Assessment of Peripheral and Central Auditory Function
James W. Hall III
Patrick J. Antonelli
New techniques and strategies for assessment of the auditory function in adults have been introduced in recent years. Puretone audiometry, immittance measurement (tympanometry and acoustic reflexes), and calculation of word recognition scores continue to be important for hearing assessment, and the traditional audiogram is useful in summarizing the results of basic audiologic assessment. Clinical audiology, however, now also includes other behavioral and electrophysiologic test procedures. For example, electrocochleography (ECochG) can contribute to the diagnosis of Ménière disease. Auditory brainstem response (ABR) offers a readily accessible, and relatively inexpensive, means for estimating hearing sensitivity in infants and young children and for the identification of retrocochlear auditory dysfunction. Otoacoustic emissions (OAEs), because of unique sensitivity and specificity to cochlear dysfunction, have become an integral component of the clinical audiologic test protocol. And, within recent years, the auditory steady state response (ASSR) has emerged as a valuable addition to the pediatric audiologic test battery. A variety of speech and nonspeech behavioral measures and several cortical auditory evoked responses are available for clinical assessment of central auditory nervous system dysfunction and associated auditory processing disorders (APDs).
This chapter summarizes current techniques and strategies for hearing assessment among adults. The emphasis is on use of a test battery that maximizes diagnostic accuracy and efficiency and minimizes test time and cost. A glossary of common audiologic terms and abbreviations is provided at the end of the chapter.
BASIC AUDIOLOGIC TEST BATTERY
Pure-Tone Audiometry
Pure-tone audiometry is the most common measurement of hearing sensitivity. Stimuli are pure tones (sinusoids) at octave frequencies typically from 250 Hz up to 8,000 Hz and, often, two interoctave frequencies (3,000 Hz and 6,000 Hz). Interoctave hearing loss is a characteristic of commonly encountered problems, such as noise-induced cochlear dysfunction. High-frequency audiometry for stimulus frequencies greater than 8,000 Hz (up to 20,000 Hz) is technically feasible and clinically useful to certain populations, such as patients at risk for ototoxicity. Test results in many clinics are graphed on an audiogram. Two versions of audiograms are illustrated in Figure 142.1. All audiograms include at the minimum a graph for plotting hearing threshold levels as a function of the frequency of pure-tone signals, although the exact format and symbols vary.
The unit of stimulus intensity is the decibel (dB), a logarithmic unit. The intensity of any sound is defined by the ratio of its sound pressure or sound intensity to a reference sound pressure or sound intensity. The reference sound pressure is the amount of pressure against the eardrum, caused by air molecules when a sound is present, that vibrates the eardrum and can just be detected by a normal human ear. Briefly, the relation for sound intensity is described as dB = 10 log10 (sound intensity/reference intensity) or for sound pressure as dB = 20 log10 (sound pressure/reference pressure). The reference sound pressure is defined as decibels sound pressure level (dBSPL) and is derived from one of two physical quantities: (a) 0.0002 dyne/cm2 or (b) 20 micropascals root mean square (µPa) = 2 × 10-5 Pa.
Clinically, the intensity of sound is described in decibels hearing level (dB HL), a biologic reference level, rather than in sound pressure level. On an audiogram (Fig. 142.1), the decibel scale has as its reference 0 dB, which is described as audiometric 0. This is the standard for the intensity level that corresponds to the mean normal hearing threshold level, the minimal detectable intensity for each test frequency for young adults with normal hearing. Another common unit for expressing sound intensity is decibels
sensation level (dB SL), which is intensity of the stimulus in decibels above an individual’s hearing threshold. For example, a word recognition test can be administered at an intensity level of 40 dB SL (40 dB above the person’s puretone average [PTA]).
sensation level (dB SL), which is intensity of the stimulus in decibels above an individual’s hearing threshold. For example, a word recognition test can be administered at an intensity level of 40 dB SL (40 dB above the person’s puretone average [PTA]).
In audiologic assessment of cooperative children and adults, hearing thresholds for tonal or speech signals are measured separately for each ear with earphones (air-conduction stimulation). Insert earphones (ER-3A) are now the transducer of choice for routine audiologic assessment. They offer distinct advantages over traditional supraaural earphones, including increased comfort, reduced likelihood of ear canal collapse, greater interaural attenuation, and greater acceptance by young children. In addition, insert earphones contribute importantly to the control of infection in a clinical setting, as the insert portion is disposable. Pure-tone audiometry can be performed with stimuli presented with a bone-conduction oscillator or vibrator placed on the mastoid bone. During pure-tone audiometry, all equipment must meet the specifications of the American National Standards Institute (ANSI). Periodic equipment calibration and validation are necessary. Testing is conducted according to clinical adaptations of psychoacoustic methods (1). Patients are instructed to listen carefully for the tones and to respond, usually by pushing a button that activates a response light on the audiometer or by raising a hand, every time they believe they hear a tone. To minimize interference by ambient background acoustic noise, pure-tone audiometry always is performed with the patient in a double-walled, sound-treated room that meets ANSI specifications.
The clinically normal region on an audiogram is 0 to 20 dB HL, although for children hearing threshold levels exceeding 15 dB should be considered abnormal. Thresholds in the 20 to 40 dB HL region constitute mild hearing loss, 40 to 60 dB HL thresholds define moderate loss, and threshold levels greater than 60 dB HL are considered severe hearing loss (2). As a reference, the intensity level of whispered speech close to the ear is less than 25 dB HL. Conversational speech is in the 40 to 50 dB HL region, and a shouted voice within 1 foot (30 cm) of the ear is at a level of about 80 dB HL. The most important frequencies for understanding speech are 500 through 4,000 Hz, although higher frequencies can contribute to discrimination between certain speech sounds. Hearing sensitivity within the speech frequency region often is summarized by means of calculation of the PTA (PTA; hearing thresholds for 500, 1,000, and 2,000 Hz divided by three and reported in decibels). A four-frequency PTA including 3,000 Hz is required by the American Academy of Otolaryngology- Head and Neck Surgery.
Audiometric results are valid only when the patient’s responses are caused by stimulation of the test ear. If a sound greater than 40 dB HL is presented to one ear through air conduction with supraaural earphones and cushions (resting on the outer ear), the acoustic energy can cross over from one side of the head to the other and stimulate the ear not being tested. The main mechanism of crossover is presumed to be bone-conduction stimulation caused by vibration of the earphone cushion against the skull at high stimulus intensity levels. The amount of sound intensity needed before crossover occurs is a reflection of interaural attenuation, that is, the sound insulation between the two ears provided by the head. Interaural attenuation is usually about 50 dB for lower test frequencies and 60 dB for higher test frequencies, such as those contributing to the ABR. Interaural attenuation is considerably higher for insert earphones (2). With bone-conduction stimulation, interaural attenuation is less than 10 dB. In clinical circumstances, the examiner needs to assume conservatively that interaural attenuation for bone-conducted signals is 0 dB. In other words, even a very faint sound presented to the mastoid bone of one ear by a bone-conduction vibrator can be transmitted through the skull to either or both inner ears. Perception of this bone-conducted signal depends on the patient’s sensorineural hearing sensitivity in each ear.
Masking is the audiometric technique used to eliminate participation of the ear not being tested whenever air- and bone-conduction stimulation exceeds interaural attenuation. An appropriate noise (narrow-band noise for pure-tone signals and speech noise for speech signals) is presented to the ear not being tested when the stimulus is presented to the test ear. With adequate masking, any signal crossing over to the ear not being tested is masked by the noise. The level of masking noise presented to the ear not being tested must exceed the threshold of hearing for that ear. Excess levels of masking noise must be avoided because the noise can cross back over to the ear being tested. Selection of appropriate masking can be difficult, especially when there is bilateral hearing loss (2). Indeed, patients with severe bilateral conductive hearing loss may present the “masking dilemma,” that is, when enough masking to the nontest ear actually crosses over to the test ear and interferes with accurate estimation of hearing threshold. An otolaryngologist interpreting audiologic results must verify that appropriate masking was used if testing was not performed by an audiologist.
Knowledge of the type of hearing loss, determined by means of comparison of the hearing thresholds for air- and bone-conduction signals, is useful in classifying a hearing loss as sensorineural (no air-bone gap), conductive (normal bone conduction and a loss by air conduction), or mixed (loss by bone conduction with a superimposed air-bone conduction gap).
Configuration refers to hearing loss as a function of the test frequency. With a sloping configuration, hearing is better for low frequencies and then becomes poorer for higher frequencies. The most common pattern associated with sensorineural hearing loss is a deficit in thresholds for higher test frequencies. The configuration can be gently sloping from low to high frequencies, be precipitously decreasing above a high frequency cutoff, such as 2,000 Hz,
or be characterized by a notching deficit within a certain frequency region, such as 4,000 Hz. A rising configuration is typified by relatively poor hearing for low-frequency stimuli and better hearing for the high frequencies. A rising configuration can be caused by varied types of middle ear abnormalities. An exception to the typical association of conductive hearing loss with rising configuration is Ménière disease (see Chapter 156). Ménière disease is one cochlear abnormality that may produce a rising configuration. A flat audiometric configuration often is recorded from patients with mixed hearing loss, that is, both sensorineural and conductive components are present. Other configurations, such as the midfrequency “cookie bite” pattern, are encountered in clinical practice. Test-retest variability in clinical pure-tone threshold estimation is typically ±5 dB.
or be characterized by a notching deficit within a certain frequency region, such as 4,000 Hz. A rising configuration is typified by relatively poor hearing for low-frequency stimuli and better hearing for the high frequencies. A rising configuration can be caused by varied types of middle ear abnormalities. An exception to the typical association of conductive hearing loss with rising configuration is Ménière disease (see Chapter 156). Ménière disease is one cochlear abnormality that may produce a rising configuration. A flat audiometric configuration often is recorded from patients with mixed hearing loss, that is, both sensorineural and conductive components are present. Other configurations, such as the midfrequency “cookie bite” pattern, are encountered in clinical practice. Test-retest variability in clinical pure-tone threshold estimation is typically ±5 dB.
Guidelines for Evaluation of Hearing Handicap
The results of pure-tone audiometry are adequately summarized in an audiogram and with the terms just defined, such as PTA and the degree, configuration, and type of hearing loss. It also is possible to quantify hearing loss in percentage units according to published and accepted guidelines (3). This approach sometimes is necessary in medicolegal cases or when a patient seeks compensation for hearing loss. According to the guidelines of the American Academy of Otolaryngology Committee on Hearing and Equilibrium and the American Council of Otolaryngology Committee on the Medical Aspects of Noise (3), permanent hearing impairment is defined as follows: “A change for the worse in either structure or function, outside the range of normal, is permanent impairment…. Permanent impairment is due to any anatomic or functional abnormality that produces hearing loss.” This is differentiated from permanent hearing handicap, which is defined as follows: “The disadvantage imposed by an impairment sufficient to affect a person’s efficiency in the activities of daily living is a permanent handicap” (3). The guidelines also detail the approach for converting hearing handicap for one or both ears into a percentage. The first step is to determine the degree of sensorineural hearing loss for four test frequencies (500, 1,000, 2,000, and 3,000 Hz) from the audiogram (Table 142.1). The next step is to follow the guidelines for computation of percentage hearing loss (3):
If the monaural percent figure is the same for both ears, that figure expresses the percent hearing handicap. If the percent monaural hearing impairments are not the same, apply the formula:
(5 × % [better ear]) + 1 × % [ poorer ear]) / 6 = % hearing handicap
The interoctave test frequency—3,000 Hz—in the calculation of percentage of hearing handicap is very important. It is good clinical practice to routinely obtain hearing thresholds from each ear for the 3,000 Hz frequency. This frequency is included in the formula for hearing loss (3) because much of the spectral information vital for speech understanding is within the 2,000 to 3,000 Hz region. Percentage of binaural hearing handicap is easily calculated with a detailed tabular matrix that relates the four-frequency degree of sensorineural hearing loss for the better versus poorer ear (4).
TABLE 142.1 GUIDELINES FOR CALCULATING PERCENTAGE OF MONAURAL HEARING IMPAIRMENTa | ||||||||||||||||||||||||||||||||||||||||||||||||||||||||||||||||||||||||||||||||||||||||||||||||||||||||||||||||||||||||||||
---|---|---|---|---|---|---|---|---|---|---|---|---|---|---|---|---|---|---|---|---|---|---|---|---|---|---|---|---|---|---|---|---|---|---|---|---|---|---|---|---|---|---|---|---|---|---|---|---|---|---|---|---|---|---|---|---|---|---|---|---|---|---|---|---|---|---|---|---|---|---|---|---|---|---|---|---|---|---|---|---|---|---|---|---|---|---|---|---|---|---|---|---|---|---|---|---|---|---|---|---|---|---|---|---|---|---|---|---|---|---|---|---|---|---|---|---|---|---|---|---|---|---|---|---|
|
Speech Audiometry
The purpose of speech audiometry is to determine how well a person hears and understands speech signals. Speech audiometry procedures usually are performed to measure hearing sensitivity (thresholds in decibels) for words or to estimate word recognition, such as speech discrimination, ability. Spondee reception threshold, also called speech threshold, is the softest intensity level at which a patient can correctly repeat words approximately 50% of the time. Spondee words, two syllable words with equal stress on
each syllable, such as hot dog, baseball, and eardrum, are presented to the patient monaurally through earphones. The technique is comparable with the method for determining pure-tone thresholds described earlier.
each syllable, such as hot dog, baseball, and eardrum, are presented to the patient monaurally through earphones. The technique is comparable with the method for determining pure-tone thresholds described earlier.
Because PTA reflects hearing threshold levels in the speech frequency region and speech threshold is measured with a speech signal, one can expect close agreement between the PTA and the speech threshold. If the difference between PTA and speech threshold exceeds ±7 dB, there is reason to suspect that one or both of the measures are invalid. An unusually good speech threshold relative to PTA, such as speech threshold of 5 dB and PTA of 45 dB, immediately alerts the examiner to the possibility of nonorganic hearing loss, such as malingering. With cooperative adult patients, particularly if pure-tone hearing thresholds are within the normal region from 500 to 4,000 Hz, there is probably little or no clinical benefit in measuring speech thresholds. Test time can be saved with no loss of diagnostic information by excluding speech threshold measurement from the test battery for such patients.
Speech recognition for phonetically balanced words is a common clinical approach for estimating a person’s ability to hear and understand speech (2). A list of 25 or 50 single-syllable words typically are presented to the patient through earphones at one or more fixed intensity levels. The percentage of words correctly repeated by the patient is calculated by the examiner. One ear is tested at a time. Within the list of words, specific speech sounds (phonemes) occur approximately as often as they would in everyday conversation, that is, they are phonetically balanced. These words traditionally were spoken into a microphone by the examiner while the level was monitored with a volume unit meter. The words were routed to the patient through an audiometer after selection of the test ear and desired intensity level. This is an outdated and poor clinical practice because it lacks standardization and consistency and increases the variability of test outcome. For adult patients, it almost always is possible and always is preferable to use professionally produced, commercially available speech materials presented with a tape recorder or compact disk player and an audiometer (2). Live voice probably continues to be used by many practitioners, especially in the assessment of children, because of minor efficiency gains.
Speech perception in the presence of noise is also assessed clinically. One of the most popular and wellresearched procedures is the hearing in noise test, abbreviated HINT (5). Representative sentences of a standard length and phonetic complexity are presented with a low level of background noise, which is more reflective of speech understanding in real-world environments. HINT is a key measure of auditory performance used to screen hearing impaired individuals for cochlear implant candidates. The HINT is now available in over a dozen languages.
Acoustic (Aural) Immittance (Impedance) Measurement
Introduction
Aural immittance (impedance) measurement is an important part of the basic audiometric test battery. Immittance is a term derived from the terms for two related techniques for assessing middle ear function—impedance and admittance. These techniques have been used clinically since 1970 (6). The external ear canal is sealed with a soft rubber probe tip. The probe tip is connected to a device that produces a tone delivered toward the eardrum. Middle ear impedance or admittance is calculated from the intensity and other physical properties, such as phase, of the tone in the ear canal. A middle ear (tympanic membrane and ossicular system) with low impedance (higher admittance) more readily accepts the acoustic energy of the probe tone. A middle ear with abnormally high impedance (lower admittance) caused by, for example, fluid in the middle ear space tends to reject energy flow. Thus impedance (admittance) characteristics of the middle ear system can be inferred objectively with this technique and related to well-known patterns of findings for various pathologic conditions of the middle ear.
Tympanometry
Tympanometry is the dynamic recording of middle ear impedance as air pressure in the ear canal is systematically increased or decreased. The technique is a sensitive measure of the integrity of the tympanic membrane and of middle ear function (Fig. 142.2). Compliance (the reciprocal of stiffness) of the middle ear, the dominant component of immittance, is the vertical dimension of a tympanogram. Tympanometry is popular clinically because it requires little technical skill and only several seconds to perform. It is an electrophysiologic, as opposed to behavioral, method that does not depend on cooperation of the patient, and it is a highly sensitive index of middle ear function. Tympanometric patterns, in combination with audiographic patterns, allow differentiation and classification of middle ear disorders.
The most clinically widespread approach to describing tympanograms was described first by Jerger in 1970 (6). There are three general types of tympanogram—A, B, and C. A normal, or type A, tympanogram has a distinct peak in compliance within 0 to 100 mm water (dPa) in the ear canal (Fig. 142.2). To be classified as normal, the location of the compliance peak on the pressure dimension and the height of the peak must be within normal range, indicated in Figure 142.2 by the stippled area. On a type B tympanogram, there is no peak in compliance, but there is a flat pattern with little or even no apparent change in compliance as a function of pressure in the ear canal. This pattern is most often associated with the presence of fluid within the middle ear space (otitis media), although other middle ear disorders also can produce a type B tympanogram. Although a type B tympanogram can appear to be recorded from
ears with perforated tympanic membranes, technically, this finding is invalid because the change in ear canal pressure needed for tympanometry is not achieved owing to the perforation. Type C tympanograms have a distinct peak in compliance as do type A recordings, but the peak is within the negative pressure region beyond approximately 100 mm water (dPa). This pattern usually occurs among patients with eustachian-tube dysfunction and inadequate ventilation of the middle ear space. It often precedes acquisition of a type B tympanogram in the development of otitis media.
ears with perforated tympanic membranes, technically, this finding is invalid because the change in ear canal pressure needed for tympanometry is not achieved owing to the perforation. Type C tympanograms have a distinct peak in compliance as do type A recordings, but the peak is within the negative pressure region beyond approximately 100 mm water (dPa). This pattern usually occurs among patients with eustachian-tube dysfunction and inadequate ventilation of the middle ear space. It often precedes acquisition of a type B tympanogram in the development of otitis media.
A variation of a type A tympanogram is type As (Fig. 142.2).
The s stands for shallow. Peak compliance is less than the lower normal limit of compliance. That is, middle ear impedance is abnormally high. The type As pattern is common among patients with fixation of the ossicular chain, including some patients with the diagnosis of otosclerosis. In contrast, with an usually steep and high-compliance tympanogram (type Ad for deep), the peak can exceed the upper compliance limits of the equipment. A type Ad tympanogram occurs among patients with disruption of the ossicular chain, which leaves the middle ear extremely mobile and overly compliant, that is, there is little impedance. In the absence of serious hearing loss, this tympanographic pattern usually is associated with minor tympanic membrane abnormality, such as atrophy. At the beginning of a tympanometric examination, high positive or negative pressure is introduced into the ear canal. This essentially decouples the middle ear system from the measurement. If the impedance device records an abnormally large equivalent volume of air (2 cm or more in an adult, or twice the volume recorded for the other ear) between the probe tip and presumably the eardrum at this stage in the procedure, the integrity of the eardrum is questioned. That is, the immittance device is recording not only ear canal volume but also volume of the middle ear space. This test finding is consistent with perforation of the tympanic membrane or the presence of an open (patent) middle ear ventilation tube.
Acoustic Stapedial Reflex Measurement
The stapedial muscle within the middle ear is the smallest muscle in the body. Measurement of contractions of the stapedial muscle in response to high sound intensity levels (usually 80 dB or greater) is the basis of the acoustic reflex. Acoustic reflex measurement is clinically useful for estimating hearing sensitivity and for differentiating sites of auditory disorders, including the middle ear, inner ear, eighth cranial nerve, and auditory brainstem (2, 6). The afferent portion of the acoustic reflex arc is the eighth cranial nerve. Complex brainstem pathways lead from the cochlear nucleus on the stimulated side to the region of the motor nucleus of the seventh cranial (facial) nerve on both sides (ipsilateral and contralateral to the stimulus) of the brainstem. The efferent portion of the arc is the seventh cranial
nerve, which innervates the stapedius muscle. The muscle contracts, causing increased stiffness (decreased compliance) of the middle ear system. The small change in compliance that follows stapedius muscle contraction within 10 ms is detected with the probe and immittance device, much as compliance changes are detected during tympanometry.
nerve, which innervates the stapedius muscle. The muscle contracts, causing increased stiffness (decreased compliance) of the middle ear system. The small change in compliance that follows stapedius muscle contraction within 10 ms is detected with the probe and immittance device, much as compliance changes are detected during tympanometry.
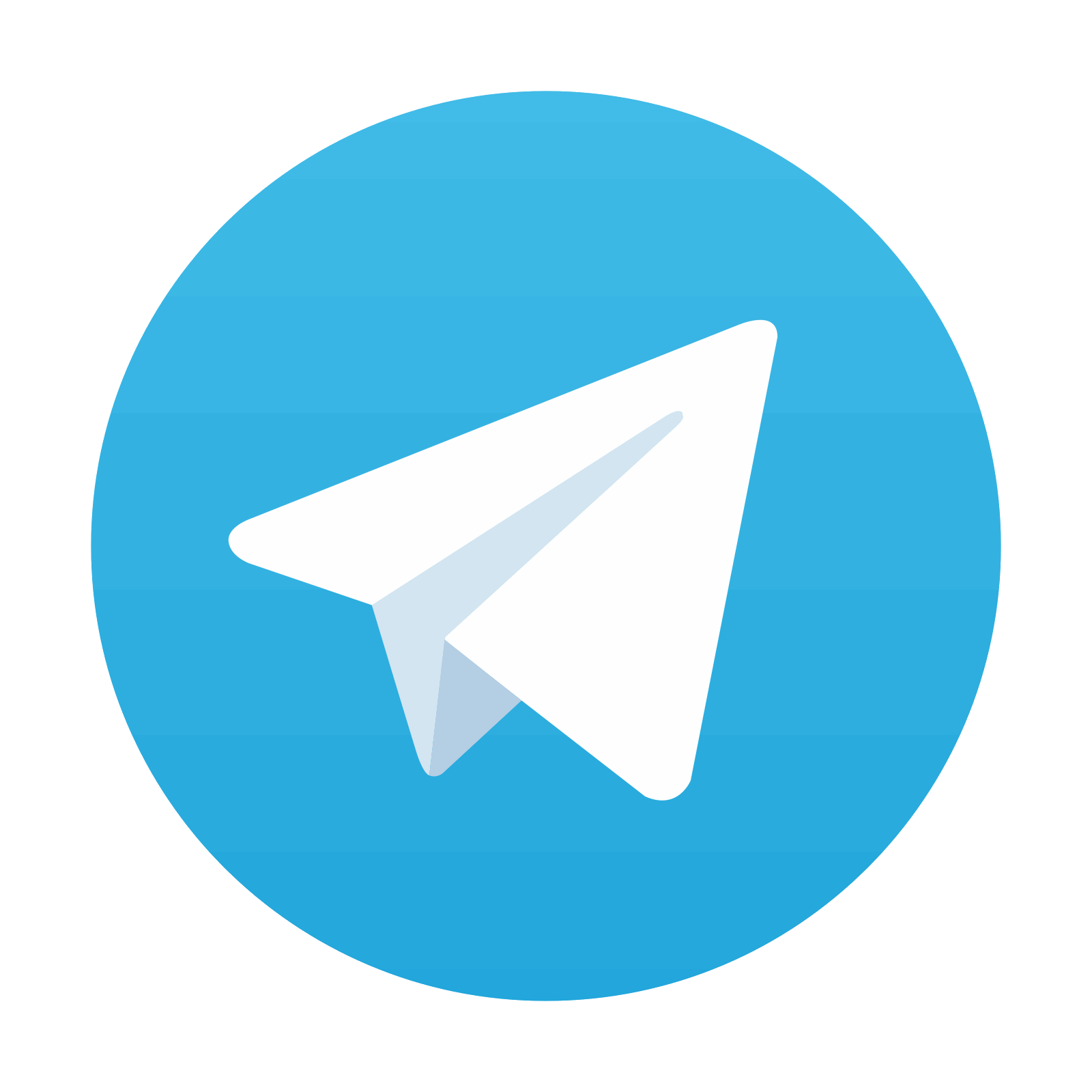
Stay updated, free articles. Join our Telegram channel

Full access? Get Clinical Tree
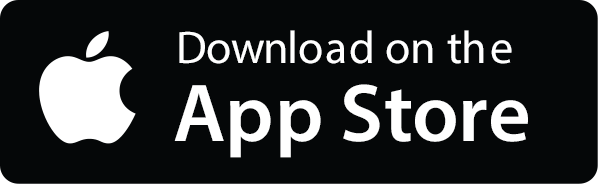
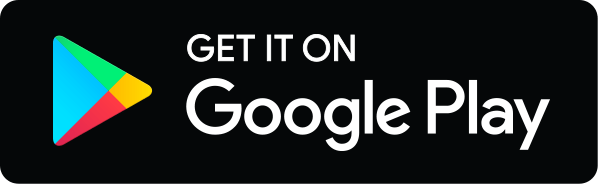