intermittent acute infections. Biofilms also provide a source for recurrent infections by releasing planktonic bacteria, resulting in implantation and population of new anatomic locations.
- •
Three-dimensional aggregates of bacteria encased in a secreted exopolysaccharide matrix
- •
Increase evasion of host defenses
- •
Increase resistance to antibiotic therapy
- •
Release of planktonic bacteria can result in new or acute infections in the host
Mucosal biofilms and CRS
It is important to note that biofilms have a heterogeneous morphology because the biofilm phenotype is highly dependent on the surrounding environmental factors, including pH, nutrient availability, and oxidative potential. Bacterial biofilms that have formed on mucosal surfaces are referred to as mucosal biofilms. Mucosal biofilms have a unique cascade of gene expression compared with biofilms on inert surfaces. Bacteria of mucosal biofilms must overcome the normal airway mucociliary clearance that usually protects the upper airways and sinonasal tract. With further detail in the sections to follow, multiple studies have visualized biofilms in an animal model of sinusitis, on frontal sinus stents removed from patients with CRS, and from the mucosa of patients undergoing sinus surgery. Fig. 2 depicts 3 separate methods of identifying bacterial biofilms from the same patient with CRS. Studies have shown that the presence of biofilms is associated with worse postoperative outcomes after endoscopic sinus surgery. Treatments of biofilm-associated CRS are generally aimed at disrupting the biofilm life cycle and are described in the later sections.
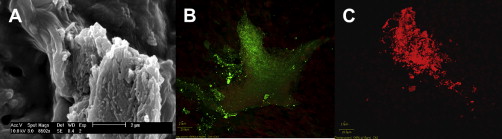
Mucosal biofilms and CRS
It is important to note that biofilms have a heterogeneous morphology because the biofilm phenotype is highly dependent on the surrounding environmental factors, including pH, nutrient availability, and oxidative potential. Bacterial biofilms that have formed on mucosal surfaces are referred to as mucosal biofilms. Mucosal biofilms have a unique cascade of gene expression compared with biofilms on inert surfaces. Bacteria of mucosal biofilms must overcome the normal airway mucociliary clearance that usually protects the upper airways and sinonasal tract. With further detail in the sections to follow, multiple studies have visualized biofilms in an animal model of sinusitis, on frontal sinus stents removed from patients with CRS, and from the mucosa of patients undergoing sinus surgery. Fig. 2 depicts 3 separate methods of identifying bacterial biofilms from the same patient with CRS. Studies have shown that the presence of biofilms is associated with worse postoperative outcomes after endoscopic sinus surgery. Treatments of biofilm-associated CRS are generally aimed at disrupting the biofilm life cycle and are described in the later sections.
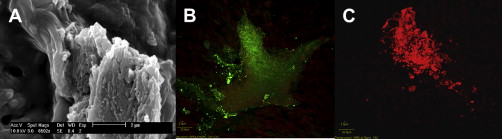
Visualization of bacterial biofilms
A variety of sophisticated assessment techniques have shown the presence of bacterial biofilms on sampled sinonasal mucosa from animals and patients with CRS. The classic approaches have included both scanning electron microscopy and transmission electron microscopy (SEM and TEM, respectively). These techniques provide detailed imaging of the intricate architecture, developmental stages, and polymicrobial nature of biofilms. However, both the techniques can be limited in clinical utility due to difficulty in fixation, the presence of artifacts in the fixation process, and difficulty in identifying individual bacterial species. SEM also has some limitations in differentiating between mucus, clot, and biofilm, whereas TEM only renders a 2-dimensional section of the biofilm. Other techniques include fluorescence in situ hybridization (FISH) and confocal laser scanning microscopy (CLSM). FISH with CLSM provide both 3-dimensional biofilm structures and information regarding the polymicrobial nature of native biofilms.
Microbiology of bacterial biofilms
It is well known that one of the leading causes of medically refractory rhinosinusitis is the opportunistic gram-negative bacteria, Pseudomonas aeruginosa . A wealth of morphologic and physiologic evidence exists that biofilms enable P aeruginosa to persist in cystic fibrosis pulmonary infections. Biofilm formation has been demonstrated by many other bacterial species including Staphylococcus aureus , Streptococcus pneumoniae , Haemophilus influenzae , and Moraxella catarrhalis . After sampling 157 consecutive patients with CRS over a 4-month period, Prince and colleagues found that 28.6% of samples showed the potential for biofilm formation. P aeruginosa and S aureus composed 71% of the samples that showed biofilm growth. Polymicrobial samples with and without P aeruginosa , coagulase-negative staphylococci, and H influenzae made up the rest of the biofilm formers in their study. Sanderson and colleagues used CLSM and FISH analyses to examine intraoperative samples taken from 18 patients with CRS and 5 controls undergoing septoplasty for the presence of biofilms. The analysis found 78% (14 out of 18) of patients with detectable bacteria in a biofilm matrix. The predominant biofilm forming species was H influenzae followed by S pneumoniae and S aureus , with P aeruginosa notably absent. They also found biofilms on 2 of 5 of the controls, underscoring the need to better clarify the connection between CRS and biofilms.
Increased antibiotic resistance is a trait common to biofilm bacteria. Bacteria in biofilms show 10- to 1000-fold less sensitivity to antibiotics than bacteria growing in culture. The mechanisms of biofilm antibiotic resistance are currently under study. Theories include a combination of restricted antibiotic penetration through the biofilm extracellular matrix, reduced metabolic activity and growth rate, upregulation of efflux pumps, and subpopulations of phenotypically and genetically different bacteria. Another hypothesis proposes that the basal layers of the biofilm are composed of bacteria in a dormant state that is secondary to the accumulation of inhibitive waste products or the depletion of necessary substrate. The decrease in metabolic activity and growth rate may be explained by altered genetic expression in bacterial biofilms leading to a persister cell phenotype. A recent study by May and colleagues demonstrated that subinhibitory concentrations of antibiotics triggered biofilm formation in Escherichia coli and the induction of antibiotic efflux pumps. This study suggests that inadequate doses or courses of antibiotic treatment can trigger biofilm formation and lead to chronic infections.
Fungal biofilms
A variety of fungal species have been demonstrated to form biofilms in vivo and in vitro. Candida spp are among the most common causative agents of yeast biofilm infections; however, Cryptococcus , Pneumocystis , Aspergillus , Coccidioides , and other fungal species have been implicated as well. Among the pathogenic fungi, Candida albicans is most frequently associated with biofilm formation, especially with infection of indwelling medical devices. Chandra and colleagues demonstrated that C albicans isolates in the form of biofilm exhibited increased resistance to amphotericin B, nystatin, chlorhexidine, and fluconazole when compared with planktonic cultures. Characterization of fungal biofilms is difficult, but studies have demonstrated that fungal biofilms, like bacterial biofilms, have defined developmental phases. The main phases of biofilm development are adhesion, filamentation, and hyphal and yeast proliferation with maturation and production of the extracellular matrix. In one study, it was demonstrated that Candida spp were able to form biofilms by directly attaching to bacteria that have already colonized a surface. The extracellular matrix consists primarily of proteins, chitins, DNA, and carbohydrates. It covers the biofilm and is through to act as a protective barrier by preventing host immune factors, antifungals, and by impeding physical disruption of the underlying cells.
Fungal elements have been demonstrated within sinus mucosal biofilms in patients with CRS. The investigators theorized that fungi may contribute to the chronic inflammation or fungi and bacteria may interact in a symbiotic way to increase resistance to host defenses and treatment. Foreman and colleagues also identified mixed bacterial-fungal biofilms from intraoperative specimens in patients with CRS. In their study, 9 of 11 (82%) patients had mixed bacterial-fungal biofilms with either S aureus or H influenzae , whereas the remaining patients had only fungal biofilms. Further research is needed to determine what effects the presence of fungal biofilms has on sinus mucosa and in the pathophysiology of CRS.
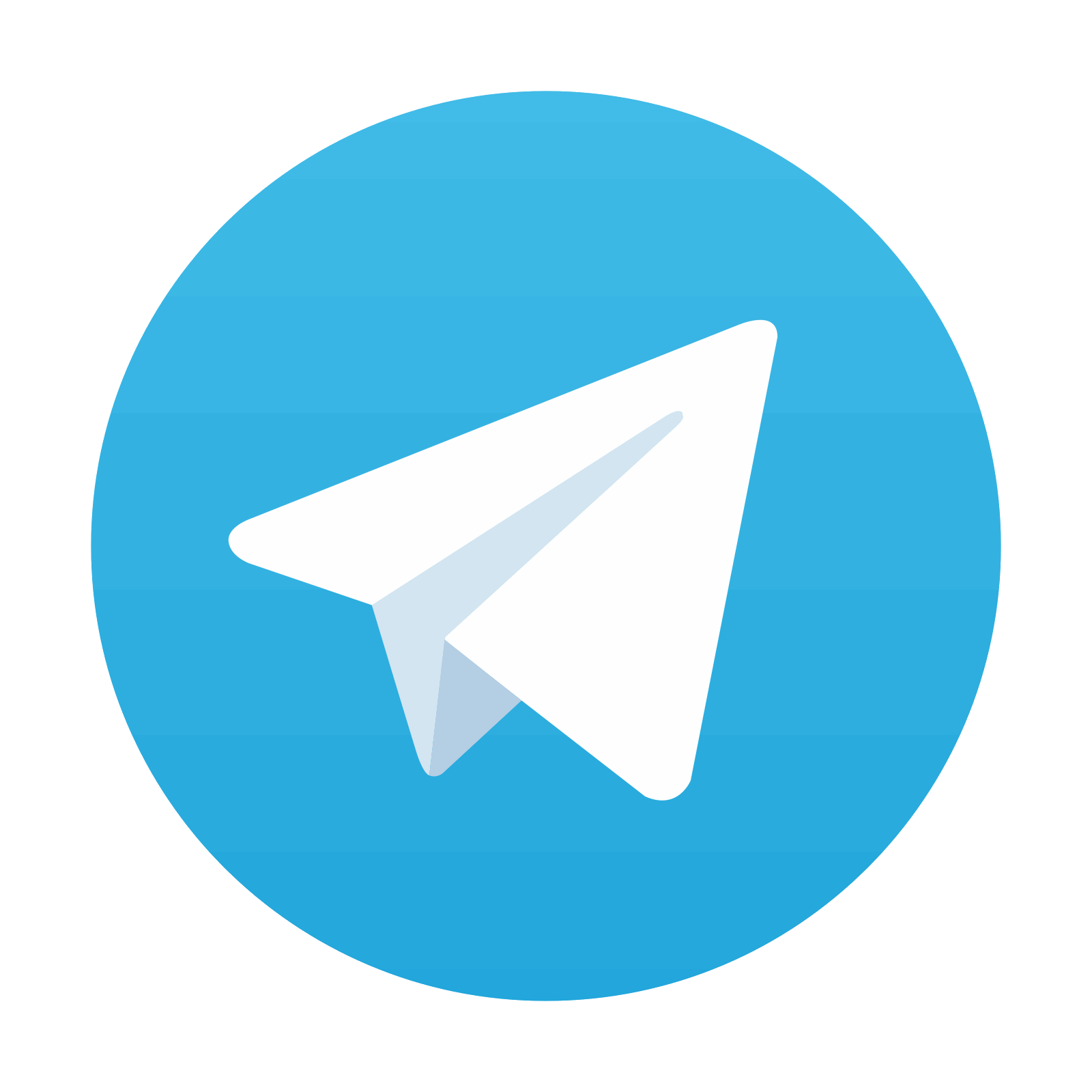
Stay updated, free articles. Join our Telegram channel

Full access? Get Clinical Tree
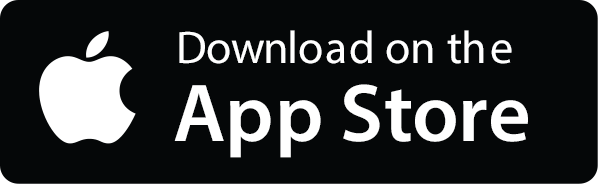
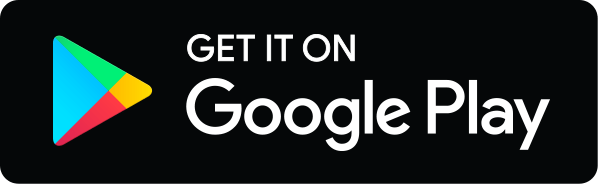