20
Auditory Brainstem Implants
Eric G. St. Clair, John G. Golfinos, and J. Thomas Roland Jr.
The auditory brainstem implant (ABI) was developed in 1979 at the House Ear Institute (Los Angeles, CA) for patients deafened by the bilateral vestibular schwannomas of neurofibromatosis type 2 (NF2). Patients with NF2 typically are left without functioning cochlear nerves bilaterally, either as a result of surgery or of the tumors themselves, and thus derive no benefit from cochlear implants. The ABI was designed to effectively bypass this disconnection and directly stimulate the cochlear nucleus in the brainstem in response to auditory stimuli.
♦ History of Development of Auditory Brainstem Implants
Neurofibromatosis type 2 (formerly “central neurofibromatosis”) was first described by Wishart in 1822 and is characterized by bilateral acoustic neuromas, multiple meningiomas, spinal ependymomas, and postcapsular cataracts. Inheritance is autosomal dominant through a gene on chromosome 22 (Miyamoto et al, 1990) though 50% of all cases represent sporadic mutations and the incidence is 1 in 40,000 births (Otto et al, 2000). The Gardner and Wishart forms of NF2 represent subtypes of lesser and greater severity respectively. The more severe form tends to present at a younger age and with more widespread central nervous system (CNS) tumors. Unlike unilateral acoustic neuromas, which typically are well encapsulated, the tumors of NF2 tend to diffusely infiltrate adjacent nerves (Brackmann et al, 1993). Patients with NF2 commonly end up with total hearing loss by the time they are in their 20s or 30s (Ramsden et al, 2005).
The bilateral acoustic neuromas (or more accurately “vestibular schwannomas”) of NF2 tend to damage both the vestibular and cochlear components of the eighth cranial nerve (Kanowitz et al, 2004). These nerves are generally sacrificed at surgery; thus, patients with NF2 are not candidates for conventional cochlear implants as these devices require an intact cochlear nerve. The ABI was designed to bypass this disconnection by directly stimulating the cochlear nucleus in the brainstem. Through the pioneering work of Drs. House and Hitselberger, the first ABI device was implanted in a human recipient in 1979.
The hardware design of the ABI is similar to that of a cochlear implant, except that the target is the cochlear nucleus within the brainstem rather than the scala tympani within the cochlea. As with a cochlear implant there are both external and internal components. Externally, a modified hearing aid receives auditory analog signals and transmits them to a miniaturized speech processor. The speech processor analyzes, filters, and digitizes the signals and then sends them to the transmitting coil. The transmitting coil in turn sends the coded signals as radio signals to the implant under the skin. Internally, the ABI delivers electrical signals to the array of platinum electrodes on the cochlear nucleus on the dorsolateral surface of the brainstem. The resulting information regarding intensity and pitch can then be relayed within the central auditory system as sound (Otto et al, 2002): “The sound processing unit (speech processor) requires appropriate programming and must be fitted to individual users. Programming speech processors involves psychophysical assessment of electrically induced auditory percepts, including threshold, comfort level, and pitch. These measures are programmed into the processor and used to control the amplitude and the sequential patterns of stimulation. In multichannel auditory implants, different sites of stimulation can generate different pitch percepts for the listener. Changes in frequency spectrum of sound can therefore be coded by appropriate changes in the patterns of electrode activation.” The speech processor and individual electrodes essentially take over the role of the cochlea as a spectrum analyzer, decomposing complex sounds into their frequency components (Kuchta et al, 2004; Otto et al, 2002; Schwartz et al, 2003).
The original design by House and Hitselberger was a simple pair of ball electrodes implanted into the substance of the cochlear nucleus of a single patient. The cochlear nucleus was chosen as the site of implantation both because it is the first neuronal junction in the auditory pathway beyond the cochlear nerve and because it is relatively accessible at surgery. Two years after this first implantation, the electrode was noted to have migrated, resulting in loss of auditory sensation and the development of lower extremity paresthesias. The ABI was subsequently replaced by a new implant designed for surface placement over the cochlear nucleus. This new single-channel ABI was made of two platinum plates on a Dacron mesh carrier (the mesh facilitated development of a fibrous capsule around the implant that impeded migration). It remains functional to this day. Subsequent developments in silicon backing, Dacron mesh, and nonelastic wires have improved “conformal adherence” to the brainstem surface. Postmortem studies by Otto’s group confirmed ingrowth of connective tissue into the fabric mesh electrode carrier. Potential damage to surrounding neural structures is minimized by use of a charge-balanced biphasic waveform and by ensuring that charge density does not exceed 20 microcoulomb per square centimeter per phase (Brackmann et al, 1993; Kovacs et al, 2000; McElveen et al, 1985; Otto 2000; Otto et al, 2002).
By 1991, the number of electrodes in the standard ABI was increased to three. The most important aspect of this development was the finding that sound frequency (pitch) varies with electrode location. Furthermore, studies of cochlear implants had demonstrated better speech recognition as a result of spectral (frequency) information with multichannel devices. Subsequently, in 1995, Laszig and colleagues developed the first multichannel ABI consisting of an array of eight platinum disk electrodes in 1991. A multicenter clinical trial of a multichannel ABI began in the United States in 1994. The ABI in current use in the United States is a multichannel implant with 21 electrodes arranged in three rows. The array is small enough to fit within the confines of the lateral recess but large enough such that the individual electrodes do not exceed the critical values of charge density that could damage neural tissue. As many of these patients will require magnetic resonance imaging (MRI) follow-up for tumors of NF2, the receiver-stimulator portion contains a removable magnet. Postoperatively, the patient’s pitch perception can be adjusted by manipulating the sites of electrode stimulation. However, at least one recent study found that beyond a critical number—perhaps seven—there is limited usefulness in the increased number of electrodes. The principal limitation of the surface electrode array of the ABI is the tonotopic organization of the cochlear nucleus itself. This tonotopic organization is perpendicular to the surface of the brainstem, thus limiting the utility of the surface electrode array. A penetrating electrode array is under development to better access this three-dimensional tonotopic organization of the cochlear nucleus. Rauschecker and Shannon (2002) believe that advances in neuroimaging (e.g., functional MRI) and stereotactic neurosurgical techniques will allow better access to small, deep brainstem structures and thus better tonotopic representation by a penetrating electrode. Of note, Colletti et al (2005) believe that the tonotopic mismatch between electrode and nucleus (and thus the limited number of electrodes that can be used) is not an issue in nontumor ABI recipients as they lack the significant anatomic and functional distortion of brainstem structures (Brackmann et al,1993; Kanowitz et al, 2004; Koch et al, 2005; Kuchta et al, 2004; McCreery et al, 1990; Otto et al, 2000).
♦ Indications for Auditory Brainstem Implants
The ABI was first developed for patients with deafness resulting from the bilateral acoustic neuromas of NF2. Histologic studies demonstrated that these tumors, although usually arising from the vestibular component of the eighth nerve, typically invade rather than compress the nerve to the point ofbeingnonfunctional(Miyamoto etal,1990).Withoutafunctioning cochlear nerve (whether from the tumor itself or from the subsequent transection at surgery) these patients were not candidates for cochlear implantation.
In the U.S., the criteria for implantation are (1) age greater than 12 years old, (2) English speaking, (3) highly motivated to participate and comply with protocol follow-up, (4) reasonable expectations, (5) bilateral vestibular schwannomas of NF2, and (6) psychologically stable (Kanowitz et al, 2004; Otto et al, 2002). Previous irradiation of acoustic neuromas does not preclude ABI placement but in at least one series was hypothesized to have accounted for delayed deterioration of ABI utility (Grayeli et al, 2003; Laszig et al, 1995).
More recently at centers outside the United States, ABIs have been implanted in patients without NF2 but who are similarly unable to undergo cochlear implantation. These indications have included cochlear ossification (a common complication of meningitis), severe auditory neuropathy (a multiform disorder characterized by cochlear nerve degeneration and subsequent sensorineural hearing loss, with word recognition reduced out of proportion to pure tone threshold), trauma/cochlear nerve avulsion, cochlear nerve aplasia, and severe cochlear malformation. Notably, these patients typically do not have the extensive distortion of brainstem anatomy that is commonly associated with the often large tumors of NF2 patients. This lack of distortion facilitates placement of the ABI in the nontumor patients (Colletti et al, 2002, 2004, 2005; Grayeli et al, 2003; Kuchta et al, 2004).
The use of the ABI in nontumor patients has meant implantation in progressively younger patients. Colletti et al (2005) have implanted ABIs in nontumor, congenitally deafened patients as young as 14 months old. Ramsden et al (2005) state, “The auditory pathway requires stimulation with sound in the first few years of life if it is going to be programmed for future speech perception and consequent language production…. By the age of four years, and certainly by the age of six years, the auditory pathways are losing their plasticity and poorer long-term outcomes are to be expected.”
The importance of early stimulation of the auditory system was also demonstrated in a recent report of two patients with congenital deafness in one ear and the subsequent development of a vestibular schwannoma in the other ear. Both patients failed initial attempts at placing a cochlear implant on the congenitally deafened side once the contra-lateral vestibular schwannoma was discovered. Ramsden et al (2005) hypothesized that the lack of stimulation of the first-order auditory neuron had left the spiral ganglion cells unable to effectively transmit auditory signals. Alternatively, or additionally, there may have been a loss of cell bodies in the cochlear nucleus as a result of lack of ipsilateral stimulation. They point out that in rodent studies, removal of the cochlea before day 12 (when hearing begins) results in death of neurons in the cochlear nucleus. Both patients subsequently underwent placement of an ABI on the side of tumor removal.
♦ Surgical Procedure for Auditory Brainstem Implants
Initially, ABIs were implanted after the removal of the second vestibular schwannoma in a patient with NF2. More recently, it has become relatively common to place the ABI following resection of the first tumor, although benefit from the device will not be noted until removal of the contralateral tumor. Brackmann et al (1993) outlined three reasons for implanting the ABI at first side tumor resection in NF2: (1) to afford the patient experience with the device prior to losing all hearing; (2) to decrease anatomic distortion at the time of implantation; and (3) to retain the option of implanting on the contralateral side should initial implantation prove unsuccessful. Regardless of whether the ABI is placed after removal of the first or second tumor, it is almost always implanted at the first operation on a given side. Implantation at reoperation is extremely difficult as a result of fibrosis in the area of previous resection (Grant et al, 2000). More recently, the U.S. Food and Drug Administration (FDA) has granted permission for implantation in patients with previous bilateral tumor resections.
Historically, the implantation of the ABI has been achieved through a standard translabyrinthine approach (Grayeli et al, 2003; Kanowitz et al, 2004; Kuchta et al, 2004; Otto et al, 2002). The proposed advantages of this approach include direct access to the lateral recess of the fourth ventricle and the surface of the cochlear nucleus complex, minimal cerebellar retraction, early identification of the facial nerve, complete tumor removal from the lateral end of the internal auditory canal, and low rates of morbidity (Otto et al, 2002; Vincent et al, 2002). Although this remains the only approach approved by the FDA, other approaches, including retrosigmoid and subtonsillar, have been used internationally.
Colletti et al (2002) proposed the advantages of a retrosigmoid approach to be: (1) short duration procedure (no extensive drill-out); (2) no risk of intracranial contamination from broaching the mastoid air cells; (3) possible preservation of hearing for some patients, which they propose as the main advantage; and (4) stabilization of the implant by placing the wire in a drilled-out groove in the posterior wall of the petrous temporal bone which may help prevent postimplantation migration. Colletti et al (2005) and Seki et al (2003) advocated subtonsillar placement of the electrode by extending the conventional retrosigmoid craniotomy with foramen magnum opening and C1 laminectomy. They proposed the following advantages of the wider exposure obtained through this subtonsillar (or “cerebellomedullary fissure”) approach: (1) it may be better for placing penetrating “depth” electrodes; (2) it is not affected by adhesions from previous surgery, thus making a second operation for ABI feasible; and (3) if necessary, it is possible to do bilateral placement of electrodes by cutting both taenia and then raising both tonsils.
Regardless of the approach used, the goal is to place the electrode array directly on the surface of the cochlear nucleus complex on the dorsolateral surface of the brainstem just rostral to the pontomedullary junction (McElveen et al, 1987). The cochlear nucleus is composed of three subnuclei: the dorsal, superior, and inferior ventral cochlear nuclei. Placement over the dorsal component is believed to result in fewer nonauditory stimuli than over the ventral component (Brackmann et al, 1993; Colletti et al, 2002). Unlike in lower vertebrates, the cochlear nucleus is not visible on gross or microscopic inspection. As such, surface landmarks are essential for correct placement of the ABI. These landmarks include the stump of the transected eighth cranial nerve, the flocculus of the cerebellum, cranial nerves seven and nine, the choroid plexus, and the tenia (the attachment of the tela choroidea to the floor of the lateral recess) of the foramen of Luschka. The dorsal cochlear nucleus lies almost entirely within the lateral recess (foramen of Luschka) of the fourth ventricle; optimal results have been achieved by placing the ABI completely within the lateral recess. This can often be aided by identifying and then transecting the tenia as it overlies the lateral extent of the lateral recess. The tenia crosses the ventral cochlear nucleus (VCN) just medial to the boundary of the VCN and the eighth nerve. Although this relationship is fairly constant, the tenia can be difficult to identify. Identification of the tenia can be aided by finding the apex of the angle between the eighth and ninth cranial nerves (Monsell et al, 1987; Otto et al, 2002; Terr and Edgerton, 1985; Terr et al, 1990; Vincent et al, 2002).
Unfortunately, these anatomic landmarks are often significantly distorted by the often large tumors of patients with NF2. In the minority of patients who do not receive auditory sensation after implantation, anatomic difficulties at surgery have been thought usually to be the cause. In the case of reoperation, gliosis can further impede cochlear nucleus identification. Several electrophysiologic tests, including electrically evoked auditory brainstem responses (EABR), near field potential (NFP), neural response telemetry (NRT), and stimulation with a bipolar probe, can assist in determining the correct location of the cochlear nucleus complex. EABR is a far-field measurement of brainstem activity. Stimulation of the cochlear nucleus in EABR should reveal either mono-or biphasic wave patterns that approximately correlate with waves III and V on traditional ABR tracings. Improper placement of the electrode array can be detected with EABR by subsequent intraoperative cranial nerve stimulation, myogenic responses, vital sign changes, or absent EABR waveforms. NFP is a near field measurement at the cochlear nucleus (Zimmerling et al, 2000). The bipolar probe consists of a circuit board with four gold-plated electrodes that correlate identically with four of the actual ABI electrodes. The electrodes can be stimulated across several electrode pair combinations just like the ABI (Roland et al, 2000). Correct placement in the lateral recess can also be confirmed by noting cerebrospinal fluid (CSF) egress from the foramen of Luschka following a Valsalva maneuver induced by the anesthesiologist. Finally, orienting the electrode array such that the electrodes face medially and superiorly maximizes stimulation of the cochlear nucleus. Intraoperative monitoring of cranial nerves five, seven (orbicularis oris), nine, and ten (ipsilateral pharyngeal muscles) is also essential (Colletti et al, 2002; Colletti et al, 2004; Kanowitz et al, 2004; Kuchta et al, 2004; Laszig et al, 1995; Otto et al, 2000; Toh and Luxford 2002; Vincent et al, 2002; Waring 1992).
Friedland and Wackym (1999) advocated the use of an endoscope to aid in visualizing the lateral recess at surgery. In cadaver studies, the 30-degree endoscope was found to be helpful in translabyrinthine and retrosigmoid approaches to the lateral recess and essential to the middle fossa approach. With the translabyrinthine approach, the endoscope allowed visualization anterior to the flocculus and choroid plexus prior to any retraction. This allowed early visualization of the eight and ninth cranial nerve root entry zones while preserving the delicate tenia. Moreover, the foramen of Luschka can be distinguished from similar structures by direct visualization into its lateral recess with the endoscope. Minimal retraction of the cerebellum and flocculus was also found to be the major advantage of the use of the endoscope with the retrosigmoid approach. The endoscope was found to be essential for the rarely used middle fossa approach, although the risk of injury to the facial nerve was thought to be significant.
♦ Postoperative Care and Complications of Auditory Brainstem Implants
Initial stimulation typically occurs approximately 6 weeks after surgery. Awareness of the consequences of vagal nerve stimulation and significant nonauditory side effects is essential. Bradycardia, motor long tract stimulation, vertigo, throat tightening, and fainting might occur during initial stimulation. For this reason, cardiac monitoring and physician attendance were part of the early protocol. The programming audiologist is always aware of the symptoms and can immediately “program out” untoward stimuli. The speech processor of the ABI is then programmed with respect to various auditory precepts including threshold, loudness, comfort level, pitch, and reduction of nonauditory sensations. The initial stimulus occurs over a 3-day period lasting several hours per day. The process is generally more complicated than for cochlear implant patients for the reasons outlined by Otto and Staller (1995): (1) presence of nonauditory sensations, (2) the more central locus of stimulation, (3) the uncertainty and irregularity of tonotopic stimulation, and (4) potential central disease from NF2. Moreover, fitting is a dynamic process that requires periodic adjustments to account for small changes in auditory perception over time (Colletti et al, 2002; Kanowitz et al, 2004; Kuchta et al, 2004; McElveen et al, 1985; Otto 2000; Otto et al, 2001; Schwartz et al, 2003).
In multichannel auditory brainstem implants, different sites of electrode stimulation can generate different pitch percepts. Changes in the frequency spectrum of sounds can thus be coded by changes in the patterns of electrode activation. The system is aided by the ability to stimulate between any pair of electrodes on the array (bipolar mode) or by the use of a remote electrode as a ground (monopolar mode). Use of the monopolar mode often allows stimulation at lower current levels and with fewer nonauditory sensations. Pitch scaling and ranking are two procedures used to determine the appropriate tonotopic order of the electrodes. With pitch scaling, electrodes are simulated and patients are asked to assign the sound a “sharpness rating” between one (lowest pitch) and 100 (highest pitch) (Colletti et al, 2002, 2005; Otto et al, 1998). With pitch ranking, two electrodes are stimulated in succession and the patient is asked to determine which sound has the higher pitch. Based on these tests, an appropriate tonotopic order of the electrodes can be determined. Unlike in cochlear implants where tonotopic order is fairly constant from patient to patient, tonotopic order in ABIs can be highly variable across patients. In Coletti’s group, the most medial and caudal electrodes tended to elicit higher frequencies. Otto et al similarly found higher frequencies associated with the more medial electrodes, whereas Vincent et al found that in three of five testable patients low frequencies were found dorsally and high frequencies were found ventrally. According to Kuchta et al (2004), however, the appropriate ranking of the pitch was of greater importance than the absolute number of electrodes used. Furthermore, according to Otto et al (1995), the ability to pitch rank is believed to correlate with improved speech recognition. The ability to pitch scale and rank may develop over time and should be tested at each follow-up even if the patient is unable to do this on initial tuning (Vincent et al, 2002).
The Nucleus ABI 22 (Cochlear Corporation, Sydney, Australia) (eight electrodes) uses the spectral peak (SPEAK) speech processing strategy. The Nucleus ABI 24 (21 electrodes) accommodates SPEAK as well as Advanced Combination Encoder (ACE) and continuous interleaved sampling (CIS) encoding strategies. “The SPEAK strategy delivers the signal at …250–300 pulses/sec and selects the number and location of the electrodes to be stimulated depending on the intensity and frequency of the incoming signal. The CIS strategy presents high fixed rates of stimulation (600 to 1800 pulses/sec) to a small number of channels. The ACE strategy combines the advantages of both SPEAK and CIS strategies by using a high rate of stimulation with dynamic electrode selection and a large number of available electrodes” (Colletti et al, 2005).
The primary postoperative complications are CSF leak, implant migration, and nonauditory stimuli. CSF leaks are treated with approximately 5 days of lumbar CSF diversion. Rarely is reoperation and leak repair necessary. Otto et al (2002) reported CSF leaks in two of 61 patients (3.3%). This percentage is comparable to those from other translabyrinthine craniotomies and was not directly attributable to electrode implantation. Roland’s group (Kanowitz et al, 2004) reported this complication in two of 18 (11%) patients. Implant migration or aberrant placement of the ABI at surgery can result in an increased number of nonauditory sensations. As discussed earlier, developments in silicon backing, Dacron mesh, and nonelastic wires have improved “conformal adherence” to the brainstem surface and thus decreased the complications of postsurgery implant migration (Kovacs et al, 2000). In the series reported by Kuchta et al (2004), only one of 61 patients “lost” more than one electrode over time.
Nonauditory stimuli develop in up to 42% of users (Otto et al, 1998, 2001, 2002; Vincent et al, 2002) and thus warrant special consideration. Nonauditory stimuli are almost always located ipsilateral to the side of implantation. The most common nonauditory sensations are dizziness and ipsilateral “tingling” (Colletti et al, 2005; Otto et al, 2001, 2004). Other sensations include “jittering” of the visual field (flocculus of the cerebellum), muscle twitches (cranial nerves VII and IX), and contralateral “tingling.” These side effects are usually considered to be minimal by the patient and often are rectifiable with adjustment of (usually increasing) the duration of the stimulus or by selecting a different ground electrode. In one series, reduction of nonauditory sensations was achieved by reducing the rate of stimulation; no effect was noted by changing stimulus duration (Vincent et al, 2002) Additionally, nonauditory sensations often decrease over time (Colletti et al, 2005; Kanowitz et al, 2004; Otto et al, 1998, 2002) whereas 9% of patients develop persistent nonauditory sensations (Otto et al, 2004).
These nonauditory stimuli appear to be related to placement low in, and with protrusion from, the lateral recess (Shannon et al, 1993). This aberrant placement allows current spread outside of the cochlear nucleus, potentially stimulating other cranial nerves, the flocculus of the cerebellum, the inferior cerebellar peduncle, and the spinothalamic tract. Keeping the electrode within the confines of the lateral recess would prevent this inappropriate stimulation (Otto et al, 2002). Nonauditory stimuli also tend to be associated with stimulation of the most medial and lateral electrodes (Otto et al, 2002; Toh and Luxford, 2002).
Rauschecker and Shannon (2002) believe that advances in neuroimaging (e.g., functional MRI) and stereotactic neurosurgical techniques will allow better access to small, deep brainstem structures and thus better tonotopic representation by a penetrating electrode.
♦ Results of Auditory Brainstem Implants
The effectiveness of the ABI over the past 25 years has been well studied. A typical follow-up protocol involves testing the patient every 3 months for the first year after the initial stimulation and then annually thereafter. A multi-institutional study in the United States found that overall 81% of implantees received auditory sensations. Results are similar to those of single-channel cochlear implants. The ABI appears to function best as a “lip-reading enhancer” and as a tool for detecting and differentiating different environmental sounds. With regard to the former, it is particularly useful in determining the rhythm, stress, timing, and intensity of speech. When combined with lip-reading cues, 93% of patients demonstrate improved sentence understanding at 3 to 6 months. Sound discrimination and identification are possible, but significant open-set word recognition is rare. In general, speech perception performance and subjective sound quality are better with the patients who received multichannel devices than in the original 25 patients who received single-channel implants (Brackmann et al, 1993; Colletti et al, 2004; Laszig et al, 1995; Lenarz et al, 2002; Otto et al, 2000, 2001, 2002; Ramsden et al, 2005; Roland et al, 2000; Schwartz et al, 2003; Toh and Luxford, 2002; Vincent et al, 2002).
One important finding is that patients gradually improve in their use of the ABI over time, with one study finding increasing benefit as late as 8 years after implantation (Otto et al, 2002; Schwartz et al, 2003; Toh et al, 2002). Additionally, a patient’s subjective evaluation of the ABI is generally higher than would be predicted by objective audiometric data (Lenarz et al, 2002). Testing of auditory sensation with visual cues improves the most over time, though auditory testing alone shows significant improvement as well. As a control, vision-only testing tends to stay stable over time (Otto et al, 2000).
Counseling, multidisciplinary rehabilitation, and auditory training are important postoperative adjuncts for all ABI recipients. Kanowitz et al (2004), Lenarz et al (2002), and Otto et al (2004, 2002) found that preoperative counseling with regard to personal motivation, reasonable expectations, and family support was of particular importance in teenage patients. This counseling should stress several points: (1) some patients do not receive auditory sensations; (2) the ABI does not provide normal sound quality; (3) most ABI patients do not achieve open-set speech recognition; (4) regular follow-ups are required for speech processor optimization; and (5) it will take time and experience to develop maximum benefit from the ABI.
Otto et al (2002) reported their results in 61 patients implanted with an eight electrode ABI for NF2. Six of 61 (9.8%) patients did not report useful auditory sensations, though one of these patients subsequently derived benefits from a contralaterally placed ABI. The authors found that their patients often were initially disappointed with the “muffled” sounds from the new ABI. However, most patients ultimately reported significant improvement in sound quality and performance following a period of adaptation and learning. Motivation and persistence, particularly in the first 3 months postimplantation, were noted to be of particular importance for long-term improvement.
The Sound Effects Recognition Test (SERT) test for environmental sound discrimination was at or above the 50% correct level for most of the 61 patients. As with other tested parameters of ABI function, scores on this test improved with experience. On Monosyllable Trochee Spondee Test (MTS) testing, 87% of patients scored above chance on closed-set word identification and 98% scored above chance on syllable stress identification. The Northwestern University Children’s Perception of Speech (NU-CHIPS) test is a closed-set sound-only test wherein the patient must choose the correct word from a list of four rhyming monosyllable words. On this test 84% of ABI recipients in this series scored significantly above chance.
Scores on vowel and consonant recognition were overall less impressive, although a few patients were able to perform at high levels. Moreover, the investigators found that to achieve sound-only speech recognition, the patient’s performance on the vowel and consonant tests has to approach 50%. A small but significant number of ABI patients were subsequently able to demonstrate some level of sound-only sentence recognition on the City University of New York (CUNY) sentence test.
Regular experience with the ABI is important for continued improvement. Regular users of the ABI with more than 2 years’ experience generally performed better on all speech perception tests than those with less experience. Additionally, MTS scores continued to improve as late as 8 years postimplantation. Some patients eventually developed some degree of open set speech recognition.
Otto et al (2000) found that 85% of patients receive some sort of auditory sensation. Lenarz et al (2002) found that all of their patients were able to differentiate speech from environmental sounds. Additionally, in that group 73% of patients were able to differentiate between adult’s and children’s voices, 64% were able to distinguish men’s and women’s voices, and 82% found the ABI to be “very useful” in differentiating environmental sounds (e.g., ringing telephone and barking dog). Two of the 11 regular ABI users developed “open-set” abilities and are able to converse on the telephone. The device appears to work best in quiet settings and when listening to a familiar voice; however, according to Lenarz et al (2002), 90% of patients found the device useful even with an unfamiliar voice.
Vincent et al (2002, 2000) reported their results with a 15-electrode device developed in France. Overall, 12 of 14 patients (86%) were able to achieve auditory sensations from their device. Eleven of 14 patients (79%) received auditory sensations at initial testing after translabyrinthine approach to device placement. One of these patients subsequently lost auditory sensation after 2 weeks. Three patients who underwent a retrosigmoid approach did not have auditory sensations at initial testing. Two of these patients were subsequently able to achieve auditory sensation after reoperation via a translabyrinthine approach. On reoperation, the electrode array was noted to be protruding from the lateral recess in both of these patients.
In Vincent’s group, nonauditory sensation occurred in five of the 12 testable patients (42%) and involved 12 electrodes (6.7%). The side effects included “dizziness” (67% of the non-auditory sensations), tingling of the arm or shoulder (17% of nonauditory sensations), and “facial stimulation” (16% of nonauditory sensations). In only one patient did auditory and nonauditory sensations (“dizziness”) emanate from the same electrodes. In this case the nonauditory sensation was eliminated by lowering the stimulation frequency. Changing the duration of stimulation had no effect on nonauditory side effects.
Twelve patients in Vincent’s group were able to detect the presence of environmental sound and on average scored 63% (range 0 to 100%) on open-set recognition of environmental sound. All patients with normal vision demonstrated improved lip-reading in sound plus vision mode when compared with vision alone. Three of nine patients (33%) scored at least 15% on sound only mode. One patient was able to converse on the telephone with people familiar to her.
Kanowitz et al (2004) reported that 17 of 18 (94%) patients had some auditory sensation at initial device stimulation. However, at 6-month follow-up one patient reported facial nerve twitching with loud noise stimulation. A subsequent computed tomography (CT) scan demonstrated that the electrode paddle had shifted to a more lateral position. Eleven of 18 patients use their ABIs daily for an average of 9.6 hours per day. Patients with the Nucleus 22 (eight electrode) implant demonstrated scores significantly greater than chance on MTS Word, MTS Stress, and NU-CHIPS (word recognition) testing. However, at subsequent follow-up, only the scores on MTS Word and MTS Stress demonstrated improvement. Patients with the Nucleus 24 (21 electrode) device similarly showed scores on MTS Word, MTS Stress, and NU-CHIPS that were significantly better than chance. The scores in the Nucleus 24 patients were considerably higher in all tests but, owing to large standard deviations, not significantly so. Neither device demonstrated open-set speech discrimination with the Central Institute for the Deaf (CID) sentence test. One of 18 patients (5.6%) developed a CSF leak. No patients limited their use because of nonauditory side effects.
Colletti et al (2005) reported their results with both tumor (NF2) and nontumor ABI recipients. In their study, all 29 patients (20 adults and nine children) received auditory sensations. At 1 year, the average adult closed-set auditory alone word recognition score was 86% (improved from 56.6% at 6 months) in nontumor patients and 24.5% (improved from 19.3%) in tumor patients. The average adult auditory alone sentence recognition score at 1 year was 63% (improved from 35% at 6 months) in nontumor patients and 12.1% (improved from 9.1%) in tumor patients. By 1 year, seven nontumor adult patients and three tumor adult patients were able to achieve some speech-tracking (improved from three and one patients at 6 months, respectively). The better results in the nontumor patients are hypothesized to be related to the significant anatomic and functional distortion caused by tumors compressing the brainstem. The authors further report that progress in children takes place “at an almost exponential rate” in the first few months after activation.
Grayeli et al (2003), in a case report of a patient deafened by pneumococcal meningitis, similarly found that “the overall performances of ABI are similar to, and in some cases better than, those reported for cochlear implants in totally ossified cochleae.”
♦ Conclusion
Because of the pioneering work of Drs. House and Hitselberger, about 200 patients have received auditory brainstem implants. These modified cochlear implants allow more proximal stimulation of the auditory system for patients without functioning cochlear nerves. Although originally developed exclusively for patients with neurofibromatosis type 2, ABIs have more recently been implanted for several other causes of bilateral hearing loss. Appropriate placement of the ABI within the confines of the lateral recess of the fourth ventricle allows the best stimulation of the cochlear nucleus complex in the brainstem and decreases nonauditory sensations. The device works best as a lip-reading enhancer, although a small minority of patients achieves some level of open-set speech recognition. Improved access to the tonotopic gradient of the cochlear nucleus, perhaps through the development of a penetrating electrode array, may lead to improvements in ABI sound quality.
References
Brackmann DE, Hitselberger WE, Nelson RA, et al. (1993). Auditory brainstem implant: I. Issues in surgical implantation. Otolaryngol Head Neck Surg 108:624–633
Colletti V, Carner M, Miorelli V, Guida M, Colletti L, Fiorino F. (2004). Cochlear implant failure: Is an auditory brainstem implant the answer? Acta Otolaryngol 124:353–357
Colletti V, Carner M, Miorelli V, Guida M, Colletti L, Fiorino F. (2005). Auditory brainstem implant: new frontiers in adults and children. Otolaryngol Head Neck Surg 133:126–138
Colletti V, Fiorino F, Carner M, Miorelli V, Guida M, Colletti L. (2004). Auditory brainstem implant as a salvage treatment after unsuccessful cochlear implantation. Otol Neurotol 25:485–496
Colletti V, Fiorino F, Carner M, Sacchetto L, Miorelli V, Orsi A. (2002). Auditory brainstem implantation: the University of Verona experience. Otolaryngol Head Neck Surg 127:84–96
Colletti V, Fiorino F, Carner M, et al. (2002). Hearing restoration with auditory brainstem implant in three children with cochlear nerve aplasia. Otol Neurotol 23:682–693
Friedland DR, Wackym PA. (1999). Evaluation of surgical approaches to endoscopic auditory brainstem implantation. Laryngoscope 109:175–180
Grant IL, Hall BB, Welling DB. (2000). Cochlear implantation in neurofibromatosis type 2. In: Waltzman SB, Cohen NL, eds. Cochlear Implants, pp. 367–368. New York: Thieme
Grayeli AB, Bouccara D, Kalamarides M, et al. (2003). Auditory brainstem implant in bilateral and completely ossified cochleae. Otol Neurotol 24:79–82
Kanowitz SJ, Shapiro WH, Golfinos JG, Cohen NL, Roland TJ Jr. (2004). Auditory brainstem implantation in patients with neurofibromatosis type 2. Laryngoscope 114:2135–2146
Koch DB, Staller S, Jaax K, Martin E. (2005). Bioengineering solutions for hearing loss and related disorders. Otolaryngol Clin North Am 38:255–272
Kovacs R, Janka M, Hochmair-Desoyer I, Helms J, Roosen K, Hochmair E. (2000). A new electrode design for the stable placing of a brainstem electrode. In: Waltzman SB, Cohen NL, eds. Cochlear Implants, pp. 368–369. New York: Thieme
Kuchta J, Otto SR, Shannon RV, Hitselberger WE, Brackmann DE. (2004). The multichannel auditory brainstem implant: how many electrodes make sense? J Neurosurg 100:16–23
Laszig R, Sollmann WP, Marangos N. (1995). The restoration of hearing in neurofibromatosis type 2. J Laryngol Otol 109:385–389
Lenarz M, Matthies C, Lesinski-Schiedat A, et al. (2002). Auditory brainstem implant part II: subjective assessment of functional outcome. Otol Neurotol 23:694–697
McCreery DB, Agnew WF, Yuen TGH, Bullara L. (1990). Charge density and charge per phase as cofactors in neural injury induced by electrical stimulation. IEEE Trans Biomed Eng 37:996–1001
McElveen JT Jr, Hitselberger WE, House WF. (1987). Surgical accessibility of the cochlear nuclear complex in man: surgical landmarks. Otolaryngol Head Neck Surg 96:135–140
McElveen JT Jr, Hitselberger WE, House WF, Mobley JP, Terr LI. (1985). Electrical stimulation of cochlear nucleus in man. Am J Otol Suppl:88–91.
Miyamoto RT, Campbell RL, Fritsch M, Lochmueller G. (1990). Preservation of hearing in neurofibromatosis 2. Otolaryngol Head Neck Surg 103:619–624
Monsell EM, McElveen JT Jr, Hitselberger WE, House WF. (1987). Surgical approaches to the human cochlear nuclear complex. Am J Otol 8:450–455
Otto SR, Brackmann DE, Hitselberger WE. (2004). Auditory brainstem implantation in 12-to 18-Year Olds. Arch Otolaryngol Head Neck Surg 130:656–659
Otto SR, Brackmann DE, Hitselberger WE, Shannon RV. (2001). Brainstem electronic implants for bilateral anacrusis following surgical removal of cerebellopontine angle lesions. Otolaryngol Clin North Am 34:485–499
Otto SR, Brackmann DE, Hitselberger WE, Shannon RV, Kuchta J. (2002). Multichannel auditory brainstem implant: update on performance in 61 patients. J Neurosurg 96:1063–1071
Otto SR, Ebinger K, Staller SJ. (2000). Clinical trials with the auditory brainstem implant. In: Waltzman SB, Cohen NL, eds. Cochlear Implants, pp. 357–366. New York: Thieme
Otto SR, Shannon RV, Brackmann DE, Hitselberger WE, Staller S, Menapace C. (1998). The multichannel auditory brain stem implant: Performance in twenty patients. Otolaryngol Head Neck Surg 118:291–303
Otto SR, Staller SJ. (1995). Multichannel auditory brain stem implant: case studies comparing fitting strategies and results. Ann Otol Rhinol Laryngol 104(suppl):36–39
Ramsden R, Khwaja S, Green K, O’Driscoll M, Mawman D. (2005). Vestibular schwannoma in the only hearing ear: cochlear implant or auditory brainstem implant? Otol Neurotol 26:261–264
Rauschecker JP, Shannon RV. (2002). Sending sound to the brain. Science 295:1025–1029
Roland JT Jr, Fishman AJ, Cohen NL. (2000). Bipolar stimulating probe for cochlear nucleus localization in auditory brainstem implant surgery. In: Waltzman SB, Cohen NL, eds. Cochlear Implants, pp. 373–377. New York: Thieme
Schwartz MS, Otto SR, Brackmann DE, Hitselberger WE, Shannon RV. (2003). Use of a multichannel auditory brainstem implant for neurofibromatosis type 2. Stereotact Funct Neurosurg 81:110–114
Shannon RV, Fayad J, Moore J, et al. (1993). Auditory brainstem implant: II. Post surgical issues and performance. Otolaryngol Head Neck Surg 108:634–642
Terr LI, Edgerton BJ. (1985). Three-dimensional reconstruction of the cochlear nuclear complex in humans. Arch Otolaryngol111:495–501
Terr LI, Fayad J, Hitselberger WE, Zakhary R. (1990). Cochlear nucleus anatomy related to central electro auditory prosthesis implantation. Otolaryngol Head Neck Surg 102:717–721
Toh EH, Luxford WM. (2002). Cochlear and brainstem implantation. Otolaryngol Clin North Am 35:325–342
Vincent C, Lejeune JP, Vaneecloo FM. (2000). The Digisonic auditory brainstem implant: report of the first three cases. In: Waltzman SB, Cohen NL, eds. Cochlear Implants, pp. 369–371. New York: Thieme
Vincent C, Zini C, Gandolfi A, et al. (2002). Results of the MXM Digisonic auditory brainstem implant clinical trials in Europe. Otol Neurotol 23:56–60
Waring MD. (1992). Electrically evoked auditory brainstem response monitoring of auditory brainstem implant integrity during facial nerve tumor surgery. Laryngoscope 102:1293–1295
Zimmerling M, Kovacs R, Janka M, et al. (2000). A new method to find the optimal location for an auditory brainstem electrode on the cochlear nucleus: preliminary results. In: Waltzman SB, Cohen NL, eds. Cochlear Implants, pp. 371–373. New York: Thieme
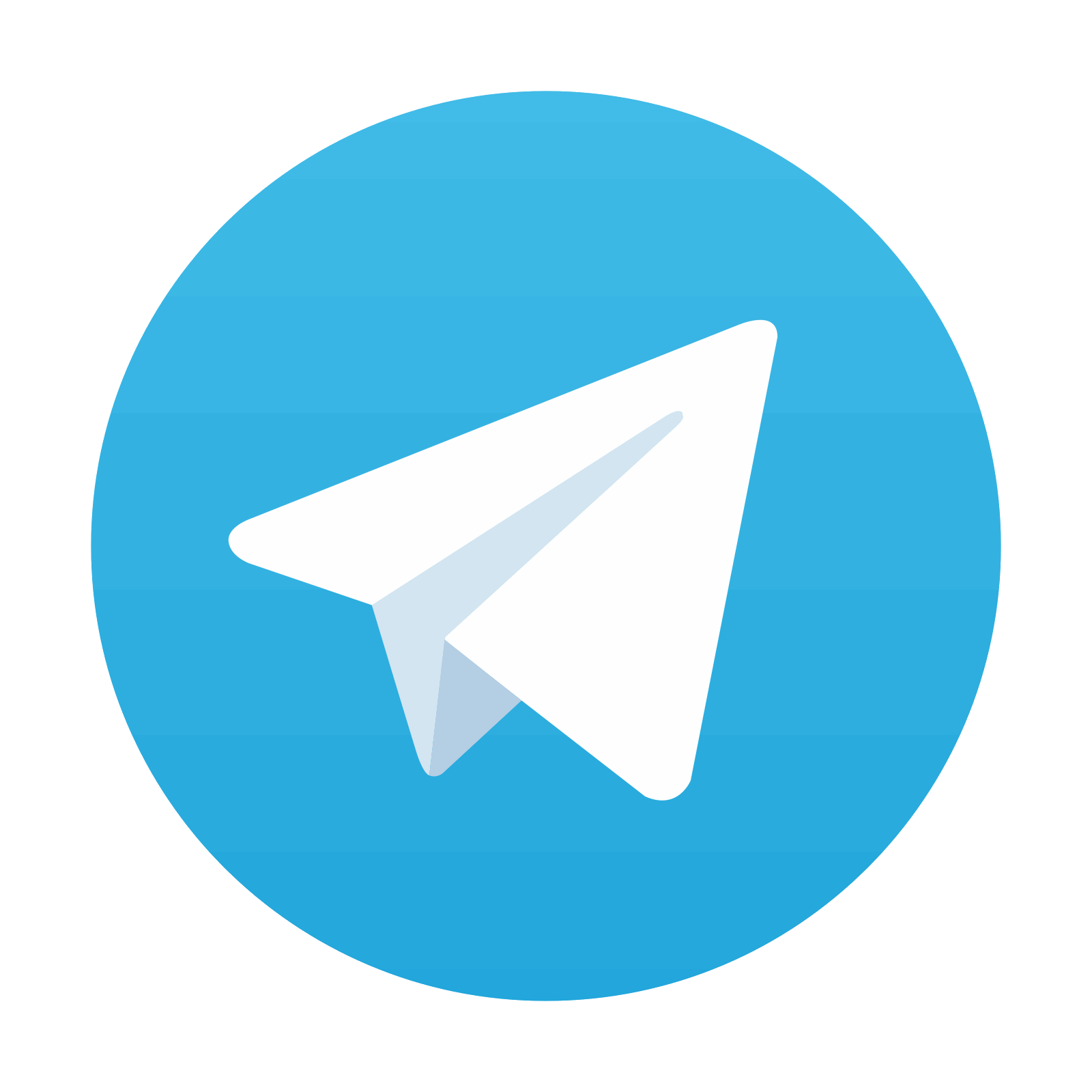
Stay updated, free articles. Join our Telegram channel

Full access? Get Clinical Tree
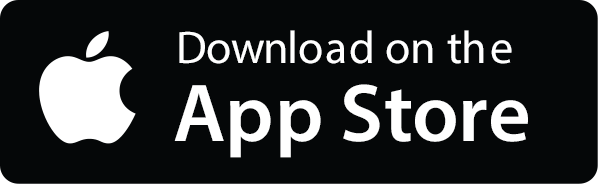
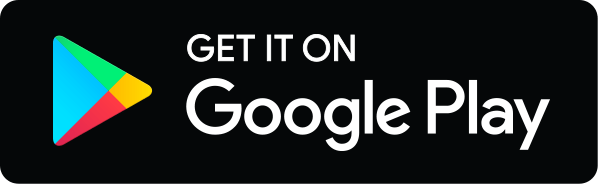