Binocular Vision: Adaptations in Strabismus and Monofixation
Bruce M. Schnall
NORMAL BINOCULAR VISION
Single binocular vision is the cortical integration of the similar retinal images produced by each eye into a single unified perception (1). It is important to recognize that binocular vision occurs at a cortical level and any changes or adaptations to binocular vision will occur on a cortical level as well. In children with infantile or congenital strabismus, early surgical alignment of the eyes is needed to either allow the cells for binocular vision to develop in the brain or prevent their loss during the critical period of binocular vision development (2). Cortical changes due to an abnormal visual experience in childhood are not unique to binocular vision. The loss of neurons in the geniculate body due to a unilateral blurred image in childhood is well documented in amblyopia (3).
The first step in understanding binocular vision is to recognize that retinal elements have a visual direction. The visual direction of a retinal element is determined by its relative position to the fovea (4). For example, if the retinal elements inferior to the fovea are stimulated, the visual direction of these retinal elements localize the image to an area in the superior visual field. An object seen by the fovea will localize to an area in space that is straight ahead. If light is shone onto the nasal retina, it will be sensed that this light is in the temporal visual field. An eye with a recent onset sixth nerve palsy will be held in adduction, such that nasal retina lies in the area normally occupied by the fovea. The nasal retina will continue to localize objects to the temporal visual field. In addition, an eye held in adduction is rotated such that the fovea now lies in an area normally occupied by temporal retina. A light shone on this fovea, which is now temporal, will be localized as straight ahead. A representation of the visual direction of retinal elements can be seen when performing visual field testing. Each point of the visual field relates to a specific area in the retina.
Retinal elements of the two eyes that share a common visual direction, or localize to the same area in space are referred to as corresponding retinal points. Similar images falling on corresponding retinal points are localized as coming from the same area in space by both eyes and can be fused and seen as a single image. An image that falls on noncorresponding retinal points are localized to two different areas in space and are seen as double. The horopter is a two-dimensional plane in space of points that fall on corresponding retinal points (Fig. 7.1). Only objects on the horopter will fall on corresponding retinal points. There is a small area in front of and behind the horopter in which the brain can fuse noncorresponding or disparate retinal points. Fusing the images that fall on these noncorresponding or disparate retinal points allows not only single binocular vision but also an individual to order these objects in space, giving rise to stereopsis. This area just in front and behind the horopter is referred to as the Panum’s area of single binocular vision (Fig. 7.2). Only within the Panum’s area is the retinal disparity small enough to allow the images to be fused into one unified perception. The retinal periphery with its larger receptive fields is able to fuse images with a larger disparity and therefore the Panum’s area is larger in the peripheral visual field and smaller centrally. It is only within the limited space of the Panum’s area that we can see single. Objects outside of the Panum’s area fall on retinal points that are too disparate to be fused and are seen as double.
The horopter and Panum’s area are not static but are continually created and recreated with every change in direction of gaze or point of focus. For example, a person looking at a clock on the wall in the distance will create a Panum’s area of single binocular vision around the clock. If a person extends their arm and holds their hand out in between themselves and the clock, while focusing on the clock, their hand is seen as double since it lies outside Panum’s area. If the focus is shifted to their hand, a Panum’s area is created around their hand. The hand will be seen as single and the clock will be seen as double as the clock on the wall now lies outside of the Panum’s area of single binocular vision. Normally, the brain intervenes to prevent diplopia that occurs with objects outside of the Panum’s area from reaching consciousness. Occasionally, children become aware of this diplopia from objects seen outside Panum’s area. This is referred to as physiologic diplopia.
![]() FIGURE 7.1. The horopter. Point F is the fixation point. Point A lies on the horopter and therefore the retinal images will fall on corresponding retinal points. |
In order to have single binocular vision, the images produced by both eyes must be fused into a single unified perception. This fusion of the images occurs at a cortical level. Fusion has both a sensory and motor component. It is the motor component of fusion that reduces the horizontal, vertical, or torsional disparity of the retinal images making it possible for the images to fuse into a single perception. The motor component of fusion helps maintain ocular alignment and prevent diplopia. A phoria is an example of motor fusion maintaining ocular alignment. If the amount of the phobia exceeds the abilities of motor fusion, a manifest strabismus or tropia will occur. The eye movements produced by motor fusion to maintain ocular alignment are referred to as vergence movements. It is possible to have fusion with both its sensory and motor components without measurable stereopsis.
Not all individuals will have binocular vision. In order to develop binocular vision, an infant must be capable of seeing with each eye, and the eyes must be aligned, allowing retinal images to project onto corresponding retinal elements during the critical period of binocular vision development (5). This critical period of binocular development is generally accepted to be the first 2 years of life. If binocular vision never develops, this individual will be monocular, viewing with one eye at a time. A monocular patient is incapable of using both eyes simultaneously. It is not possible for a monocular patient to experience diplopia. A monocular patient will not have fusion and therefore will not have the motor component of fusion or vergence movements to help maintain ocular alignment. There are sensory tests that can determine if the patient has monocular or binocular vision (simultaneous perception).
VISUAL SYMPTOMS IN STRABISMUS
A strabismic patient who is monocular is not capable of simultaneous perception and will not experience diplopia. In patients with binocular vision a newly acquired strabismus will produce diplopia. The diplopia results from the simultaneous perception of the images of the object of regard projecting onto noncorresponding retinal points. These noncorresponding retinal points will localize the object to two different areas in space and the object will be seen as doubled. In strabismic eyes two different objects in space that would normally fall on noncorresponding retinal points can fall onto corresponding retinal points. Both of these objects could be localized to the same area in space and would be perceived as two different objects in the same place in space (5). This is referred to as visual confusion. This differs from diplopia, which is one object being seen in two places. When adult patients acquire a new strabismus, they complain of diplopia, an object located in two places at once. They do not complain of seeing one object on top of another, or two objects in one place (visual confusion). Visual confusion is not clinically significant.
Visual confusion does not exist for all portions of the visual field (5). Only similar images projecting on the foveas are perceived simultaneously. From the moment an eye deviates, a macula scotoma exists in one eye. This facultative macula scotoma will occur immediately preventing central confusion. This facultative macula scotoma occurs in all strabismic patients and can be plotted using a binocular perimetric technique (5). The facultative macula scotoma is not an adaptation that slowly develops over time, it occurs immediately in strabismus and is not a suppression scotoma.
CORTICAL ADAPTATIONS IN STRABISMUS
Adaptations can occur to the binocular vision system, which eliminate central and peripheral diplopia in acquired strabismus. These adaptations will only occur only in patients who have preexisting binocular vision. These cortical adaptations that develop gradually over time are suppression and anomalous retinal correspondence (ARC). Suppression and ARC are adaptations that occur in the brain to prevent diplopia from reaching consciousness. Suppression develops to prevent central diplopia. ARC develops to prevent peripheral diplopia. Patients who are monocular are incapable of simultaneous perception (diplopia) and will not develop suppression or ARC. The time needed to develop suppression and ARC is related to the age of the patient. The younger the child, the faster these adaptations will develop.
Suppression is a positive inhibitory reflex (5) that permits the cortex to ignore the images of conscious regard (what is seen centrally in the fixing eye) produced by retinal elements outside the macula in the deviated eye. Unlike the facultative scotoma that occurs in the macula to prevent central confusion, suppression is pathologic and requires time to develop. Suppression only exists when viewing with both eyes. The moment one eye is closed, the suppression scotoma disappears. If either eye can maintain fixation, the suppression scotoma can alternate between eyes, being present in the eye that is nonfixating at that instant. A suppression scotoma will not produce amblyopia because a suppression scotoma involves extramacular retina. It is the facultative macula scotoma that occurs immediately when an eye deviates that causes strabismic amblyopia, not suppression (5).
A suppression scotoma can be measured under binocular conditions with prism or binocular perimetry. In esotropic patients, there is a suppression scotoma nasally of approximately 5 degrees (Fig. 7.3). In exotropia there is a larger hemiretinal scotoma extending to the number of degrees required to include the object of conscious regard (Fig. 7.4). In a strabismic patient with suppression, increasing amounts of prisms can be added until the patient reports diplopia. In an esotropic patient, the amount of prism will generally be smaller than the angle of the esotropia. In an exotropic patient the amount of base-in prism needed to move the image of the object of conscious regard off the suppression scotoma will be slightly greater than the angle of the exotropia. Once the image has been moved off the suppression scotoma, the patient will report diplopia. The patient who reports diplopia once the image of conscious regard has been moved off the suppression scotoma has binocular vision and is capable of having either single binocular vision or diplopia after strabismus surgery. A strabismic patient who does not have diplopia when the strabismus is overcorrected with prism is most likely monocular and not expected to be capable of binocular vision and therefore should not be at risk for diplopia after strabismus surgery.
ARC is a cortical adjustment in a strabismic patient of the normal directional values of the extramacular retinal elements of the nonfixing eye to prevent peripheral diplopia (5). ARC exists only under conditions of binocular viewing. Immediately upon closing the fixing eye the visual direction of the retinal elements of the deviated eye with ARC return to their original directional values.
Suppression and ARC occur at a cortical level and can change rapidly (5). In an A or V pattern strabismus, as the patient changes from down gaze to up gaze, the angle of the horizontal strabismus is continually changing. In a patient with suppression and ARC, each time the angle of the strabismus changes, the suppression scotoma and ARC (visual direction of the extramacular elements) will change. Suppression and ARC are adaptations to prevent diplopia. They do not have an associated motor component. They do not provide vergence amplitudes to help maintain ocular alignment as seen with the motor component of fusion. When the angle of a strabismus changes, a patient with ARC and suppression has a sensory response, rather than a motor response, to prevent diplopia. A new suppression scotoma and ARC pattern will develop to accommodate the new angle of the strabismus. In patients with ARC there is no measurable stereopsis.
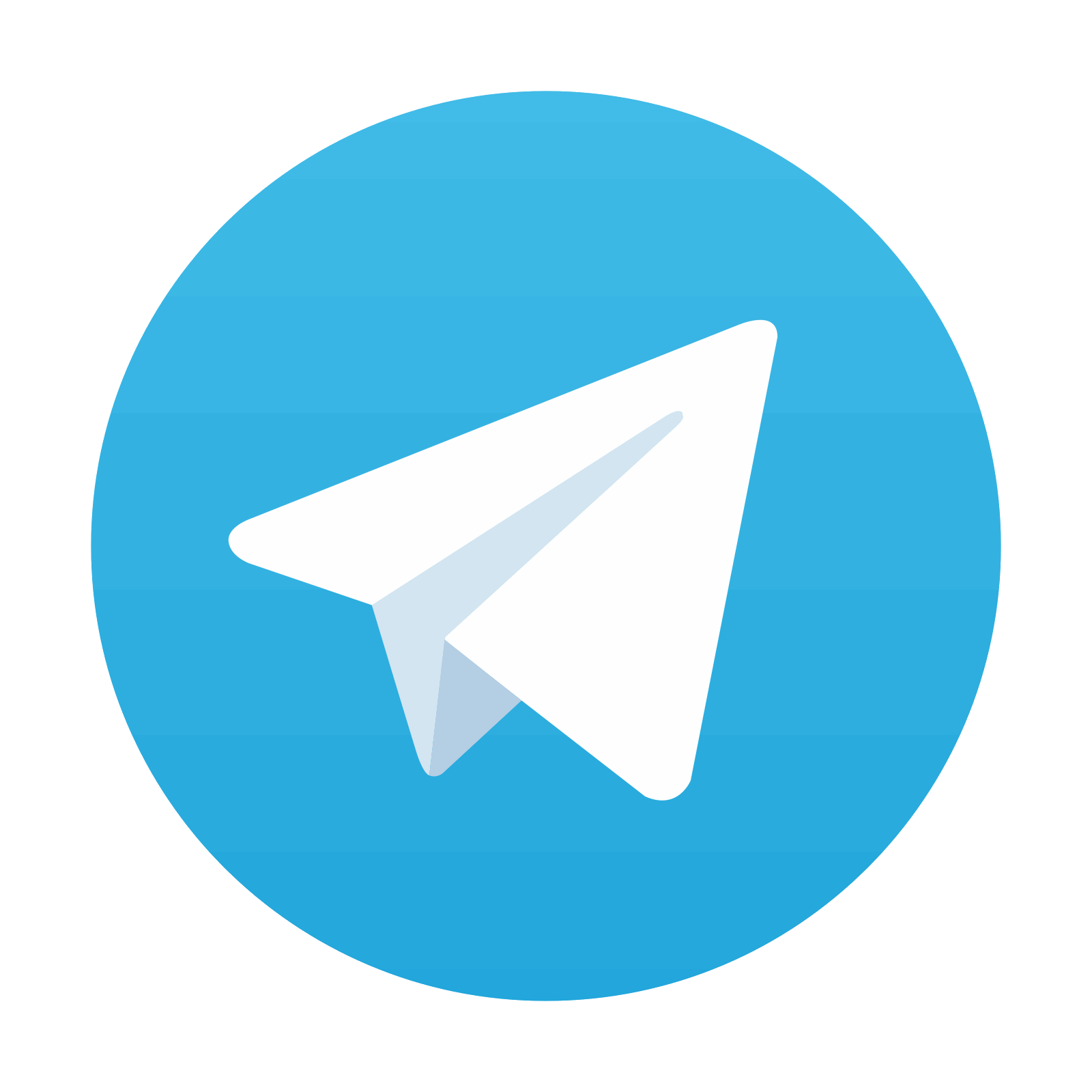
Stay updated, free articles. Join our Telegram channel

Full access? Get Clinical Tree
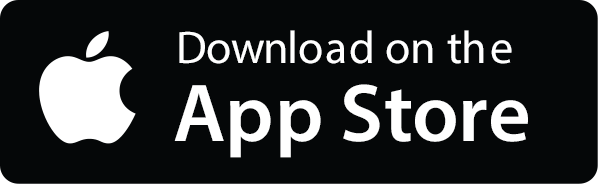
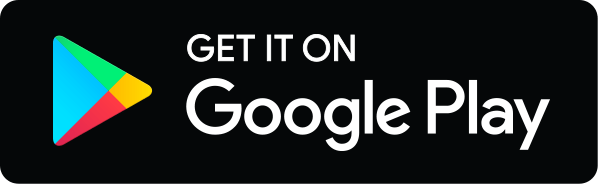